the Creative Commons Attribution 4.0 License.
the Creative Commons Attribution 4.0 License.
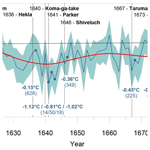
Climatic, weather, and socio-economic conditions corresponding to the mid-17th-century eruption cluster
Christophe Corona
Francis Ludlow
Michael Sigl
Heli Huhtamaa
Emmanuel Garnier
Samuli Helama
Sébastien Guillet
Arlene Crampsie
Katrin Kleemann
Chantal Camenisch
Joseph McConnell
Chaochao Gao
The mid-17th century is characterized by a cluster of explosive volcanic eruptions in the 1630s and 1640s, climatic conditions culminating in the Maunder Minimum, and political instability and famine in regions of western and northern Europe as well as China and Japan. This contribution investigates the sources of the eruptions of the 1630s and 1640s and their possible impact on contemporary climate using ice core, tree-ring, and historical evidence but will also look into the socio-political context in which they occurred and the human responses they may have triggered. Three distinct sulfur peaks are found in the Greenland ice core record in 1637, 1641–1642, and 1646. In Antarctica, only one unambiguous sulfate spike is recorded, peaking in 1642. The resulting bipolar sulfur peak in 1641–1642 can likely be ascribed to the eruption of Mount Parker (6∘ N, Philippines) on 26 December 1640, but sulfate emitted from Komaga-take (42∘ N, Japan) volcano on 31 July 1641 has potentially also contributed to the sulfate concentrations observed in Greenland at this time. The smaller peaks in 1637 and 1646 can be potentially attributed to the eruptions of Hekla (63∘ N, Iceland) and Shiveluch (56∘ N, Russia), respectively. To date, however, none of the candidate volcanoes for the mid-17th century sulfate peaks have been confirmed with tephra preserved in ice cores. Tree-ring and written sources point to cold conditions in the late 1630s and early 1640s in various parts of Europe and to poor harvests. Yet the early 17th century was also characterized by widespread warfare across Europe – and in particular the Thirty Years' War (1618–1648) – rendering any attribution of socio-economic crisis to volcanism challenging. In China and Japan, historical sources point to extreme droughts and famines starting in 1638 (China) and 1640 (Japan), thereby preceding the eruptions of Komaga-take (31 July 1640) and Mount Parker (4 January 1641). The case of the eruption cluster between 1637 and 1646 and the climatic and societal conditions recorded in its aftermath thus offer a textbook example of difficulties in (i) unambiguously distinguishing volcanically induced cooling, wetting, or drying from natural climate variability and (ii) attributing political instability, harvest failure, and famines solely to volcanic climatic impacts. This example shows that while the impacts of past volcanism must always be studied within the contemporary socio-economic contexts, it is also time to move past reductive framings and sometimes reactionary oppositional stances in which climate (and environment more broadly) either is or is not deemed an important contributor to major historical events.
- Article
(11303 KB) - Full-text XML
-
Supplement
(198 KB) - BibTeX
- EndNote
The Little Ice Age (or LIA) is a proposed climate interval during which glaciers expanded in mountain regions across the globe (Grove, 1988; Matthews and Briffa, 2005; Solomina et al., 2016), including the European Alps (Holzhauser et al., 2007; Nussbaumer et al., 2007), New Zealand (Lorrey et al., 2014), Alaska (Wiles et al., 1999), the Northern Rockies (Luckman, 2000), and the southern Andes (Masiokas et al., 2009). Glacier advance in the 17th century was favored by widespread but spatially and temporally heterogeneous cooling (Hegerl et al., 2011; Ljungqvist et al., 2019; Neukom et al., 2019) of mean annual temperatures on the order of ca. 0.5–1 ∘C relative to the reference period 1961–1990 (Mann et al., 2009; Christiansen and Ljungqvist, 2017).
In this context, the mid- and late-17th century represents an important phase of the LIA – and hence for paleoclimate research – due to the occurrence of very low solar activity from approximately 1621 to 1718 (Usoskin et al., 2014; Owens et al., 2017; Brehm et al., 2021). This phase, referred to as the Maunder Minimum (Eddy, 1976), coincides with a period of cooler temperatures (Luterbacher et al., 2004; Xoplaki et al., 2005) and strong decadal variability in summer and autumn precipitation as of the mid-17th century (Pauling et al., 2006) over Europe. Physicists have thus considered the cooling as compelling evidence of a large, direct solar influence on climate (Gray et al., 2010). More recent research argues that this temporal agreement alone does not establish physical causation between the solar Maunder Minimum and the climatic conditions during the late 17th century. Instead, multiple factors probably contributed to the cooling, including the drop in solar activity and changes in land use. The largest influence is, however, likely attributable to volcanic eruptions (Crowley, 2000; Owens et al., 2017; Slawinska and Robock, 2018) and their impact on the North Atlantic Oscillation (NAO; Booth et al., 2012; Zanchettin et al., 2013), the subpolar gyre (SPG; White et al., 2022), and sea ice growth over the Nordic seas (Lehner et al., 2013; Schleussner and Feulner, 2013).
