the Creative Commons Attribution 4.0 License.
the Creative Commons Attribution 4.0 License.
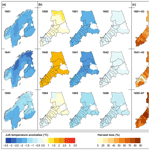
Recession or resilience? Long-range socioeconomic consequences of the 17th century volcanic eruptions in northern Fennoscandia
Markus Stoffel
Christophe Corona
Past volcanic eruptions and their climatic impacts have been linked increasingly with co-occurring societal crises – like crop failures and famines – in recent research. Yet, as many of the volcanic cooling studies have a supra-regional or hemispheric focus, establishing pathways from climatic effects of an eruption to human repercussions has remained very challenging due to high spatial variability of socio-environmental systems. This, in turn, may render a distinction of coincidence from causation difficult. In this study, we employ micro-regionally resolved natural and written sources to study three 17th century volcanic eruptions (i.e., 1600 Huaynaputina, 1640/1641 Koma-ga-take–Parker, and 1695 unidentified) to look into their climatic and socioeconomic impacts among rural agricultural society in Ostrobothnia (Finland) with high temporal and spatial precision. Tree-ring and grain tithe data indicate that all three eruptions would have caused significant summer season temperature cooling and poor grain harvest in the region. Yet, tax debt records reveal that the socioeconomic consequences varied considerably among the eruptions as well as in time, space, and within the society. Whether the volcanic events had a strong or weak socioeconomic effect depended on various factors, such as the prevailing agro-ecosystem, resource availability, material capital, physical and immaterial networks, and institutional practices. These factors influenced societal vulnerability and resilience to cold pulses and associated harvest failures. This paper proposes that, besides detecting coinciding human calamities, more careful investigation at the micro-regional scale has a clear added value as it can provide deeper understanding of why and among whom the distal volcanic eruptions resulted in different societal impacts. Such understanding, in turn, can contribute to interdisciplinary research, advise political decision-making, and enhance scientific outreach.
- Article
(5358 KB) -
Supplement
(860 KB) - BibTeX
- EndNote
Common understanding exists that volcanic aerosol forcing has been a considerable driver of pre-industrial summer temperature variability at interannual-to-decadal timescales (Robock and Mao, 1995; Sigl et al., 2015; Stoffel et al., 2015; Büntgen et al., 2020), and the climate system reactions to volcanic aerosol forcing have been studied extensively over recent decades (see, e.g., Robock, 2000; Cole-Dai, 2010; Timmreck, 2012). By contrast, the various distal societal consequences of volcanic eruptions have hitherto received much less attention (Oppenheimer, 2015). This generalized lack of research dedicated to societal impacts of volcanic cooling is likely related to the limited availability of published societal data, especially for the more distant past. In fact, in many states, official statistics only reach back to the 19th or 20th century and therefore cover a time period for which the number of large eruptions is rather limited. Based on estimated global aerosol forcing, 23 of the 25 largest aerosol forcing eruptions of the last 2500 years occurred before 1800 (Sigl et al., 2015; Toohey and Sigl, 2017), i.e., during a time for which published societal data are generally rather scarce. For this “pre-statistical era”, various historical sources can provide alternative information on the well-being of contemporaries. Some recent interdisciplinary studies have employed these archival sources to focus on far-flung societal impacts of particular eruptions of the last millennium such as the 1108/1110 unidentified (Guillet et al., 2020), the 1257 Samalas (Stothers, 2000; Campbell, 2017; Guillet et al., 2017), the 1600 Huaynaputina (Fei et al., 2016; White et al., 2022), the 1640s cluster (Stoffel et al., 2022), the 1690s unidentified (D'Arrigo et al., 2020), the 1783 Laki (Demarée and Ogilvie, 2001; Witham and Oppenheimer, 2004; Grattan et al., 2007), and especially also the 1815 Tambora (Oppenheimer, 2003; Auchmann et al., 2012; Krämer, 2015; Luterbacher and Pfister, 2015; Brönnimann and Krämer, 2016) eruptions. All these studies highlighted that volcanic eruptions can indeed have considerable, yet diverse, human consequences far away from the eruption location.
Although these studies have successfully identified linkages between eruptions, climatic responses, and various human repercussions, any quantification and attribution of societal impact resulting from volcanic events have remained very challenging. This is because human societies are extremely complex systems, where existing institutions, the surrounding environment, and actions of individuals, among other factors, influence the materialization of natural hazards – like volcanic eruptions – on a societal level (Haldon, 2016; van Bavel et al., 2020; Ljungqvist et al., 2021; Degroot et al., 2021). Therefore, any distinction of how much eruption-related climatic anomalies on the one hand, and existing socio-environmental conditions and emerging human responses on the other, explain the detected societal events remains difficult. To isolate possible volcanic effects from other natural and human-made factors, systematic longitudinal studies are recommended, that is, studies that span multiple volcanic eruptions and compare societal impacts associated with different eruptions over time. However, as societies can evolve rather quickly, for example due to advances in technology and science, limiting the focus on one historical epoch might be desirable. Consequently, we will focus on the so-called Early Modern Period (spanning roughly from end of the Middle Ages to the era of modernization), which overlapped with the climatic regime of the Little Ice Age.
Longitudinal studies require detailed, continuous, and relatively homogeneous documentation on various aspects of human life, which should overlap with climate data with similar temporal and spatial resolution. This limits the number of potential subjects of study considerably. The history of collecting and storing information systemically is commonly related to efforts aiming to gain social and economic control and to legitimize the power of authorities (Hallenberg et al., 2008). In this respect, the taxation accounts of the 17th century Swedish realm (consisting of roughly present-day Sweden, Finland, and Estonia) provide an exceptionally detailed proxy data archive on the livelihood and socioeconomic status of contemporaries. To utilize the limited resources of this sparsely populated northern kingdom (Fig. 1), the Crown established an extensive, centralized administration to collect taxes from the peasantry (Kujala, 2003; Hallenberg et al., 2008). The officers of the realm kept detailed records on the quantity and quality of cultivated lands, of the taxes and other tributes collected, and of possible delays and reductions of these payments.
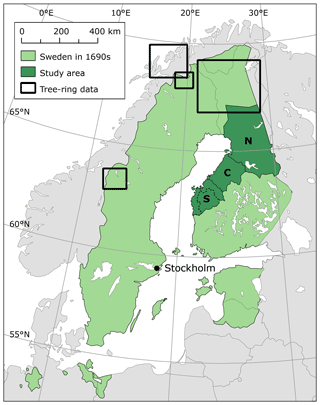
Figure 1Study area, the approximate tree-ring data sample sites for the temperature reconstruction used in this study, and the sub-regions (northern, central, and three southern regions; see Table 1) referenced in the text.
Besides rich documentary material, the 17th century also provides good temporal scope for a longitudinal study, as it witnessed three eruptions with comparable volcanic stratospheric sulfur injection (VSSI) from the 1600 Huaynaputina (Peru), the 1640/1641 Koma-ga-take–Parker (Japan–Philippines), and the 1695 unidentified tropical (Briffa et al., 1998; Sigl et al., 2015; Toohey and Sigl, 2017; Stoffel et al., 2022; White et al., 2022) eruptions. In fact, reconstructions based on sulfate records from Greenland and Antarctic ice cores indicate that the three eruption events are comparable not only in terms of the estimated VSSI, but also regarding global mean aerosol optical depth and global radiative forcing (Toohey and Sigl, 2017).
By integrating tree-ring and written source materials, we investigate here the micro-regional temperature response and related livelihood and socioeconomic impacts of these three 17th century volcanic events in the historical province of Ostrobothnia (present-day Finland). The objective is not limited to detecting and quantifying the societal consequences in space and time. Special emphasis is also laid on exploring the various socio-environmental factors that influenced the degree of impact. In doing so, we hope to demonstrate the potential of detailed historical research contributing to the interdisciplinary community exploring the climatic and societal impacts of past volcanic eruptions.
The 17th century CE has been described as the era of “global crisis”, when the world was burdened by wars, rebellions, famines, and the outbreak of disease. Geoffrey Parker (2013) connected these events to deteriorating climatic conditions, resulting from increased volcanic activity, frequent El Niño events, and decreased solar activity. Yet the climatic responses to volcanic forcing are known to vary considerably in spatial and seasonal terms globally (see, e.g., Fischer et al., 2007; D'Arrigo et al., 2013; Zanchettin et al., 2013a, b; Swingedouw et al., 2017). Furthermore, broad narratives, like the 17th century global crisis, commonly fail to provide causal reasoning on the relationships between distant volcanic eruptions, regional climate variability, and local societal consequences (Warde, 2015; Degroot et al., 2021). Previous research on the human consequences of past volcanic events was focused largely on supra-regional crises, like famines and societal collapse. By contrast, possible distal impacts on a local grassroots level have received much less attention. By studying the climatic and societal impacts of the 1690s crisis in Scotland, D'Arrigo et al. (2020) demonstrated that instead of performing large-scale analyses, a local or micro-regional spatial focus can provide deeper understanding of the spatio-temporal and socioeconomic disparity of the human repercussions.
Consequently, the spatial scope of this study is restricted to the historical province of Ostrobothnia, located in present-day Finland (Fig. 1). The northernmost parts (above 68.3∘ N) of the province are excluded from the analysis, however, as agriculture was non-existent or marginal in the 17th century, and hence the farmstead-based written documentation is limited. The province was extremely sparsely populated in the 17th century: whereas the study area covers 111 583 km2, only ca. 91 150 people inhabited the area by 1695 (Muroma, 1991), of which a substantial majority were rural peasants. Their livelihood was based on arable crop cultivation in the southern and central parts of the province, but in the north-easternmost part of the province, agriculture consisted primarily of slash-and-burn cultivation, and in the northernmost study area people gained their livelihood mainly from a mixture of animal husbandry and subsidiary crop cultivation (Soininen, 1974). The practiced agriculture is considered to be “traditional”, and almost “stagnated”, over the century (Soininen, 1974). The onset of the thermal conditions and during the growing season were the main yield-limiting factors in this northern province, such that solely April–August mean air temperatures can explain approximately one-third of the annual pre-industrial crop yield variations (Huhtamaa et al., 2015).