The first few decades of the 17th century also experienced marked political instability and warfare. In Europe, the Thirty Years' War (1618–1648), the origins of which were connected to religious civil wars over German-speaking areas, turned quickly into a geopolitical war affecting most of continental Europe (Parker, 2006, 2013; Schmidt, 2018). By the turn of the 1630s and 1640s, the Holy Roman Empire, the Spanish Empire, Denmark–Norway, the Swedish Kingdom, France, and the Dutch Republic were involved in at least some warfare. Moreover, the period was characterized by civil wars including the Scottish Revolution (1637–1644), the Croquant (1637) and the Nu-pieds (1639) revolts in France, the Catalan Revolt (1640–1659), the Portuguese Revolution (1640, continuing to 1668 as the War of Restoration), the Irish Rebellion (1641–1642), and the English Civil War (1642–1651) (Parker, 2013). In China, the collapse of the Ming Dynasty (1368–1644), overthrown by a peasant uprising coinciding with severe drought and famine, is yet another superposition of climatic and political events (Zheng et al., 2014; Gao et al., 2021b).
This paper aims to shed light on the climatic and socio-economic events that shaped 17th-century societies across Europe, China, and Japan, thereby putting the eruption cluster between 1637 and 1646 into context and particularly the most substantial eruption(s) around 1640. This paper aims to underline how pre-existing weaknesses in socio-economic systems and unstable political conditions were key to transforming the climatic effects of volcanic eruptions into natural disasters. To this end, we here (i) summarize the current state of knowledge on the mid-17th-century eruptions from ice cores, (ii) identify – to the degree possible – the likely source volcanoes, (iii) reconstruct the climatic conditions that prevailed at the time of these eruptions over Northern Hemisphere (NH) landmasses and within Europe, and (iv) examine weather and climatic anomalies reported by chroniclers in Europe and Asia as well as the socio-political contexts in which these occurred. We conclude that the attribution of impacts from the mid-17th-century eruptions on contemporary societies remains difficult as these events – and their associated volcanic climatic impacts – occurred at a time of an already worsening climate, with pre-existing widespread and marked political instability as well as diminishing solar activity at the start of the Maunder Minimum, though this should not be automatically seen as precluding a role for volcanically induced climatic disturbance in the historical events of the period.
Ice cores from Greenland and Antarctic ice sheets contain information of Earth's past atmospheric composition, including concentrations of acid and/or sulfate emitted by past eruptions (Crowley and Unterman, 2013; Gao et al., 2008; Sigl et al., 2014; Toohey and Sigl, 2017b). Compared to other geological records (Brown et al., 2014), ice cores provide high dating accuracy and precision and offer virtually complete time series of historical explosive eruptions of the size of Pinatubo (1991) or larger (Sigl et al., 2015). The network of available ice cores is dense for the mid-17th century, and here we employ sulfate measurements dated according to the annual-layer-counted chronology WD2014 for the WAIS Divide (WDC) core (Antarctica) and the NS1-2011 chronology for the NEEM (2011S1) core (Greenland). We additionally use new high-resolution non-sea-salt sulfur (nssS) measurements corrected for sulfur contained in sea salt from Summit15 (Greenland; 72.6∘ N, 38.5∘ W, 3210 m a.s.l.), TUNU13 (Greenland; Sigl et al., 2015), and B40 (Antarctica; Sigl et al., 2015). Measurements were obtained by inductively coupled plasma mass spectrometry (ICPMS) conducted at the Desert Research Institute, Reno (USA), using the methodology described by Sigl et al. (2014). Monthly values shown in Fig. 1 were derived by assuming linear snowfall throughout the year.
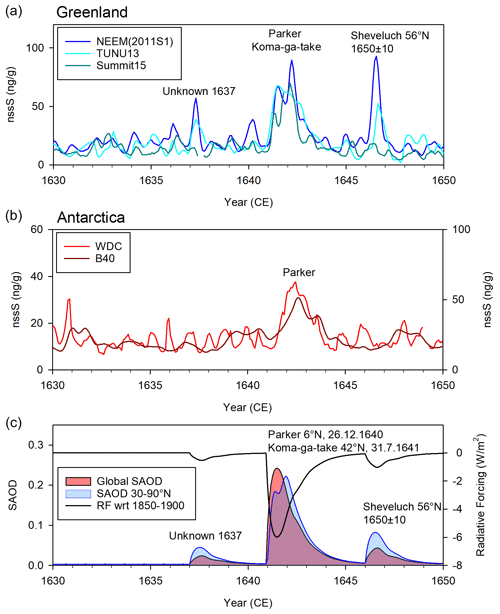
Figure 1Monthly resolution non-sea-salt sulfur records from ice cores in (a) Greenland and (b) Antarctica, with (c) stratospheric aerosol optical depth (SAOD) and estimated radiative forcing (RF) between 1630 and 1650 (data: Toohey and Sigl, 2017b).
The period 1630–1650 shows three distinct volcanic sulfur peaks in Greenland with concentrations peaking in 1637, 1641–1642, and 1646 (Fig. 1a). A smaller peak observed in NEEM (2011S1) in 1640 is not recorded in the two other ice cores. During the same period, only one unambiguous sulfur spike is recorded in Antarctica, observed in 1642 in both cores (Fig. 1b). The duration of elevated sulfate concentrations is 0.5 (1637), 2.3 (1641–1642), and 0.8 (1646) years in Greenland and 2.1 (1641–1642) years in Antarctica, respectively (Table 1), most likely reflecting differences in the aerosol life cycle of each eruption (Schmidt and Robock, 2015). For reference, the mean duration of volcanic sulfate deposition from the same ice core sites is 2.6 years for the eruptions of Tambora in 1815 (Brönnimann et al., 2019) and Samalas in 1257 (Lavigne et al., 2013; Guillet et al., 2017) and 1.7 years for the eruptions of Krakatau in 1883 (Verbeek, 1884; Winchester, 2003) and Pinatubo in 1991 (Parker et al., 1996).
Table 1Metadata for volcanic eruptions and radiative aerosol forcing in the mid-17th century and for selected large historical eruptions. VSSI: volcanic stratospheric sulfur injection.
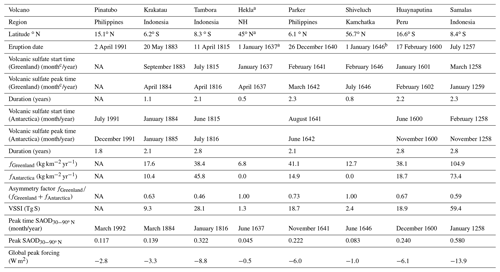
a Unknown event tentatively assigned to Hekla and assigned eruption date of 1 January 1637. b Unknown event tentatively assigned to Shiveluch (1651 ± 10) and assigned eruption date of 1 January 1646. c Nominal month assuming linear snowfall throughout the calendar year. NA: not available.
Time-integrated sulfate mass deposition rates (f) are 7 years (1637), 41 years (1641–1642), and 13 kg km−2 yr−1 (1646) over the Greenland ice core sites and 15 kg km−2 yr−1 (1641–1642) over the Antarctica ice core sites (Table 1; Toohey and Sigl, 2017b). An asymmetry ratio defined as of 0.73 indicates that the stratospheric sulfate burden after the 1641–1642 cluster was more strongly confined to NH. This contrasts with other notable stratospheric eruptions in the tropics that produced more symmetrical hemispheric sulfate burdens (e.g., Tambora, Indonesia, 1815: A=0.46; or Samalas, Indonesia, 1257: A=0.59). This suggests that even if we attribute the sulfate peak in 1641–1642 observed in both polar regions primarily to a tropical eruption, a NH eruption may also have contributed to increased sulfate concentrations in Greenland. However, this potential contribution cannot presently be quantified given the necessarily close timing of both such hypothesized eruptions and the different life cycle of atmospheric aerosol from tropical and extratropical eruptions (Toohey et al., 2013, 2019). As aerosol model simulations suggest limited transport of volcanic sulfate aerosols into the polar regions of the opposing hemisphere, the two smaller sulfate peaks observed only in the ice cores from Greenland in 1637 and 1646 can likely be attributed to eruptions in the mid to high NH latitudes.
The magnitude of the stratospheric sulfur injection for the eruptions producing the observed ice core sulfur signals has been estimated at 1.3 (1637), 18.7 (1641–1642), and 2.4 (1646) Tg S (Toohey and Sigl, 2017b). Taking the temporal clustering of the Parker and Komaga-take eruptions in 1640/41 as the best present candidates for the 1641/42 signal, they thus injected twice as much sulfur as Krakatau (6∘ S, Indonesia, 1883; 9.3 Tg S), almost as much as Huaynaputina (17∘ S, Peru, 1600; 18.9 Tg S), and roughly two-thirds of Tambora (1815; 28.1 Tg S) (Table 1). Forward modeling of atmospheric properties suggests strong increases in atmospheric opacity (i.e., stratospheric optical depth, SAOD) globally and particularly between 30 and 90∘ N latitude after the eruption cluster (Fig. 1c). Peak SAOD values occurred in June 1637 (0.05), December 1641 (0.22), and June 1646 (0.08). By comparison, the 1991 Pinatubo eruption had an SAOD of 0.12, while the 1815 Tambora eruption triggered an increase of up to 0.32. Following Hansen et al. (2005), we estimate that peak global radiative forcing (RF) due to the increased SAOD from these eruptions was −0.5 W m−2 (1637), −6.0 W m−2 (1641/42) and −1.0 W m−2 (1646).
The radiative forcing of the 1640/41 eruption(s) thus ranks among the largest of the past 1000 years, being about twice as large as Pinatubo (1991) or Krakatau (1883) and comparable to Huaynaputina in 1600 (Fig. 2). The eruption in 1646, though substantially smaller than the 1640/41 eruption(s), would still have been a significant eruption had it occurred in the 20th century. Its SAOD (Table 1) would have been surpassed by Pinatubo in 1991 and El Chichón (Mexico) in 1982 (Jungclaus et al., 2017) but by none of the other 20th-century eruptions.