Unlike in many other parts of Europe, a majority of the 17th century Ostrobothnian peasants were freeholders. However, as 17th century Sweden was a strictly centralized state, all land still belonged technically to the Crown, except for the nobility's properties. Thus, the freeholders had only the hereditary and usufruct rights of their holding. If a peasant failed to pay taxes on time, the Crown took full ownership of the farmstead, and the peasant lost its hereditary and usufruct rights (Soininen, 1974). In such a case, properties became administratively Crown holdings (kronohemman) and their inhabitants Crown peasants. Additionally, new settlements, (i.e., farmsteads which cleared agricultural land from forest areas) were increasingly categorized as Crown holdings over the century. Consequently, the number of Crown holdings increased over the 17th century, and by the 1690s, the ratio between freeholder and Crown peasant holdings was approximately three to one. In addition to the freeholder and Crown holdings, the study area also included varying numbers of so-called manorial peasant farmsteads (Kujala, 2003). These were peasant holdings which were located on the lands of nobility. Yet this group is excluded from this study, as manorial peasants paid their taxes and rents to the nobility's estates and thus are largely absent in the administrative records of the Crown.
3.1 Temperature data
Tree-ring width (TRW), and even more so maximum latewood density (MXD) chronologies, have become the backbone of summer temperature reconstructions and the assessment of the climatic impact of past volcanic eruptions (D'Arrigo et al., 2013; Stoffel et al., 2015). To put the cooling induced by the three 17th century volcanic eruptions into perspective, we reconstructed summer (i.e., June–August, or JJA) temperature anomalies for Fennoscandia and Ostrobothnia using multi-centennial Scots pine (Pinus sylvestris L.) chronologies (MXD and TRW) from four sites located in northern Fennoscandia (Fig. 1) (Schweingruber et al., 1988; Kirchhefer, 2001; Esper et al., 2012; Melvin et al., 2013; Schneider et al., 2015). Each chronology was standardized using the regional curve standardization (RCS) method as it optimally preserves multi-decadal temperature variations in the reconstruction (Helama et al., 2017). We then transferred this record into JJA temperatures through a bootstrap linear model using a principal component analysis (PCA) calibrated against JJA land surface temperatures (1920–2000) from the E-OBS 10 min × 10 min gridded dataset (Cornes et al., 2018). For each grid point, the calibration and validation process was repeated 1000 times using a bootstrap approach. To test the robustness of the reconstruction, we employed the coefficient of determination (R2 for the calibration and r2 for the verification periods), RE (reduction of error), and CE (coefficient of efficiency) statistics (Supplement, Fig. S1).
3.2 Grain harvest fluctuations
As the main livelihood of the majority of the study area's population came from agriculture, and specifically from crop cultivation, the possible impacts of volcanic eruptions on livelihoods are studied here with grain harvest proxy data. Grain tithe tax records are commonly used to detect annual harvest fluctuations in historical Europe (Le Roy Ladurie and Goy, 1982), and tithes have been shown to reflect relative harvest fluctuations rather well, particularly in early modern Sweden (Leijonhufvud, 2001). In 17th century Ostrobothnia, each peasant holding was supposed to pay a share (ca. 10 %) of their yearly harvest as a tithe tax to the authorities (Huhtamaa et al., 2020). The tithes consisted of roughly equal shares of rye and barley, but in the northern region, tithes were paid almost entirely in barley. Previously published (Huhtamaa and Helama, 2017) tithe series from southern Ostrobothnia were extended to cover the whole study area. The tithe time series were collected on a parish level and then transformed to indicate annual variations with respect to a pre-crisis (or pre-peak forcing) 10-year mean.
The grain tithe time series were analyzed with a superposed epoch analysis (SEA) to investigate the possible volcanic signal in the data (Robock and Mao, 1995). As the volcanic events included at least one unidentified eruption (1695), the time series were superposed on the year of peak global volcanic aerosol forcing rather than on the year of the eruption. The dating of peak forcing year is based on Toohey and Sigl (2017). In addition, spatial variations and relationships between 3-year mean harvest losses were explored at the parish level.
3.3 Proxy data on socioeconomic change: desertion ratio
The main economic burden for the 17th century peasant was paying taxes to the Crown. Thus, the possible socioeconomic repercussions are assessed here with tax debt records. These records are particularly appropriate to address the societal and economic consequences on a grassroots level, as the majority of the people living in the studied area were peasants, their family members, or farmhands living with the peasant family. Thus the tax-paying ability of a holding reflects the overall subsistence status of the extended family household.
If a farmstead within the 17th century Swedish kingdom failed to pay taxes over 3 consecutive years, it was marked as öde, which means “deserted”. Hence, the holding was not always deserted in a literal sense if it was marked as such (Fig. 2). Nevertheless, we will use here the literal English translation of öde to avoid possible anachronistic expressions, like insolvency. In addition, the category of deserted holdings included uninhabited farmsteads as well, because a holding was also marked as deserted if the peasant fled, migrated, or perished (Mäntylä, 1988). Thus, these desertion markings overall serve as a proxy for subsistence decline, and in many cases for severe societal hardships. Furthermore, desertion variations can imply changes in land tenure and social status. If a freeholder farmstead was marked as deserted, the peasant lost its hereditary right for the property, and it was released for the Crown to claim. In the best case, the peasant could continue cultivating the farm as a tenant of the Crown – i.e., as a Crown peasant – without the hereditary and usufruct rights for the holding. In the worst case, the family was evicted from the farm. The situation was even more perilous when a Crown peasant had tax-paying difficulties, as the authorities did not recognize their usufruct rights. Consequently, these situations commonly ended with eviction (Kujala, 2003). In a rural society like that of 17th century Ostrobothnia, such evictions inevitably affected the economic possibilities and social status of a person.
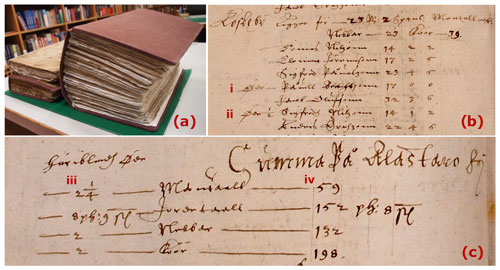
Figure 2(a) Early 17th century annual land, account, and verification books (from bottom left to right). (b) Examples of two deserted farmsteads in the 1603 land book, which also documented the numbers of adults living in the holding (second column from the right). The first holding (i) was uninhabited, whereas the second holding (ii) still had one adult living on the farmstead. (c) Example of the registers from which the desertion data for this study are gathered: the chapel of Alastaro (Vähäkyrö) held 59 mantal farmsteads (iv), of which 2.25 mantal units were deserted (iii).
The desertion material was retrieved from various accounts related to taxation, which are bound as verification, account, and land books (Fig. 2a). These records are available in so-called bailiff (up until 1634) and provincial (from 1635 onwards) accounts, stored at the National Archives of Finland. The number of total and deserted holdings are given in mantal units in the documents (Fig. 2c). Mantal was a Swedish farm taxation unit, which was assigned according to the quantity and quality of the arable land of a peasant holding (Olsson and Svensson, 2010). The desertion data were collected for the 7 years preceding and the 7 years following peak volcanic aerosol forcing. When available, the share of freeholder and Crown peasant holdings (both the total number of both holding types and the share of deserted holdings) was also gathered. The collected time series indicate the average desertion ratio, that is the share of deserted holdings in relation to all holdings (based on the number of holdings existing in the year of peak volcanic aerosol forcing).
The desertion ratio data were examined with the SEA procedure similar to the grain tithe data. However, whereas the grain tithes were recorded on a parish level throughout the 17th century, the number of deserted holdings were documented on spatially wider administrative units in the years 1594–1599 and in 1602 in the sources. These wider areas are not comparable with each other regarding the number of farmsteads (varying from 122 to 805 holdings). Thus, to avoid a possible bias towards more sparsely populated areas in the analysis, the desertion ratio SEA was performed for five comparable sub-regions (Fig. 1). Additionally, the 3-year mean desertion ratios were explored at the parish level.
The summer temperature, grain tithe, and desertion data show clear post-volcanic signals when aligned with the years during which volcanic stratospheric sulfur injection reached its highest values (Figs. 3, 4). Summer temperatures were reconstructed temporally over Ostrobothnia and spatially over Fennoscandia. The reconstructions are robust with a CE >0.2 over Scandinavia and Finland; they explain more than 70 % of June–August (JJA) temperature variability approximately over the Swedish province of Lapland and Norwegian county of Nordland and 50 % over Ostrobothnia (Fig. S1).
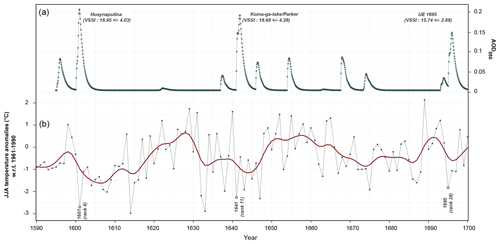
Figure 3Three major eruptions are recorded in ice-core records for the 17th century in 1600 (Huaynaputina), 1640–41 (Koma-ga-take and Mount Parker), and 1695 (unidentified eruption): (a) stratospheric aerosol optical depth (SAOD) estimated for the latitude of Ostrobothnia using the Volv2k_v2 EVA AOD 500–1900 (Toohey and Sigl, 2017) dataset; (b) summer (JJA) temperature anomalies (with respect to 1961–1990) reconstructed for the period 1590–1700 over Ostrobothnia. Note that cold years followed the three major volcanic events but were also reconstructed for years without volcanic activity (e.g., 1614, 1633).
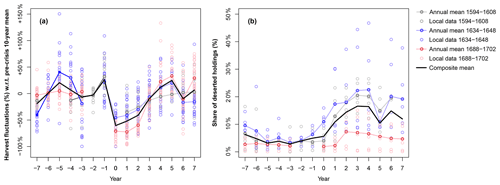
Figure 4The 15-year segments of composite (a) grain tithe and (b) desertion data indicating the grain harvest and socioeconomic responses, respectively, centered on the 17th century years of peak global volcanic aerosol forcing (1601, 1641, 1695).