Ice core sulfur signals do not allow discrimination between potential volcanic sources. Attribution only becomes possible if written documents confirm the coincident eruption of a given volcano (Lavigne et al., 2013; Verbeek, 1884) or (more securely) where tephra conserved in ice cores bears the geochemical fingerprints of a specific volcanic source (e.g., Jensen et al., 2014; McConnell et al., 2020). For none of the mid-17th-century eruptions has tephra been analyzed in the ice cores so far, such that the attribution of sources to the 1637, 1641–1642, and 1646 sulfur spikes remains provisional. The 1637 sulfur spike in Greenland ice cores can potentially be ascribed to an eruption of Hekla (63∘ N, Iceland), which erupted at VEI (Volcanic Explosivity Index) 3 on 8 May 1636. The eruption lasted more than a year, and the estimated 5×107 m3 of ejected tephra damaged pasture in northeastern Iceland, where it killed livestock (Thorarinsson, 1970). A spatially heterogenous sulfate distribution (note the absence of sulfate enrichment in the Summit15 ice core) is typical for eruptions in close proximity or upwind of the ice core sites. Sulfate deposition from distant eruptions with transport primarily occurring in the stratosphere is characterized by more uniform sulfate deposition over the ice sheets (Gao et al., 2007), as illustrated by the 1477 Veiðivötn eruption from Iceland (Abbott et al., 2021). The 1646 sulfur spike is often ascribed to Shiveluch volcano (56∘ N, Kamchatka Peninsula), which produced substantial pumice fall, voluminous ignimbrites, and pyroclastic flows in the mid-17th century. As the first Russian Cossacks arrived in Kamchatka only by the end of the 17th century, the eruption cannot be found in historical sources. Multiple trees were, however, killed by the pyroclastic flows and preserved in volcanic deposits. As the bark and some growth rings were burned by these flows, kill dates range between 1646 and 1649, with the latter likely corresponding to the absolute date (Solomina et al., 2008; Brown et al., 2014). The volcanic eruption(s) in 1640 and 1641 have been described in much greater detail in contemporary sources and ascribed to Komaga-take (Japan) and Mount Parker (Philippines). Both eruptions are discussed below.
3.1 Komaga-take
Komaga-take (; 42∘ N, 140∘ E; 1131 m a.s.l.), also
known as Hokkaidō Komaga-take (
), Oshima Komaga-take
(
), or Oshima Fuji (
), is an andesitic stratovolcano
located on Hokkaidō, Japan. According to the Global Volcanism Program
(GVP, 2013a), much of the truncated volcano is of Pleistocene age and the
breached crater (resulting from an edifice collapse in 1640) remains visible
(Yoshimoto and Ui, 1998). The collapse was presumably caused by a phreatic
eruption (Fig. 3a).
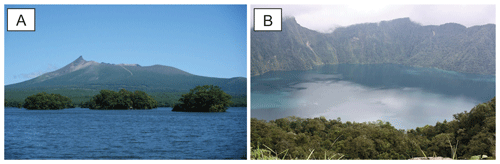
Figure 3(a) Komaga-take volcano (Hokkaido, Japan) (source:
Zelkova, CC BY-SA 3.0) as seen from Yakumo (in the NW); (b) Mount Parker (locally known as Mélébingóy), South
Cotabato, Philippines (Source: Noriah Jane Lambayan, CC BY-SA 4.0).
The yearly records of Matsumae, or Matsumae Nennen-Ki, report that on 31 July 1640, part of Komaga-take's summit collapsed to send pyroclastic flows and a huge debris avalanche (estimated at 0.25 km3) down its eastern slopes to produce a tsunami in Uchiura Bay (Katsui et al., 1975; Katsui and Chimoto, 1985; Yoshimoto et al., 2003), drowning at least 700 people in the present-day Toya and Usu region (Tsuji et al., 1994; Nishimura and Miyaji, 1995). Furious eruptive activity continued on 1–2 August 1640, so that contemporary sources report that “the day became dark like nighttime with little sunshine” (Katsui et al., 1975). While ashfall continued, the color of the cloud turned purple on 3 August in Matsumae. Activity weakened substantially thereafter and stopped after about 70 d (Katsui and Ishikawa, 1981). Ashfall deposits of the Komaga-take's 1640 eruption are 2 m thick next to the volcano and still reach 10 cm in Tsugaru region (northern Honshū), some 130 km south-southwest of the volcano (Yamada, 1958) for an estimated ejected volume of 3.5 km3 (Sasaki et al., 1970; Katsui et al., 1986). The 1640 Komaga-take eruption is thus one of the largest in Japan during historical times and deposited ash even in central Honshū, located >800 km to the south.
3.2 Mount Parker
Mount Parker (6∘ N, 125∘ E; 1824 m a.s.l.), locally known as Mélébingóy, is a densely vegetated, andesitic–dacitic stratovolcano near the southern tip of Mindanao Island (Philippines; GVP, 2013b). Its English name originates from 1934 when American general Frank Parker spotted the mountain and claimed its discovery during a flight he piloted (Davis, 1998). Mount Parker has a base diameter of 40 km; its summit is truncated by a 2.9 km wide caldera with steep walls rising 200–500 m above Lake Maughan (Lake Hólón locally; Fig. 3b).
The 1641 eruption was first described in western sources by the French naturalist Guillaume Le Gentil, who visited the region some 140 years after the cataclysm. In his two-volume work, A Voyage in the Indian Ocean (published in 1779 and 1781), he referred to a big eruption (erroneously dated to January 1640) that would have devastated large parts of the Philippines. Relying on contemporary Hispanic sources and geological investigations, Delfin et al. (1997) provided more insights into the sequence of the eruption. Mount Parker started erupting on 26 December 1640, and produced a major explosive event on 4 January 1641, resulting in the formation of the caldera as well as voluminous pyroclastic flows and lahars on its northern and eastern flanks (Delfin et al., 1997). The eruption produced pumice comparable in type to that described for the 1991 Pinatubo eruption (David et al., 1996).
While this eruption is known from contemporary Hispanic documents, an eyewitness report of Jesuit monk Raymundo Magisa written in 1641 has long been misattributed to Gunung Awu volcano in Indonesia (3∘ N, 125∘ E), ca. 300 km south of Mount Parker. The dating of nine charcoal pieces and oral traditions of local indigenous people finally allowed the attribution of the 1641 eruption described by Magisa to Mount Parker (Delfin et al., 1997). The same authors also translated Magisa's text testifying to the eruption:
“On … 4 January by 9 AM, the (supposed) artillery fire increased to such an extent that it was feared that the squadron (sent to investigate) might have run into some Dutch galleons. It lasted for about half an hour. People soon became convinced, however, that the noise originated from a volcano which had opened up, because by noon we saw a great darkness approaching from the south … By 1 PM we found ourselves in total night and at 2 PM in such profound darkness that we could not see our hands before our eyes. This darkness … lasted until 2 AM [5 January 1641] when a little moonlight appeared, to the great relief of Spaniards and Indios who had feared that they would be buried beneath the mass of ash which had started to fall on them at 2 PM. … At 10 AM the ships found themselves in such deep darkness and terrible blackness that they believed the Judgement Day to be at hand. It started raining so much rock, soil, and ash that the ships considered themselves in grave danger and had to light lanterns and quickly lighten the heavy load of soil and ash that was accumulating” (Delfin et al., 1997, p. 35).
The climatic conditions prevailing during the mid-17th century are quantified with a network of NH and European tree-ring records and complemented by excerpts from the vast corpus of historical records referring to harsh and hostile conditions in the late 1630s and early 1640s.
4.1 Northern Hemisphere summer temperatures
To quantify NH summer temperature (JJA) and identify possible volcanic cooling induced by mid-17th-century eruptions, we employed the NVOLC v2 reconstruction of Guillet et al. (2017, 2020). NVOLC v2 comprises 25 proxy records (13 tree-ring width (TRW) and 12 maximum latewood density (MXD) chronologies). A principal component regression (PCR) approach was applied to reconstruct NH JJA temperature anomalies (compared to the 1961–1990 mean). A bootstrap method was then combined with the PCR to estimate the skill of the reconstruction and to compute confidence intervals of reconstructed JJA temperatures. To gradually adjust to the changing number of records available back in time, the PCR was combined with a nested approach (Guillet et al., 2017). The NVOLC v2 reconstruction is consequently based on 23 subsets of tree-ring chronologies or nests, with the most replicated nest containing 25 chronologies spanning the period 1230–1972. For each nest, a principal component analysis was calculated on the proxy predictors, and the first n principal components (PCs) with eigenvalues >1 were retained as predictors to develop a multiple linear regression model. The regression models were all calibrated on JJA temperature obtained from the Berkeley Earth Surface Temperature (BEST; Cowtan and National Center for Atmospheric Research Staff, 2019) dataset over the period 1805–1972. For each nested regression model, a split calibration–verification procedure was repeated 1000 times using the bootstrap method to assess the robustness of the transfer function, and 1000 reconstructions were generated. The final reconstruction of each nest is the median of the 1000 realizations and is given with its 95 % bootstrap confidence interval. To quantify the reconstructed cooling within a context of climate variability at the time of the major eruptions under study, we filtered the NVOLC v2 series with a 31-year running mean (NVOLC_filt v2). For example, in the case of the year 1640, a background was calculated by averaging the window 1625–1639 and 1641–1655. The magnitude of the anomaly is then determined by subtracting this background from the temperature reconstructed in 1640.
Figure 4 illustrates the cooling induced by major volcanic eruptions of the 17th century. According to the NH JJA NVOLC v2 temperature reconstruction, the largest cooling induced by any eruption of the past 1500 years occurred after 1600 Huaynaputina in Peru (White et al., 2022) with a NH temperature reduction of −1.56 ∘C in 1601 relative to the 1961–1990 reference period, followed by a −1.48 ∘C reduction in 1816 (rank 2, Tambora), −1.42 ∘C in 1699 (rank 3, absent apparent volcanic activity), −1.40 ∘C in 536 (rank 4, unidentified source), −1.35 ∘C in 1453 (rank 5, unidentified source; Abbott et al., 2021), and −1.33 ∘C in 1109 (rank 6, the notable eruption cluster between 1108 and 1110, presumably Mount Asama, among others; Guillet et al., 2020). The assumedly largest volcanic eruption of the Common Era occurred in 1257 (Samalas; Lavigne et al., 2013; Guillet et al., 2017) and induced a cooling of −1.04 ∘C in 1259, ranking 16th in the NVOLC v2 reconstruction.
The years 1641 and 1643 stand out as the 13th and 17th coldest years of the last 1500 years with −1.13 and −1.02 ∘C anomalies, respectively. By contrast, cooling was less marked after the assumed 1636 Hekla (−0.15 ∘C; rank 628) and the 1646 Shiveluch (−0.36 ∘C; rank 348) eruptions.