The mean JJA temperature reconstruction containing all grid points (10 min × 10 min) of Ostrobothnia is shown in Fig. 3. For the years following the three major 17th century eruptions, the reconstruction points to substantial cooling with −2.70 ∘C in 1601 (with respect to the reference period 1961–1990; rank 4 in terms of cooling for the period 1500–2000), −2.27 ∘C in 1641 (rank 11), and −1.82 ∘C in 1695 (rank 38). The cooling in 1601 and 1641 falls within the 5th percentile (−1.98 ∘C) of the coldest JJA temperatures reconstructed since 1500 CE. We also note, however, that very cold summers existed in the 17th century in the absence of volcanic forcing. By way of example, 1614 and 1633 were particularly cold with −2.98 ∘C (rank 2) and −2.89 ∘C (rank 3), respectively. Additionally, we do not observe substantial cooling in the study region after the 1815 Tambora eruption (−0.74 ∘C in 1816, rank 158) (Fig. S2). The unprecedented resolution of the E-OBS dataset (10 min × 10 min) also allowed comparison of the cooling reconstructed for Ostrobothnia with temperatures across the study region as well as elsewhere in Fennoscandia (Fig. 5a, b). In order to quantify the reconstructed cooling within a context of climate variability prevailing at the time of the three volcanic eruptions, reconstructed anomalies are expressed with respect to a 31-year running mean. For example, in the case of the year 1641 CE, a background was calculated by averaging the window 1626–1640 CE and 1642–1656 CE. The anomaly is then created by subtracting this background from the 1641 CE reconstructed temperature. In 1601, cooling is rather homogeneous across Fennoscandia – and hence also Ostrobothnia, whereas for the other eruptions, clear differences exist in the magnitude of cooling across the area. In 1641, the strongest cooling is observed north of the Arctic circle, whereas in 1695, cooling was most pronounced in southern Scandinavia (below 60∘ N).
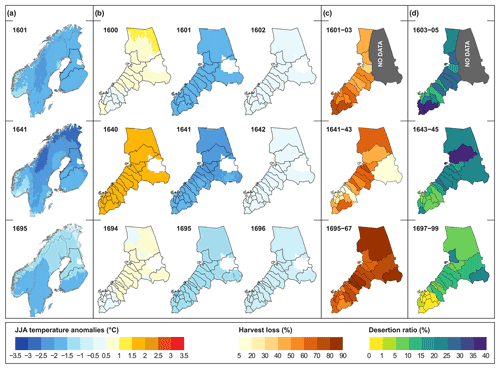
Figure 5Spatial JJA temperature anomalies reconstructed over (a) Fennoscandia and (b) Ostrobothnia using the E-OBS 10 min ×10 min gridded dataset (with respect to 31-year running mean), (c) 3-year harvest loss mean (with respect to 10-year pre-crisis mean), and (d) 3-year desertion ratio mean for the cooling induced by the Huaynaputina eruption in 1601 (upper row), the Koma-ga-take–Mount Parker eruptions in 1641 (middle row), and unidentified 1695 eruption (lower row).
When compared to the pre-crisis (or pre-forcing) 10-year mean, the tithe data suggest grain yields to be less than half of the average during the year of peak volcanic forcing (Fig. 4). Whereas the harvest losses were the greatest in the year of peak forcing, harvest quantities remained low over the 2 following years as well. In 1601, grain harvest was so badly destroyed that the tithes were not collected at all. However, this year was excluded from the SEA composite as it seems unlikely that not a single grain of rye or barley was harvested – even if contemporaries described that this was indeed the case (Ulkuniemi and Thomasson, 1975). Furthermore, the tithe data were not found for the nine northernmost provinces for the year 1641. Thus, as the year of peak forcing evidenced the greatest harvest losses, likely the total harvest lost over the period 1641–1643 in these nine northern parishes was greater than illustrated in Fig. 5c.
The ratio of deserted holdings increased considerably in the years after the first harvest losses (Fig. 4b), peaking on average in the third year (±1 year) following peak volcanic forcing and the first harvest failure. This is in accordance with the practice of registering a holding as deserted if taxes remained unpaid for 3 years in a row. Yet the 15-year segments of the desertion ratio indicated more pronounced spatio-temporal response variations than the grain tithe series. The highest desertion ratios are observed after the Huaynaputina and Koma-ga-take–Parker eruptions, when 20 % of the holdings, on average, were deserted from 2 to 4 years after peak forcing. The mean desertion ratio was considerably lower (7 %) in the aftermath of the 1695 unidentified volcanic event.
As the major impact on the harvest took place in the year of peak volcanic forcing and the 2 subsequent years, and the main increase in desertion ratios in years +2 to +4 after peak forcing (Fig. 4), we plotted mean harvest loss and desertion ratios at the parish level covering these 3-year periods, respectively, in order to gain further understanding of the spatio-temporal variability of the impacts (Fig. 5c, d). Interestingly, no specific locality stood out. Instead, the regions hit worst in terms of harvest losses and desertion varied among the eruptions. Furthermore, the relation between harvest loss and desertion ratio varied over the different crisis periods as well (Fig. 6). Following the 1600 eruption, both harvest losses and desertion ratios were relatively high in each parish. The tithe data indicate that harvest losses were not as severe after the 1640/41 eruptions, yet many parishes still ended up marking over 20 % of the holdings as deserted. The most severe harvest losses followed the 1695 event, when mean harvest in 1695–97 was more than 56 % lower than the pre-crisis mean in each parish. However, the following desertion was moderate, as 17 out of the 26 parishes had less than 10 % of the holdings deserted (Fig. 6).
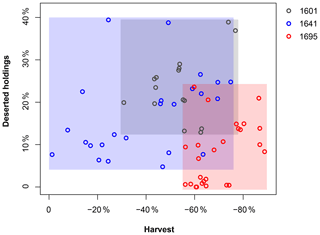
Figure 6Relationship between 3-year harvest loss and desertion ratio means (data same as in Fig. 5) in Ostrobothnian parishes following the three volcanic events.
The socioeconomic impacts also varied within the peasant society. In the northern half of the study area, the accounts do not reveal whether a deserted holding was inhabited by a freeholder or a Crown peasant until the late 17th century, whereas these details are listed throughout the century in the account books of the three southern regions (Fig. 1). As almost all holdings were freeholder farms at the beginning of the century, all the deserted holdings were such farms as well. Due to the tax-paying difficulties resulting from the hardships of the early 1600s and repeated harvest failures in 1632–1635 (Johanson, 1924), when reconstructed air temperatures were very cold in the study region – especially in 1633 and in the absence of volcanic forcing – peasants started to lose their hereditary and usufruct rights, and the number of Crown holdings started to rise slowly. By the mid-1640s, approximately 93 % of the holdings were freeholder farms, and the remaining 7 % were Crown holdings. Despite Crown peasants constituting less than of the rural peasant population, their share of desertion markings in the account books is striking: from all deserted peasant farms, on average 48 % were Crown peasant holdings in 1643–45. In fact, in 1645 as many as 146 out of the 171 Crown holdings in the region had this marking, indicating severe deprivation. The situation is similar during the 1690s crisis: by this time Crown peasants constituted approximately one-quarter of the peasant population, but their share of occupying the deserted holdings was between ca. 69 % and 99 % over the years 1697 and 1699 (Table 1). Thus, the socioeconomic consequences were not equal among the rural society, but were substantially pronounced among the Crown peasants.
Table 1Number of all holdings, the share of Crown peasant holdings in relation to all holdings, the number of all deserted holdings, and the share of deserted Crown peasant holdings in relation to all deserted holdings (all 3-year means) in the three southern parts of the study area (see Fig. 1, dashed lines). Note that data from the central and northern parts, which constituted ca. 80 % of the land area and hold ca. 45 % of the farmsteads of the province, are not included in the table.
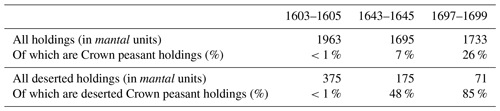
5.1 The 17th century eruptions
The 17th century saw a series of major volcanic eruptions – according to the volcanic explosivity index (VEI) and using a threshold of VEI ≥5, between two and seven eruptions comparable to 1601, 1641, and 1695 would have occurred in the 17th century (see, e.g., Briffa et al., 1998; Esper et al., 2013; Venzke et al., 2013). By contrast, Parker (2013) even listed as many as nine VEI ≥5 eruptions that would have contributed to the climatic regime of the 17th century global crisis besides the 1600, 1640–41, and 1695 events. In the most recent database, Toohey and Sigl (2017) list 12 eruptions for the 17th century, but they also state that nine of these had a limited climatic impact as their estimated volcanic stratospheric sulfur injection (VSSI) was <5 Tg[S]. Only the three eruptions in 1600, 1640/41, and 1695 released more than 15 Tg[S], and thus likely triggered substantial cooling over the study area. In addition to the absence of a linear relation between the amount of sulfur injected to the stratosphere and the resulting temperature cooling, one should also consider the prevailing background climatic conditions as well as eruption location and season (Robock, 2000; Zanchettin et al., 2013a), as they will critically determine the climatic effects of a given eruption. Many past studies solely used the VEI to link climatic or societal deterioration to volcanic forcing, even if it is well established that this index does not directly indicate the climate impact potential of a volcanic eruption (Robock and Free, 1995; Gao et al., 2008). We can thus only insist that any direct attribution of societal repercussions to volcanically forced cooling using evidence of VEI estimates alone should be avoided.
Whereas the cooling induced by some of the other 17th century eruptions cannot therefore be attributed easily to volcanism alone, we evidence that the VSSIs and their impact on global radiative forcing (Sigl et al., 2015; Toohey and Sigl, 2017) likely contributed considerably to the cold summers observed over Fennoscandia in 1601, 1641, and 1695. The strong JJA cooling shown in this study (Fig. 3), especially in 1601 and 1641, is in agreement with observations (Jones et al., 2003) and model simulations (Schneider et al., 2009), indicating pronounced post-volcanic summer cooling over most of Europe. Considering the unidentified 1695 volcanic eruption, the very cold JJA temperatures reconstructed in 1695 can be associated with volcanic forcing if the eruption has occurred in summer or autumn 1694. Should the unidentified eruption instead only have taken place in winter 1694–1695 or thereafter, one could not exclude that the cold conditions in summer 1695 resulted simply from internal climate variability, which produced several cold extremes in the absence of volcanic aerosol forcing during the Maunder Minimum.