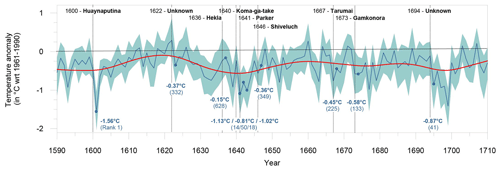
Figure 4Tree-ring-based (NVOLC v2; Guillet et al., 2020) Northern Hemisphere (NH; 40–90∘ N) summer (JJA) temperature reconstruction of the 17th century with volcanic eruptions and the associated cooling highlighted with blue dots. The rank values (in parentheses) indicate the amount of cooling induced by the eruption compared to all cooling events recorded over the past 1500 years. Volcanic cooling is calculated for all major 17th-century eruptions: 1600 Huaynaputina, 1636 Hekla, 1640/41 Komaga-take/Mount Parker, 1646 Shiveluch, and the 1694 unidentified eruption(s).
4.2 Reconstructed regional variability of summer cooling between 1638 and 1643
To estimate the regional variability of summer cooling induced by the mid-17th-century eruptions, we used the climate field reconstruction of extratropical NH JJA temperatures developed by Guillet et al. (2017, 2020) spanning the last 1500 years. The target field (predictand) used for the reconstruction is the BEST JJA gridded temperature dataset ( lat–long grid). The NH was divided into 11 regions based on the spatial distribution of the 25 chronologies and their correlations. Performance and temporal stability of reconstruction models was assessed for each grid point with a split-sample calibration and verification approach repeated 1000 times using the bootstrap method.
Figure 5 shows JJA-gridded reconstructions for some of the largest CE eruptions (Fig. 5a) and all years between 1638 and 1643 (Fig. 5b). As with other eruptions, we observe widespread yet heterogeneous NH summer cooling, above all in 1641. The most affected regions are the western US (up to −2 ∘C), Scandinavia (−1.5 ∘C), Siberia (–1 ∘C), Central Asia (−1 ∘C), and, to a lesser extent, western Europe (−0.4 ∘C). Whereas Central Asia, Scandinavia, and the western US experienced cooling until 1643, summer temperatures recovered more rapidly in the other regions.
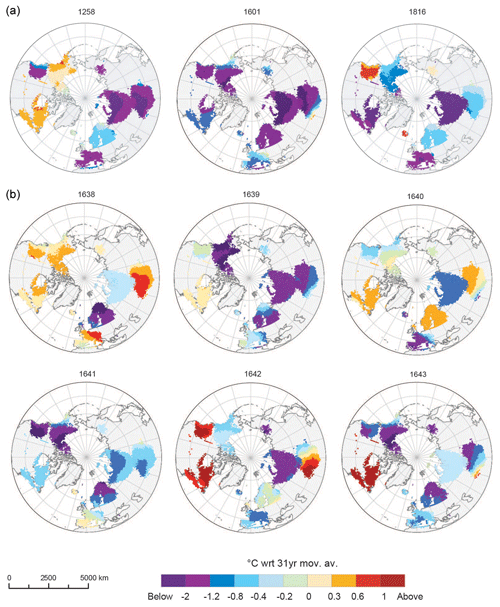
Figure 5Tree-ring reconstructions of NH extratropical land (40–90∘ N) summer temperature (July–August; JJA) anomalies (NVOLC v2; Guillet et al., 2020) after (a) major volcanic eruptions in 1257 (Samalas), 1600 (Huaynaputina), and 1815 (Tambora) as well as (b) between 1638 and 1643, representing the years before and after the 1640/41 Komaga-take and Mount Parker eruptions.
4.3 European summer temperatures between 1638 and 1643
The European summer temperature reconstruction uses 12 multi-centennial TRW (i.e., Forfjorddalen, French Alps, Tatra Mountains), MXD (Jämtland, Lauenen, Lötschental, Nscan, Pyrenees, Torneträsk, Tyrol), and stable oxygen isotope (Angoulême, Fontainebleau) chronologies covering western Europe and Scandinavia.
A PCR approach (Stoffel et al., 2015; Guillet et al., 2017) was applied to reconstruct summer temperature anomalies from these chronologies. Regression models were calibrated on JJA temperatures obtained from the E-OBS pre-1950 and v22.0e gridded datasets ( lat–long) over the period 1920–2010. For each regression model, a split calibration–verification procedure was repeated 1000 times using a bootstrap method to assess the robustness of the transfer function and to generate 1000 reconstructions. The final reconstructions for each grid point comprised the median of 1000 realizations and covered the period 1500–2010. For each reconstruction, we calculated coefficient of determination (R2 for the calibration period) and squared correlation coefficient (r2 for the verification period), RE (reduction of error), and CE (coefficient of efficiency) statistics. Anomalies are given with respect to a moving 31-year average calculated around the years given in Fig. 6. We compare our reconstruction using 12 TRW, MXD, and isotope chronologies (Fig. 6a) with the Luterbacher et al. (2004) dataset in Fig. 6b. For the domain and period of interest, the latter is based on ordinal-scale climate indices from Germany, Switzerland, and the Low Countries as well as TRW records from the Lofoten Islands for JJA temperatures, at a spatial resolution of lat–long.
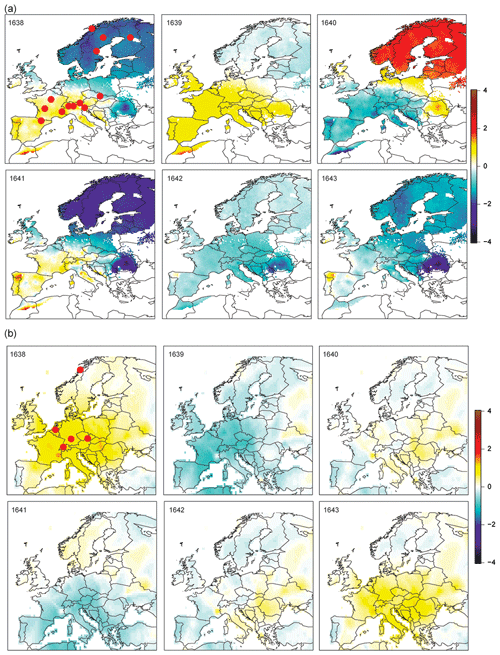
Figure 6Summer (JJA) temperature anomalies over Europe between 1638 and 1643 as derived from (a) a network of 12 tree-ring width, maximum latewood density, and stable isotope chronologies and (b) the Luterbacher et al. (2004) dataset. Temperatures are expressed as departures from summer (JJA) mean using a moving 31-year time window. Red dots denote the origin of the proxies used in either of the reconstructions.
Our gridded summer temperature reconstruction allows analyses of warm and cold spells that have affected Europe. Although not comparable to the cooling observed in Europe after the 1600 Huaynaputina (White et al., 2022; not shown) eruption (with −1.87 ∘C in 1601; rank 1), 1641 stands among the coldest summer seasons of the last 500 years (−1.19 ∘C; rank 16), with a cooling comparable to that observed after the Tambora eruption (−1.23 ∘C in 1816; rank 14). Notably, cooling is most obvious in Scandinavia where summer temperatures were more than −2 ∘C below average (compared to the 31-year moving average around the event) but much less marked in the Alpine and Mediterranean domains. The cooling becomes much more generalized across most of Europe in 1642 (−0.47 ∘C; rank 105) and in 1643 (−1.01 ∘C; rank 27). While it is tempting to attribute the strong, yet localized, cooling observed in Scandinavia in 1641 as well as the more widespread, but less marked, cooling in 1642 and 1643 to the eruptions of Komaga-take and Mount Parker in July 1640 and January 1641, respectively, at least some cooling may be the result of natural climate variability. Indeed, cold spells like those observed in the early 1640s and affecting most of Europe were frequent in the 17th century and occasionally occurred absent any known major volcanic activity, for instance in 1608, 1609, or 1633 (−1.57 ∘C; rank 4 for the latter).
Whereas both spatial reconstructions presented in Fig. 6 point to similarities in the overall tendency of temperatures in different years, they also point to regional departures and – on some occasions – even larger differences. Part of these differences can be explained by the much larger dataset used in Fig. 6a, but some differences may also be related to the choice of proxies, their spatial representativeness during particular years, the method applied with their the underlying assumptions, the length of the calibration period, and different choices in terms of instrumental data to derive the statistical connections.
In July 1641, several contemporary texts report “thick fog” in different regions of Germany. The Landgrave Hermann IV of Hesse (1607–1658) observed the weather around Rotenburg an der Fulda (Hesse) and in other locations on his frequent travels. On several occasions, he describes the almost continuous occurrence of fog after 17 July 1641 (HS1; appendix). The “thick fog” returns on 19–20 July and persists from 1 to 4 August (HS2). The weather is often described as “dull”, sometimes also reasonably pleasant and warm, “but not quite bright” (7 August 1641; HS3). A “thin fog” is described on multiple other occasions in August and early September 1641. On 13 and 15 August, the fog was so dense that the weather could not be observed (HS4). Interestingly, on 21 August, a “nicely warm” day was followed by “fog and hoarfrost” after midnight (HS5), a phenomenon observed again on 23 August 1641. On 6–7 September, Hermann described the weather as “dull and smoky” and used the word heerrauchig – a term that was often used in German to describe volcanic haze, even if observers were historically unaware of the origin of such dust veils (HS6, Demarée, 2014; Kleemann, 2019). From the evening of 7 September 1641, he continued to make observations from the town of Eisenach (Thuringia), where he witnessed fog on 7–8 and 12 September as well as hoarfrost on 8–9 September (HS7). On 18, 20, and 22 September, he noticed fog in Kassel and in Rotenburg an der Fulda (HS8). Fog was also observed in Paris, where Canon Jean de Toulouse of the Abbey of Saint-Victor stated that “from August 25, 1641, cold rains arrived, accompanied by unusual fogs and caused many diseases and a high mortality in the city until March 1642” (Garnier, 2010).
Hermann IV of Hesse's multiple notices of heerrauch are particularly noteworthy. These are found in March and September 1641 (as noted earlier), recurring in April, May, and July 1642. We may question whether all such observations are linked to a volcanic dust veil or should be ascribed to other phenomena such as forest or turf fires. During summer 1641, however, it seems unlikely that forest or turf fires made a significant contribution to the fog and haze as the season had been described as wet and cold, with conditions rather unfavorable for ignition.