The grain harvest and socioeconomic responses lasted considerably longer than the volcanic-induced cold pulses (Fig. 4). The prolonged impact on grain harvest is likely partly explained by the poor quality and quantity of seed grain and partly because of the continuing unfavorable (non-volcanic) weather conditions, or the combination of both (Voipio, 1914; Muroma, 1991; Huhtamaa and Helama, 2017; Huhtamaa, 2018a). For example, the seed grain aid imports from the Baltics arrived too late for sowing in 1697 because ice clogged the Ostrobothnian harbors long into spring or because the ships were wrecked in storms (Mäntylä, 1988; Lappalainen, 2012). Also, whereas the onset of successive years of poor harvests in 1601 and 1641 coincides with the estimated peaks in global radiative forcing and SAOD (Toohey and Sigl, 2017), this tight temporal correspondence between the estimated SAOD and the cold summer 1695 reconstructed over Ostrobothnia is not evident (Fig. 3). Thus, we cannot rule out the possibility that factors other than volcanic aerosol forcing may have contributed to the degree of harvest losses in that particular year. Indeed, dry summer and early autumn frosts damaged the harvest already in 1693 (Luukko, 1945). Furthermore, in addition to summer season temperature variability, pre-industrial crop cultivation in Finland was sensitive to winter severity and the related onset of the growing season (Huhtamaa et al., 2015; White et al., 2022). In years following long, cold, and snow-rich winters, the onset of the new growing season was badly delayed, and the crops may not have had time to ripen before the occurrence of the first autumn frosts (Huhtamaa and Ljungqvist, 2021). Notably, winter temperatures in Finland are influenced partly by the intensity of westerly airflow, and the latter is linked to the modes of the North Atlantic Oscillation (NAO) (Tuomenvirta et al., 2000). The years of negative winter NAO phases are strongly correlated with cooler winter temperatures and thicker ice and snow cover, with likely impacts including a later onset of springs and early summers due to ice–albedo feedbacks (Helama and Holopainen, 2012). A documentary-based NAO reconstruction (Luterbacher et al., 1999, 2001) indicates that the winter (i.e., December–February) 1694–95 NAO was among the most negative (−2.56; first percentile) over the period 1500–2000. Furthermore, ice break-up dates from the Torne River (65.84∘ N, 24.15∘ E) provide further evidence for an extremely cold winter and spring, as the ice broke up as late as 5 June 1695 (Kajander, 1993), i.e., at the second latest ice break-up date recorded since observations started in 1693. In the year of the latest ice break-up, 1867, extreme harvest failures and hunger were also witnessed in Ostrobothnia (Huhtamaa, 2018b). Thus, the substantial harvest losses in 1695 cannot likely be explained exclusively by the cold summer, but also by the extremely cold winter 1694–95 and the resulting delayed onset of the growing season, which may have been unrelated to the volcanic aerosol forcing.
The socioeconomic consequences varied considerably over time, space, and the peasant community (Fig. 5, Table 1). The moderate socioeconomic consequences following the 1695 unidentified eruption are rather unexpected in particular, as it is well established that the demographic effects of this crisis were devastating. One of the most calamitous famines in European history raged in Finland in 1696–1697, when over one-quarter of its population perished (Muroma, 1991). Furthermore, although the majority of the population gained their livelihood from agriculture, the severity of harvest losses did not always dictate the socioeconomic outcomes (Fig. 6). Thus, some other factors must have influenced whether the volcanic eruptions had strong or weak societal effects over different periods and locations, or among different societal groups. In order to reveal these influencing factors, we need to investigate the socio-environmental context with high spatial and temporal precision.
5.2 The historical context
Following the 1601 peak in volcanic forcing, the parishes with greatest harvest losses had the highest share of deserted holdings (Fig. 5a, d). This indicates that the crop failures had a direct influence on the socioeconomic conditions. The reason for this is likely connected to the overall stability of the society. The Swedish realm was in great distress at the turn of the century. The kingdom was at war with Russia, and Russian military raids caused destruction over northern Ostrobothnia (Luukko, 1945). Within the realm, King Sigismund and Duke Charles fought over the throne. These struggles materialized among the people in Ostrobothnia as well, as taxes increased and the peasants were obliged to provide maintenance and provisions for the troops passing through (Huhtamaa, 2018a). Furthermore, besides the increased fiscal burden and plundering soldiers, several crop failures emptied the grain stores in the 1590s. This political and military distress escalated with a peasant uprising in 1596–1597 in Ostrobothnia and a civil war in 1598–1599 in Sweden proper (Katajala, 2002). The turmoil likely fundamentally decreased the capacity to cope with the 1601 crop failure: institutions for providing aid were paralyzed, trade was disturbed, and grain could not be shipped from less affected regions (Huhtamaa, 2018a). The society was overall in a vulnerable state, and the troublesome times decreased the resilience of individuals. These factors likely explain why the harvest losses directly influenced socioeconomic conditions during the first volcanic event.
The direct spatial correspondence between harvest losses and desertion ratios weakens over the 1641 crisis (Fig. 5c, d). Furthermore, the share of deserted holdings starts to rise already in the first year of bad harvest (Fig. 4b). These matters imply that some other factors may have influenced the relationship between climate variability and socioeconomic consequences. The Swedish realm entered the Thirty Years War in 1630. The material and human resources for the war were raised mostly from the peasant society by increasing taxes and by conscripting peasants as soldiers, especially from the late 1630s onward (Villstrand, 2000; Myrdal, 2007). Thus, the main reason for the recorded tax debts in the 1640s may not have been linked to harvest failures, but to the raised overall tax burden. Also, the peasants likely had less grain for storing because of the raised taxes and other financial burdens of wartime, which may have negatively influenced individual coping capacity. Furthermore, the wartime may have also influenced agricultural productivity, which relied on man- and horsepower (Stoffel et al., 2022). During the years 1638–1649, altogether 4155 men were shipped from the study area to fight the war on the continent (Lappalainen, 1987). Thus, on average, almost each household was missing one adult man contributing to the labor supply, as there were approximately 4500 peasant holdings in the area at the time. This lack of manpower may have also hindered the correct timing of farm duties, which was crucial to secure a sufficient harvest (Mäkelä-Alitalo, 2003).
The harvest losses in 1695–1697 were dramatic across the study area, although the southern parishes seem to have coped slightly better than the northern ones (Fig. 5c). These parishes might have benefited from the grain magazines maintained by the regional authorities, where peasants could loan seed grain (Teerijoki, 1993). However, the mitigating impact of the southern granaries was likely rather marginal as even the less affected regions lost more than half of their harvest on average.
Despite the devastating harvest losses of the 1690s, the socioeconomic impacts were rather moderate when compared to the earlier crises (Fig. 5d). By the end of the century, Sweden had risen as one of Europe's great powers and was preparing for a war against Russia. The economy of the realm was largely financed by taxes and tributes paid by the peasantry (Lappalainen, 2012). Thus, the Crown could not afford to evict masses of peasants due to unpaid taxes, as in this sparsely populated kingdom there would not have been enough new people to take over the deserted farmsteads (Kujala, 2003). It was more reasonable for the realm to lose tax incomes for some individual years, instead of farmsteads being permanently deserted and not producing any tax payments in the future. Consequently, the Crown responded to the 1690s crisis with unusually extensive agro-economic relief measures in the form of tax reductions and deferments (Mäntylä, 1988). Furthermore, the authorities organized additional grain shipping and issued exemptions for Baltic Sea trade so as to increase the amount of imported grain (Mäntylä, 1988; Lappalainen, 2012). Yet, it is likely the volume of shipped grain was not sufficient, as the main export regions – like the Baltic provinces – also suffered from severe harvest losses (Seppel, 2015). Besides, the grain imports helped people living close to seaside towns and along the main transportation networks (Muroma, 1991), but the measures did not reach regions with poor road networks, like the central and easternmost regions of the study area (Fig. 5d). More importantly, not everyone was entitled to the shipped grain, as it was not given out for free, but either sold or given as a loan (Lappalainen, 2012). Unlike in many western European regions, where formal poor-relief institutions and charity organizations helped the poor to mitigate and cope with crop failures (Solar, 1995; van Bavel and Rijpma, 2016; Curtis and Dijkman, 2019; Dijkman, 2018; Jespersen, 2016), such formal institutions barely existed in early modern Sweden – and especially in its rural peripheries like Ostrobothnia. Thus, the rural poor were largely excluded from any institutional relief actions during the 17th century crises.
Although the socioeconomic consequences of the 1690s crisis were not substantial, they had severe demographic consequences. The extensive harvest failures triggered one of the worst famines in European history. In 1697–1698, population numbers dropped by 18 % in the southern parts and by 34 % in the central and northern parts of the study area (Muroma, 1991). The high mortality cannot, however, be explained solely by starvation per se, but by the spread of fatal hunger-related epidemics (Lappalainen, 2012). As there were no effective official poor-relief institutions, food aid in 17th century Ostrobothnia was heavily based on help that was provided by commoner to commoner. At times when a household had no food left, people left their dwellings to travel from house to house asking for food and a place to stay for the night. Thus, gaining help required mobility, which escalated the spread of infectious diseases. The differing degree of socioeconomic and demographic consequences following the 1695 event implies that there is not only one relevant measure of “volcanic impact on society”, but the intensity of the impact depends on the aspects of human life that are investigated.
When comparing the socioeconomic consequences between freeholders and Crown peasants, the deprivation is substantially more profound among the latter group. As the Crown holdings were commonly previously deserted or newly cleared farmsteads on the rural periphery, geographical factors may partly explain the differences. The location of these farmsteads, for example regarding soil properties and microclimate, might have simply been less suitable for crop cultivation than the locations of the freeholder farms (Muroma, 1991; Solantie, 2012). Furthermore, over the century subsidiary livelihood options, like sea and fresh-water fishing or tar burning, became increasingly important in Ostrobothnia. The additional financial assets from these activities may partly explain why certain areas had minor socioeconomic impacts during the 1690s crisis (Fig. 5d). However, the rights to practice these subsidiary activities were strictly controlled by the authorities, and new licenses were not commonly issued (Luukko, 1945; Virrankoski, 1973). Thus, the new Crown peasant settlers may not have had the same entitlement to these subsidiary sources for living as the freeholders, who had held the rights generation after generation.
Nonetheless, perhaps the most important factor explaining the differences in terms of socioeconomic consequences between the freeholders and the Crown peasants is immaterial: the continuity of contacts. The peasant families who had settled their holdings over multiple generations had a social status and respect within the community and beyond, which helped in keeping trade and credit relations with burghers of the towns (Luukko, 1945; Piilahti, 2007). The mutual trust resulting from these continuous contacts was crucial for the peasants, for example, for gaining loans from the burghers to purchase shipped grain during the 1690s crisis. As an essential element of sustaining these contacts was keeping the holding within one family over generations (Piilahti, 2007), newly settled Crown peasant farms or holdings with earlier tax debts were greatly excluded from these arrangements. Continuity may thus have played a role in farm management as well, as examples from other parts of pre-industrial Europe demonstrate (Sonderegger, 2020). Peasants with hereditary rights may have also been more motivated to invest into the productivity of a farm, and were likely more familiar with local challenges and ways to overcome these than newly settled Crown peasants.
5.3 Vulnerability and resilience
The investigation of the dynamic historical context revealed how different environmental, political, institutional, and cultural factors influenced the socioeconomic consequences of the 17th century eruptions. These components (which are detailed in the previous section) determined the societal and/or individual sensitivity to the adverse cold pulses triggered by volcanic eruptions and the capacity of the local populations to respond to and recover from these events, that is, the degree of vulnerability and resilience (Fig. 7).
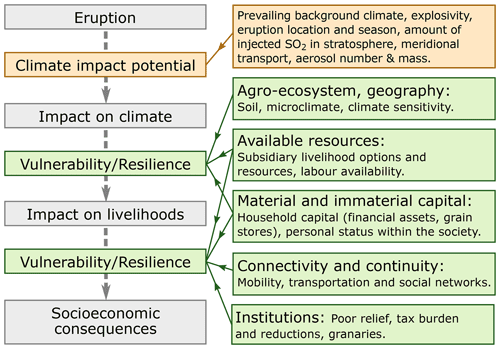
Figure 7Conceptual model linking eruption events to socioeconomic consequences (left), eruption characteristics influencing the climatic impact (right, orange), and components of vulnerability and resilience (right, green), which dictate the degree of socioeconomic impacts.
The concepts of vulnerability and resilience have received critical reassessment by historians over recent years (see, e.g., Bankoff, 2007; Haldon et al., 2020; van Bavel et al., 2020; Degroot et al., 2021). Soens (2018) has noted that these two concepts are not opposing or mutually exclusive, but that a resilient society can contain vulnerable people within. The socioeconomic consequences of the 1690s crisis demonstrate this concept: overall, the society seems to be socioeconomically resilient to the 1695 event, but closer investigation reveals one vulnerable societal group, the Crown peasants, among whom the societal hardships accumulated. Likewise, a livelihood system based on climate-sensitive crop cultivation, located in one of the northernmost agricultural areas of Europe, can be considered vulnerable in general. Nevertheless, the people who had access and entitlement to imported grain or could subside their livelihood from alternative resources were more resilient than the others. Thus, vulnerability and resilience coexist within societies, and the influence of these in explaining the societal impacts of volcanic eruptions depends on the scope of our investigation.
The components which influenced the peasant society's vulnerability and resilience were neither spatially nor temporally constant. Furthermore, the effect of these components differed depending on whether, for instance, the demographic or the socioeconomic consequences are investigated. In this regard, whereas the 1601 and the 1641 events had dire socioeconomic consequences, partly due to prevailing political and military circumstances, the tax deferments during the 1690s crisis moderated the ensuing socioeconomic effects. When viewed from a socioeconomic perspective, the authorities' tax relief actions can be seen as a successful institutional coping mechanism. However, when looked at from a demographic perspective, the measures that were directed only to the more advantaged segment of the peasant society had catastrophic consequences. The landless population and the less advantaged Crown peasants that were excluded from the relief measures faced hunger first, and related disease epidemics started to spread, eventually also reaching the wealthier freeholders (Mäntylä, 1988). Thus, although the authorities' relief actions increased socioeconomic resilience, they also increased the vulnerability to epidemic disease.
The model (Fig. 7) was developed from the so-called impact-order model used to detect societal consequences of the 1815 Tambora eruption (Krämer, 2015; Luterbacher and Pfister, 2015). The identified components of vulnerability and resilience, however, are relevant to the case study presented in this paper. Likely, alternative components will be identified if similar schemes are created to study the distant socioeconomic consequences of large eruptions within different societies. Nevertheless, it is also likely that some of these components are the same regardless of time and space. For example, similar to Ostrobothnia, in Scotland, poor soil quality, lack of poor-relief institutions, and socio-political distress made the society more vulnerable to the 1690s crisis (D'Arrigo et al., 2020). Consequently, further micro-regional research focusing on different eruption periods among different societies is needed, if the model is to be improved towards a more universally applicable one.
Historical examples can help to assess possible long-range societal impacts of future explosive volcanism and thus inform contingency planning (Oppenheimer, 2015; Riede, 2019). Additionally, past examples can advise policy makers on the unintended adverse human consequences of controversial climate change mitigation options, such as solar radiation modification (Kostick and Ludlow, 2020). Whereas archeologists have recently developed frameworks to investigate societal consequences of past eruptions (Riede, 2016, 2017, 2019), such theoretical work has scarcely been carried out within the discipline of history. However, historians are working with abundant and detailed documentary sources, which enable multi-decadal, or even multi-centennial, investigations with high spatial and temporal precision. When this high-resolution evidence is combined with careful historical contextualization, we are able to detect various material, institutional, and cultural changes and to estimate how these influenced the degree of vulnerability and resilience of societies to cope with volcanic cooling over time. This understanding can provide more nuanced perspectives than the macro-scale studies, which are common in the field, and thus contribute to political decision-making and enhance scientific outreach.
Various natural and written sources, like tree-ring data, grain tithe accounts, and tax debt records used in this study, enable rigorous investigation of the climatic and societal impacts of past volcanic eruptions at a given location. By applying this micro-regional approach, we evidenced that the eruption–climate–society causalities are not as straightforward as they might appear at first sight.
Here, we demonstrated that the summer temperature cooling following the three major distal 17th century eruptions had significant agricultural and socioeconomic impacts on contemporary Ostrobothnia. The eruptions of Huaynaputina in 1600 and Koma-ga-take–Mount Parker in 1640/41 coincide with the coldest decades of the 17th century. Whereas we reconstruct marked cooling between 1601–1610 and 1641–1646, this cooling should not be ascribed to the volcanic eruptions alone but regarded in its broader climatic context with the deteriorating climatic conditions of the Maunder Minimum. Thus, the societal impacts observed during these periods should not be solely attributed to volcanism either. Moreover, the relationship between harvest failures and socioeconomic repercussions was not direct – despite the fact that local populations gained their main livelihood substantially from climate-sensitive agriculture. Although the volcanic cold pulses in 1601 and 1641 – and to a lesser degree in 1695 – may have acted as triggers, the answers to the questions of why, where, and among whom the situation escalated into a crisis are far more complex. We found that the varying degrees of vulnerability and resilience, which were influenced by the prevailing agro-ecosystem, resources, capital, physical and social networks, and institutions, determined whether the eruption-related cold pulses had strong or weak effects on human life. Importantly, these components were not mutually exclusive. For example, the same components that increased the peasant society's socioeconomic resilience to cope with harvest failures likely increased the vulnerability to hunger-related disease epidemics. Additionally, the policies on receiving grain aid helped the wealthier freeholder peasants with good social networks, whereas the same practices made the less advantaged Crown peasants more vulnerable to crop failure. Moreover, we found that many components that obviously influenced the degree to which societal impacts were felt are difficult to quantify. For example, political stability, continuity of personal relations, and institutional practices were such factors in 17th century Ostrobothnia. Thus, understanding the distal societal consequences of past volcanic eruptions requires both quantitative and qualitative assessments.
Previous research has focused mainly on detecting famine and societal collapse following past volcanic eruptions, with limited critical assessment on the differences between coincidence and causation. Although such examples can be captivating, lessons from pre-modern large-scale crises might be difficult to adapt to today's societies. A focus on vulnerability and resilience, and how these influenced the dynamics of how eruption events materialized at the grassroots level, may provide more applicable lessons. Needless to say, history cannot predict the future. Yet the past can provide tangible examples and consequently advise decision-makers on the various long-range human consequences of future volcanic eruptions.
The tree-ring datasets used in this study (Schweingruber et al., 1988, https://doi.org/10.1111/j.1502-3885.1988.tb00569.x; Kirchhefer, 2001, https://doi.org/10.1191/095968301670181592; Esper et al., 2012, https://doi.org/10.1016/j.gloplacha.2012.01.006; Melvin et al., 2013, https://doi.org/10.1177/0959683612460791; Schneider et al., 2015, https://doi.org/10.1002/2015GL063956) are freely available on the NOAA repository https://www.ncdc.noaa.gov/data-access/paleoclimatology-data/datasets/tree-ring (last access: 29 August 2022); the E-OBS datasets (Cornes et al., 2018, https://doi.org/10.1029/2017JD028200) can be downloaded at https://www.ecad.eu/download/ensembles/download.php (last access: 29 August 2022). The original archival material for the tithe and desertion data is publicly available at the National Archives of Sweden (tithe records for 1631–40) and at the National Archives of Finland (tithe and desertion records for the rest of the study period).
The supplement related to this article is available online at: https://doi.org/10.5194/cp-18-2077-2022-supplement.
HH was responsible for the initial study design, historical data collection, analysis and interpretation, and manuscript preparation. CC and MS performed the tree-ring reconstruction and climatic analyses. All authors have worked on the manuscript and approved the submitted version.
The contact author has declared that none of the authors has any competing interests.
Publisher’s note: Copernicus Publications remains neutral with regard to jurisdictional claims in published maps and institutional affiliations.
This article is part of the special issue “Interdisciplinary studies of volcanic impacts on climate and society”. It is not associated with a conference.
This research benefited from participation in the Volcanic Impacts on Climate and Society (VICS) and the Climate Reconstruction and Impacts from the Archives of Societies (CRIAS) working groups of the Past Global Changes (PAGES) project. The research work of Christophe Corona and Markus Stoffel was supported by the Swiss National Science Foundation (SNSF, grant no. CRSII5_183571). The initial archival work of Heli Huhtamaa was supported by the European Research Council-sponsored project “Coordinating for Life” (grant no. 339647), and the finalizing of the manuscript was supported by the SNSF (grant no. PZ00P1_201953) and by the Swiss State Secretariat for Education, Research and Innovation under the contract no. MB22.00030.
This research has been supported by the Schweizerischer Nationalfonds zur Förderung der Wissenschaftlichen Forschung (grant no. PZ00P1_201953) and the Staatssekretariat für Bildung, Forschung und Innovation (contract no. MB22.00030).
This paper was edited by Francis Ludlow and reviewed by Joseph Manning and Katrin Kleemann.
Auchmann, R., Brönnimann, S., Breda, L., Bühler, M., Spadin, R., and Stickler, A.: Extreme climate, not extreme weather: the summer of 1816 in Geneva, Switzerland, Clim. Past, 8, 325–335, https://doi.org/10.5194/cp-8-325-2012, 2012. a
Bankoff, G.: Comparing vulnerabilities: toward charting an historical trajectory of disasters, Hist. Soc. Res., 32, 103–114, https://doi.org/10.12759/hsr.32.2007.3.103-114, 2007. a
Briffa, K. R., Jones, P. D., Schweingruber, F. H., and Osborn, T. J.: Influence of volcanic eruptions on Northern Hemisphere summer temperature over the past 600 years, Nature, 393, 450–455, https://doi.org/10.1038/30943, 1998. a, b
Brönnimann, S. and Krämer, D.: Tambora and the “Year Without a Summer” of 1816. A Perspective on Earth and Human Systems Science, Geographica Bernensia, 90, 48 pp., https://doi.org/10.4480/GB2016.G90.01, 2016. a
Büntgen, U., Arseneault, D., Boucher, É., Churakova, O. V., Gennaretti, F., Crivellaro, A., Hughes, M. K., Kirdyanov, A. V., Klippel, L., Krusic, P. J., Linderholm, H. W., Ljungqvist, F. C., Ludescher, J., McCormick, M., Myglan, V. S., Nicolussi, K., Piermattei, A., Oppenheimer, C., Reinig, F., Sigl, M., Vaganov, E. A., and Esper, J.: Prominent role of volcanism in Common Era climate variability and human history, Dendrochronologia, 64, 125757, https://doi.org/10.1016/j.dendro.2020.125757, 2020. a
Campbell, B. M.: Global climates, the 1257 Mega-eruption of Samalas Volcano, Indonesia, and the English food crisis of 1258, T. Roy. Hist. Soc., 27, 87–121, https://doi.org/10.1017/S0080440117000056, 2017. a
Cole-Dai, J.: Volcanoes and climate, WIREs Clim Change, 1, 824–839, https://doi.org/10.1002/wcc.76, 2010. a
Cornes, R. C., van der Schrier, G., van den Besselaar, E. J. M., and Jones, P. D.: An ensemble version of the E-OBS temperature and precipitation data sets, J. Geophys. Res.-Atmos., 123, 9391–9409, https://doi.org/10.1029/2017JD028200, 2018 (data available at: https://www.ecad.eu/download/ensembles/download.php, last access: 29 August 2022). a, b
Curtis, D. R. and Dijkman, J.: The escape from famine in the Northern Netherlands: A reconsideration using the 1690s harvest failures and a broader Northwest European perspective, Seventeenth Cent., 34, 229–258, https://doi.org/10.1080/0268117X.2017.1410494, 2019. a
D'Arrigo, R., Wilson, R., and Anchukaitis, K. J.: Volcanic cooling signal in tree ring temperature records for the past millennium, J. Geophys. Res.-Atmos., 118, 9000–9010, https://doi.org/10.1002/jgrd.50692, 2013. a, b
D'Arrigo, R., Klinger, P., Newfield, T., Rydval, M., and Wilson, R.: Complexity in crisis: The volcanic cold pulse of the 1690s and the consequences of Scotland's failure to cope, J. Volcanol. Geoth. Res., 389, 106746, https://doi.org/10.1016/j.jvolgeores.2019.106746, 2020. a, b, c
Degroot, D., Anchukaitis, K., Bauch, M., Burnham, J., Carnegy, F., Cui, J., de Luna, K., Guzowski, P., Hambrecht, G., Huhtamaa, H., Izdebski, A., Kleemann, K., Moesswilde, E., Neupane, N., Newfield, T., Pei, Q., Xoplaki, E., and Zappia, N.: Towards a rigorous understanding of societal responses to climate change, Nature, 591, 539–550, https://doi.org/10.1038/s41586-021-03190-2, 2021. a, b, c
Demarée, G. R. and Ogilvie, A. E.: Bons Baisers d’Islande: Climatic, environmental, and human dimensions impacts of the Lakagígar eruption (1783–1784) in Iceland, in: History and Climate, edited by: Jones, P. D., Ogilvie, A. E. J., Davies, T. D., and Briffa, K. R., Springer, 219–246, https://doi.org/10.1007/978-1-4757-3365-5_11, 2001. a
Dijkman, J.: Bread for the poor: Poor relief and the mitigation of the food crises of the 1590s and the 1690s in Berkel, Holland, in: Famines During the 'Little Ice Age' (1300–1800), edited by: Collet, D. and Schuh, M., Springer, 171–193, https://doi.org/10.1007/978-3-319-54337-6_9, 2018. a
Esper, J., Büntgen, U., Timonen, M., and Frank, D. C.: Variability and extremes of northern Scandinavian summer temperatures over the past two millennia, Global Planet. Change, 88, 1–9, https://doi.org/10.1016/j.gloplacha.2012.01.006, 2012 (data available at: https://www.ncdc.noaa.gov/data-access/paleoclimatology-data/datasets/tree-ring, last access: 29 August 2022). a, b
Esper, J., Schneider, L., Krusic, P. J., Luterbacher, J., Büntgen, U., Timonen, M., Sirocko, F., and Zorita, E.: European summer temperature response to annually dated volcanic eruptions over the past nine centuries, B. Volcanol., 75, 736, https://doi.org/10.1007/s00445-013-0736-z, 2013. a
Fei, J., Zhang, D. D., and Lee, H. F.: 1600 AD Huaynaputina eruption (Peru), abrupt cooling, and epidemics in China and Korea, Adv. Meteorol., 2016, 3217038, https://doi.org/10.1155/2016/3217038, 2016. a
Fischer, E. M., Luterbacher, J., Zorita, E., Tett, S., Casty, C., and Wanner, H.: European climate response to tropical volcanic eruptions over the last half millennium, Geophys. Res. Lett., 34, L05707, https://doi.org/10.1029/2006GL027992, 2007. a
Gao, C., Robock, A., and Ammann, C.: Volcanic forcing of climate over the past 1500 years: An improved ice core-based index for climate models, J. Geophys. Res., 113, D23111, https://doi.org/10.1029/2008JD010239, 2008. a
Grattan, J., Michnowicz, S., and Rabartin, R.: The long shadow: understanding the influence of the Laki fissure eruption on human mortality in Europe, in: Living under the shadow: cultural impacts of volcanic eruptions, edited by: Grattan, J. and Torrence, R., Routledge, 153–175, ISBN: 9781598742695, 2007. a
Guillet, S., Corona, C., Stoffel, M., Khodri, M., Lavigne, F., Ortega, P., Eckert, N., Sielenou, P. D., Daux, V., Churakova, O. V., Davi, N., Edouard, J.-L., Zhang, Y., Luckman, B. H., Myglan, V. S., Guiot, J., Beniston, M., Masson-Delmotte, V., and Oppenheimer, C.: Climate response to the Samalas volcanic eruption in 1257 revealed by proxy records, Nat. Geosci., 10, 123–128, https://doi.org/10.1038/ngeo2875, 2017. a
Guillet, S., Corona, C., Ludlow, F., Oppenheimer, C., and Stoffel, M.: Climatic and societal impacts of a “forgotten” cluster of volcanic eruptions in 1108–1110 CE, Scientific Reports, 10, 6715, https://doi.org/10.1038/s41598-020-63339-3, 2020. a
Haldon, J.: Cooling and societal change, Nat. Geosci., 9, 191–192, https://doi.org/10.1038/ngeo2659, 2016. a
Haldon, J., Eisenberg, M., Mordechai, L., Izdebski, A., and White, S.: Lessons from the past, policies for the future: resilience and sustainability in past crises, Environ. Syst. Decis., 40, 287–297, https://doi.org/10.1007/s10669-020-09778-9, 2020. a
Hallenberg, M., Holm, J., and Johansson, D.: Organization, legitimation, participation: State formation as a dynamic process – The Swedish example, c. 1523–1680, Scand. J. Hist., 33, 247–268, https://doi.org/10.1080/03468750802150242, 2008. a, b
Helama, S. and Holopainen, J.: Spring temperature variability relative to the North Atlantic Oscillation and sunspots – a correlation analysis with a Monte Carlo implementation, Palaeogeogr. Palaeocl., 326, 128–134, https://doi.org/10.1016/j.palaeo.2012.02.013, 2012. a
Helama, S., Melvin, T. M., and Briffa, K. R.: Regional curve standardization: State of the art, The Holocene, 27, 172–177, https://doi.org/10.1177/0959683616652709, 2017. a
Huhtamaa, H.: Combining written and tree-ring evidence to trace past food crises: A case study from Finland, in: Famines During the “Little Ice Age” (1300–1800), edited by: Collet, D. and Maximilian, S., Springer, 43–66, https://doi.org/10.1007/978-3-319-54337-6_3, 2018a. a, b, c
Huhtamaa, H.: “Kewät kolkko, talwi tuima” – Ilmasto, sää ja sadot nälkävuosien taustalla, in: Nälkävuodet 1867–1868, edited by: Jussila, T. and Rantanen, L., Suomalaisen Kirjallisuuden Seura, 33–65, ISBN: 9789518580150, 2018b. a
Huhtamaa, H. and Helama, S.: Distant impact: tropical volcanic eruptions and climate-driven agricultural crises in seventeenth-century Ostrobothnia, Finland, J. Hist. Geogr., 57, 40–51, https://doi.org/10.1016/j.jhg.2017.05.011, 2017. a, b
Huhtamaa, H. and Ljungqvist, F. C.: Climate in Nordic historical research – a research review and future perspectives, Scand. J. Hist., 46, 665–695, https://doi.org/10.1080/03468755.2021.1929455, 2021. a
Huhtamaa, H., Helama, S., Holopainen, J., Rethorn, C., and Rohr, C.: Crop yield responses to temperature fluctuations in 19th century Finland: provincial variation in relation to climate and tree-rings, Boreal Environ. Res., 20, 707–723, 2015. a, b
Huhtamaa, H., Helama, S., Leijonhufvud, L., and Ljungqvist, F. C.: Combining the archives of nature and society: Tree rings and tithes, PAGES Magazine, 28, 20–21, https://doi.org/10.22498/pages.28.2.50, 2020. a
Jespersen, K. J. V.: Social consequences, in: The Cambridge History of Scandinavia: Volume 2, 1520–1870, edited by: Kouri, E. I. and Olesen, J. E., Cambridge University Press, 192–210, https://doi.org/10.1017/CHO9781139031639.013, 2016. a
Johanson, V. F.: Finlands agrarpolitiska historia: en skildring av det finländska lantbrukets ekonomiska betingelser. Från 1600-talet till år 1870, Suomen Maataloustieteellinen Seura, 1924 (in Swedish). a
Jones, P., Moberg, A., Osborn, T., and Briffa, K.: Surface climate responses to explosive volcanic eruptions seen in long European temperature records and mid-to-high latitude tree-ring density around the Northern Hemisphere, in: Volcanism and the Earth's Atmosphere, edited by: Robock, A. and Oppenheimer, C., American Geophysical Union, Geoph. Monog. Series, 139, 239–254, https://doi.org/10.1029/139GM15, 2003. a
Kajander, J.: Methodological aspects on river cryophenology exemplified by a tricentennial break-up time series from Tornio, Geophysica, 29, 73–95, 1993. a
Katajala, K.: Suomalainen kapina: Talonpoikaislevottomuudet ja poliittisen kulttuurin muutos Ruotsin ajalla (n. 1150–1800), Suomalaisen Kirjallisuuden Seura, ISBN: 9517463065, 2002 (in Finnish). a
Kirchhefer, A. J.: Reconstruction of summer temperatures from tree-rings of Scots pine (Pinus sylvestris L.) in coastal northern Norway, The Holocene, 11, 41–52, https://doi.org/10.1191/095968301670181592, 2001 (data available at: https://www.ncdc.noaa.gov/data-access/paleoclimatology-data/datasets/tree-ring, last access: 29 August 2022). a, b
Kostick, C. and Ludlow, F.: Medieval History, Explosive Volcanism, and the Geoengineering Debate, in: Making the Medieval Relevant, edited by: Jones, C., Kostick, C., and Oschema, K., De Gruyter, Berlin, 45–98, https://doi.org/10.1515/9783110546316-003, 2020. a
Krämer, D.: “Menschen grasten nun mit dem Vieh”: Die letzte grosse Hungerkrise der Schweiz 1816/17, Schwabe AG, ISBN: 9783796533754, 2015 (in German). a, b
Kujala, A.: The Crown, the Nobility and the Peasants, 1630–1713: Tax, rent and relations of power, Suomalaisen Kirjallisuuden Seura, ISBN: 9517464738, 2003. a, b, c, d
Lappalainen, J. T.: Valtiopäiviltä väenottopaikalle. Suomen jalkaväen rekrytointi 1638–1649, Tiede ja ase, 45, 62–84, 1987 (in Finnish). a
Lappalainen, M.: Jumalan vihan ruoska: Suuri nälänhätä Suomessa 1695–1697, Siltala, ISBN: 9789522344441, 2012 (in Finnish). a, b, c, d, e
Le Roy Ladurie, E. and Goy, J.: Tithe and Agrarian History from the Fourteenth to the Nineteenth Century: An Essay in Comparative History, Cambridge University Press, ISBN: 0521239745, 1982. a
Leijonhufvud, L.: Grain tithes and manorial yields in early modern Sweden, PhD thesis, Swedish University of Agricultural Sciences, ISBN: 9157658293, 2001. a
Ljungqvist, F. C., Seim, A., and Huhtamaa, H.: Climate and society in European history, WIREs Clim Change, 12, e691, https://doi.org/10.1002/wcc.691, 2021. a
Luterbacher, J. and Pfister, C.: The year without a summer, Nat. Geosci., 8, 246–248, https://doi.org/10.1038/ngeo2404, 2015. a, b
Luterbacher, J., Schmutz, C., Gyalistras, D., Xoplaki, E., and Wanner, H.: Reconstruction of monthly NAO and EU indices back to AD 1675, Geophys. Res. Lett., 26, 2745–2748, https://doi.org/10.1029/1999GL900576, 1999. a
Luterbacher, J., Xoplaki, E., Dietrich, D., Jones, P., Davies, T., Portis, D., Gonzalez-Rouco, J., Von Storch, H., Gyalistras, D., Casty, C., and Wanner, H.: Extending North Atlantic oscillation reconstructions back to 1500, Atmos. Sci. Lett., 2, 114–124, https://doi.org/10.1006/asle.2002.0047, 2001. a
Luukko, A.: Etelä-Pohjanmaan historia: 3, Nuijasodasta isoonvihaan, Etelä-Pohjanmaan historiatoimikunta, 1945 (in Finnish). a, b, c, d
Mäkelä-Alitalo, A.: Verotus, autioituminen ja väenotot, in: Suomen maatalouden historia 1, edited by: Rasila, V., Jutikkala, E., and Mäkelä-Alitalo, A., Suomalaisen Kirjallisuuden Seura, 183–206, ISBN: 9517464800, 2003 (in Finnish). a
Mäntylä, I.: Kruunu ja alamaisten nälkä: 1690-luvun katovuosien verotulojen vähennys Pohjanmaalla ja esivallan vastatoimenpiteet, Oulun Historiaseura, ISBN: 9519571388, 1988 (in Finnish). a, b, c, d, e
Melvin, T. M., Grudd, H., and Briffa, K. R.: Potential bias in 'updating' tree-ring chronologies using regional curve standardisation: Re-processing 1500 years of Torneträsk density and ring-width data, The Holocene, 23, 364–373, https://doi.org/10.1177/0959683612460791, 2013 (data available at: https://www.ncdc.noaa.gov/data-access/paleoclimatology-data/datasets/tree-ring, last access: 29 August 2022). a, b
Muroma, S.: Suurten kuolonvuosien väestönmenetys Suomessa, Suomen Historiallinen Seura, ISBN: 9518915474, 1991 (in Finnish). a, b, c, d, e, f
Myrdal, J.: Food, war, and crisis: The seventeenth century Swedish empire, in: Rethinking Environmental History, edited by: Hornborg, A., McNeill, J. R., and Martinez-Alier, J., AltaMira Press Lanham, MD, 79–98, ISBN: 9780759110281, 2007. a
Olsson, M. and Svensson, P.: Agricultural growth and institutions: Sweden, 1700–1860, Eur. Rev. Econ. Hist., 14, 275–304, https://doi.org/10.1017/S1361491610000067, 2010. a
Oppenheimer, C.: Climatic, environmental and human consequences of the largest known historic eruption: Tambora volcano (Indonesia) 1815, Prog. Phys. Geog., 27, 230–259, https://doi.org/10.1191/0309133303pp379ra, 2003. a
Oppenheimer, C.: Eruption politics, Nat. Geosci, 8, 244–245, https://doi.org/10.1038/ngeo2408, 2015. a, b
Parker, G.: Global Crisis: War, Climate Change, & Catastrophe in the Seventeenth Century, Yale University Press, ISBN: 9780300219364, 2013. a, b
Piilahti, K.-M.: Aineellista ja aineetonta turvaa: Ruokakunnat, ekologis-taloudelliset resurssit ja kontaktinmuodostus Valkealassa 1630–1750, PhD thesis, University of Helsinki, ISBN: 9789517468862, 2007 (in Finnish). a, b
Riede, F.: Volcanic activity and human society, Quatern. Int., 394, 1–5, https://doi.org/10.1016/j.quaint.2015.08.090, 2016. a
Riede, F.: Past-forwarding ancient calamities. Pathways for making archaeology relevant in disaster risk reduction research, Humanities, 6, 79, https://doi.org/10.3390/h6040079, 2017. a
Riede, F.: Doing palaeo-social volcanology: Developing a framework for systematically investigating the impacts of past volcanic eruptions on human societies using archaeological datasets, Quatern. Int., 499, 266–277, https://doi.org/10.1016/j.quaint.2018.01.027, 2019. a, b
Robock, A.: Volcanic eruptions and climate, Rev. Geophys., 38, 191–219, https://doi.org/10.1029/1998RG000054, 2000. a, b
Robock, A. and Free, M. P.: Ice cores as an index of global volcanism from 1850 to the present, J. Geophys. Res., 100, 11549–11567, https://doi.org/10.1029/95JD00825, 1995. a
Robock, A. and Mao, J.: The volcanic signal in surface temperature observations, J. Climate, 8, 1086–1103, https://doi.org/10.1175/1520-0442(1995)008<1086:TVSIST>2.0.CO;2, 1995. a, b
Schneider, D. P., Ammann, C. M., Otto-Bliesner, B. L., and Kaufman, D. S.: Climate response to large, high-latitude and low-latitude volcanic eruptions in the Community Climate System Model, J. Geophys. Res., 114, D15101, https://doi.org/10.1029/2008JD011222, 2009. a
Schneider, L., Smerdon, J. E., Büntgen, U., Wilson, R. J. S., Myglan, V. S., Kirdyanov, A. V., and Esper, J.: Revising midlatitude summer temperatures back to AD 600 based on a wood density network, Geophys. Res. Lett., 42, 4556–4562, https://doi.org/10.1002/2015GL063956, 2015 (data available at: https://www.ncdc.noaa.gov/data-access/paleoclimatology-data/datasets/tree-ring, last access: 29 August 2022). a, b
Schweingruber, F. H., Bartholin, T., Schaur, E., and Briffa, K. R.: Radiodensitometric-dendroclimatological conifer chronologies from Lapland (Scandinavia) and the Alps (Switzerland), Boreas, 17, 559–566, https://doi.org/10.1111/j.1502-3885.1988.tb00569.x, 1988 (data available at: https://www.ncdc.noaa.gov/data-access/paleoclimatology-data/datasets/tree-ring, last access: 29 August 2022). a, b
Seppel, M.: Feeding the motherland: grain exports from the Swedish Baltic provinces during the Great Famine of 1696–1697, Scandinavian Economic History Review, 63, 215–234, https://doi.org/10.1080/03585522.2015.1081855, 2015. a
Sigl, M., Winstrup, M., McConnell, J. R., Welten, K. C., Plunkett, G., Ludlow, F., Büntgen, U., Caffee, M., Chellman, N., Dahl-Jensen, D., Fischer, H., Kipfstuhl, S., Kostick, C., Maselli, O. J., Mekhaldi, F., Mulvaney, R., Muscheler, R., Pasteris, D. R., Pilcher, J. R., Salzer, M., Schüpbach, S., Steffensen, J. P., Vinther, B. M., and Woodruff, T. E.: Timing and climate forcing of volcanic eruptions for the past 2,500 years, Nature, 523, 543–549, https://doi.org/10.1038/nature14565, 2015. a, b, c, d
Soens, T.: Resilient societies, vulnerable people: coping with North Sea floods before 1800, Past Present, 241, 143–177, https://doi.org/10.1093/pastj/gty018, 2018. a
Soininen, A. M.: Vanha maataloutemme: Maatalous ja maatalousväestö Suomessa perinnäisen maatalouden loppukaudella 1720-luvulta 1870-luvulle, Suomen Historiallinen Seura, ISBN: 951925048, 1974 (in Finnish). a, b, c
Solantie, R.: Ilmasto ja sen määräämät luonnonolot Suomen asutuksen ja maatalouden historiassa, PhD thesis, University of Jyväskylä, ISBN: 9789513950095, 2012 (in Finnish). a
Solar, P. M.: Poor relief and English economic development before the industrial revolution, Econ. Hist. Rev., 48, 1–22, https://doi.org/10.2307/2597868, 1995. a
Sonderegger, S.: Active Manorial Lords and Peasant Farmers in the Economic Life of the Late Middle Ages: Results from New Swiss and German Research, in: Peasants, Lords, and State: Comparing Peasant Conditions in Scandinavia and the Eastern Alpine Region, 1000–1750, edited by: Ivarsen, T., Myknig, J. R., and Sonderegger, S., Brill, 292–318, https://doi.org/10.1163/9789004433458_012, ISBN: 9789004429703, 2020. a
Stoffel, M., Khodri, M., Corona, C., Guillet, S., Poulain, V., Bekki, S., Guiot, J., Luckman, B. H., Oppenheimer, C., Lebas, N., Beniston, M., and Masson-Delmotte, V.: Estimates of volcanic-induced cooling in the Northern Hemisphere over the past 1,500 years, Nat. Geosci., 8, 784–788, https://doi.org/10.1038/ngeo2526, 2015. a, b
Stoffel, M., Corona, C., Ludlow, F., Sigl, M., Huhtamaa, H., Garnier, E., Helama, S., Guillet, S., Crampsie, A., Kleemann, K., Camenisch, C., McConnell, J., and Gao, C.: Climatic, weather, and socio-economic conditions corresponding to the mid-17th-century eruption cluster, Clim. Past, 18, 1083–1108, https://doi.org/10.5194/cp-18-1083-2022, 2022. a, b, c
Stothers, R. B.: Climatic and demographic consequences of the massive volcanic eruption of 1258, Clim. Change, 45, 361–374, https://doi.org/10.1023/A:1005523330643, 2000. a
Swingedouw, D., Mignot, J., Ortega, P., Khodri, M., Menegoz, M., Cassou, C., and Hanquiez, V.: Impact of explosive volcanic eruptions on the main climate variability modes, Global Planet. Change, 150, 24–45, https://doi.org/10.1016/j.gloplacha.2017.01.006, 2017. a
Teerijoki, I.: Nälkävuosien turva? Pitäjänmakasiinit Suomessa 1700-luvulla, Suomen historiallinen Seura, ISBN: 9518915776, 1993 (in Finnish). a
Timmreck, C.: Modeling the climatic effects of large explosive volcanic eruptions, WIREs Clim Change, 3, 545–564, https://doi.org/10.1002/wcc.192, 2012. a
Toohey, M. and Sigl, M.: Volcanic stratospheric sulfur injections and aerosol optical depth from 500 BCE to 1900 CE, Earth Syst. Sci. Data, 9, 809–831, https://doi.org/10.5194/essd-9-809-2017, 2017. a, b, c, d, e, f, g, h
Tuomenvirta, H., Alexandersson, H., Drebs, A., Frich, P., and Nordli, P. O.: Trends in Nordic and Arctic temperature extremes and ranges, J. Climate, 13, 977–990, https://doi.org/10.1175/1520-0442(2000)013<0977:TINAAT>2.0.CO;2, 2000. a
Ulkuniemi, M. and Thomasson, L.: Ljungo Tuomaanpojan lainsuomennokset: Maanlain ja kaupunginlain teksti, Suomalaisen Kirjallisuuden Seura, ISBN: 9516830374, 1975 (in Finnish). a
van Bavel, B. and Rijpma, A.: How important were formalized charity and social spending before the rise of the welfare state? A long-run analysis of selected western E uropean cases, 1400–1850, Econ. Hist. Rev., 69, 159–187, https://doi.org/10.1111/ehr.12111, 2016. a
van Bavel, B., Curtis, D. R., Dijkman, J., Hannaford, M., de Keyzer, M., Van Onacker, E., and Soens, T.: Disasters and History: The Vulnerability and Resilience of Past Societies, Cambridge University Press, ISBN: 9781108477178, 2020. a, b
Venzke, E., Wunderman, R., McClelland, L., Simkin, T., Luhr, J., Siebert, L., Mayberry, G., and Sennert, S.: Global Volcanism Program, Volcanoes of the World, v. 4.9.1, Smithsonian Institution, https://doi.org/10.5479/si.GVP.VOTW4-2013, 2013. a
Villstrand, N. E.: Adaptation or Protestation: Local Community Facing the Conscription of Infantry for the Swedish Armed Forces, 1620–1679, in: A Revolution from Above? The Power State of 16th and 17th Century Scandinavia, edited by: Jespersen, L., Odense University Press, 253–313, ISBN: 8778384079, 2000. a
Virrankoski, P.: Pohjois-Pohjanmaan ja Lapin historia: 3, Pohjois-Pohjanmaa ja Lappi 1600-luvulla, Pohjois-Pohjanmaan, Kainuun ja Lapin maakuntaliittojen yhteinen historiatoimikunta, 1973 (in Finnish). a
Voipio, V.: Katovuosi 1601 vaikutuksineen Varsinais-Suomessa, Hist. Arkist., 24, 1–37, 1914. a
Warde, P.: Global crisis or global coincidence?, Past Present, 228, 287–301, https://doi.org/10.1093/pastj/gtv028, 2015. a
White, S., Moreno-Chamarro, E., Zanchettin, D., Huhtamaa, H., Degroot, D., Stoffel, M., and Corona, C.: The 1600 CE Huaynaputina eruption as a possible trigger for persistent cooling in the North Atlantic region, Clim. Past, 18, 739–757, https://doi.org/10.5194/cp-18-739-2022, 2022. a, b, c
Witham, C. S. and Oppenheimer, C.: Mortality in England during the 1783–4 Laki Craters eruption, B. Volcanol., 67, 15–26, https://doi.org/10.1007/s00445-004-0357-7, 2004. a
Zanchettin, D., Bothe, O., Graf, H. F., Lorenz, S. J., Luterbacher, J., Timmreck, C., and Jungclaus, J. H.: Background conditions influence the decadal climate response to strong volcanic eruptions, J. Geophys. Res.-Atmos., 118, 4090–4106, https://doi.org/10.1002/jgrd.50229, 2013a. a, b
Zanchettin, D., Timmreck, C., Bothe, O., Lorenz, S. J., Hegerl, G., Graf, H.-F., Luterbacher, J., and Jungclaus, J. H.: Delayed winter warming: A robust decadal response to strong tropical volcanic eruptions?, Geophys. Res. Lett., 40, 204–209, https://doi.org/10.1029/2012GL054403, 2013b. a
- Abstract
- Introduction
- Local perspective on the “global crisis” – Ostrobothnia in the 17th century
- Materials and methods
- Results
- Discussion
- Conclusions
- Data availability
- Author contributions
- Competing interests
- Disclaimer
- Special issue statement
- Acknowledgements
- Financial support
- Review statement
- References
- Supplement
- Abstract
- Introduction
- Local perspective on the “global crisis” – Ostrobothnia in the 17th century
- Materials and methods
- Results
- Discussion
- Conclusions
- Data availability
- Author contributions
- Competing interests
- Disclaimer
- Special issue statement
- Acknowledgements
- Financial support
- Review statement
- References
- Supplement