Independent evidence for the presence of volcanic aerosols in the stratosphere comes from a dark lunar eclipse at 01:52 UTC on 25 April 1642. The luminosity and brightness of total lunar eclipses are a reliable indicator of stratospheric turbidity (Stothers, 2004, 2005; Guillet et al., 2020). While copper-red lunar eclipses generally point to a clear stratosphere, the eclipsed Moon tends to appear darker or even to disappear entirely if the stratosphere contains sufficient volcanic aerosols. Dark total lunar eclipses were thus observed following the 1257 Samalas, the 1600 Huaynaputina, the 1884 Krakatau, the 1912 Katmai–Novarupta, the 1963 Agung, the 1982 El Chichón, and the 1991 Pinatubo eruptions (Guillet et al., 2020). For our period, the astronomer Johannes Helvetius (1611–1687) reports, in his Selenographia published in 1647, that the location of the moon could not be ascertained during the lunar eclipse of 25 April 1642, even with the aid of the telescope, although the air was sufficiently pure to discern the stars (Helvetius, 1647; HS9). This account contrasts starkly with the usual records of total lunar eclipses depicting bloodred eclipsed moons and suggests that a significant volume of volcanic aerosols still remained in the stratosphere in April 1642, i.e., 15 months after the eruption of Mount Parker.
Weather anomalies were widespread in the late 1630s and the early 1640s, as described in diverse contemporary sources. The following subsections present evidence for unseasonal, sometimes even severe, weather conditions in western, central, and northern Europe as well as in China and Japan. Given the vast number of historical documents surviving from the mid-17th century, we can provide only a glimpse of what exists regarding weather data for parts of Europe and eastern Asia at the time of the Komaga-take and Mount Parker eruptions and in the years following.
6.1 Western and central Europe
Western Europe of the early to mid-17th century was dominated by the Holy Roman Empire, which roughly included the territory of modern-day Austria, Belgium, Czechia, Denmark, Germany, northern Italy, Luxembourg, westernmost Poland, the Netherlands, Slovenia, and Switzerland. The Empire was both religiously and politically fragmented and was riven by the Thirty Years' War (1618–1648), occurring predominantly within its own territory. The war involved multiple European powers with the combatants often divided between the different religious denominations – Catholicism and Protestantism – though the centrality of the role of religion can be questioned (e.g., Wilson, 2008) and related exceptions existed, not least Catholic France, which fought on the “Protestant” side for strategic reasons. What is certain is that the spread of diseases, the rise of poverty, lack of resources, quartering of soldiers, general violence, and looting also cost many lives and created immeasurable hardships for populations within the Holy Roman Empire over these 3 decades. In 1620, the Holy Roman Empire had about 16 million inhabitants; around 1650 it had only 10 million (Gotthard, 2016), though there are considerable difficulties in accurately estimating population loss for this period (see the review by Wilson (2009), who states that total deaths in the empire may have reached 8 million). The war was in its final phase in the 1640s and, having already been suffering from the prolonged conflict, disease and hunger were likely enhanced by the severe weather of the early 1640s.
Contemporary chroniclers detail harsh weather in the early 1640s and numerous eyewitness reports underline the cold and wet conditions prevailing across the region. Both spring and summer 1640 are reported as being wet and cold, with many rivers flooding. For the territory of modern-day Switzerland, March temperatures sank to such a low that smaller water bodies were covered with ice and snow on the Swiss Plateau into April (HS10). Grain harvest on the Swiss Plateau was also poor (Pfister, 1984). The following winter 1640/41 was long and severe, followed by a rather wet and cool spring. In the area of present Switzerland, the winter 1640/41 saw heavy snow. On the Swiss plateau, snowfall occurred in late March and April (HS11), followed by a late frost in May (HS12). Summer 1641 was cold across Europe, ice formed, for instance, several times in southern Germany (Middle Franconia), and especially on 25 July 1641 (4 August 1641) (HS13, 14), and summer was reported as anything but sultry in Europe even during the dog days. In Krupka (today's Czechia), frost was noted on 10 August 1641 – and cucumbers and cabbages froze (Brázdil et al., 2004).
At Frankfurt an der Oder, the harvest was affected by incessantly rainy, cold weather, and thunderstorms (HS15), and frost in mid-September 1641 destroyed wine in southern Germany (HS16): “The wine of 1641 was sour …, grapes did not grow at all during the dog days, [and] before harvest they froze on the vines” (HS17). Various independent sources confirm the presence of strong hoarfrost around mid-September 1641 in Germany. In the area of present Switzerland, the harvests were poor and late as well (Pfister, 1984).
In May 1642, much of present-day Germany saw a cold spell: in Bavaria, “the coldest winds and hoarfrost” continued until mid-May, damaging grain and fruit trees greatly (HS18-19). Late snowfall and hard frosts are reported for May 1642 in Krupka, causing damage to the vineyards (Brázdil et al., 2004). hoarfrost and snow are again reported for several days in southwestern Germany (HS20) and Krupka (Brázdil et al., 2004) in June 1642. By contrast, a report from Diez (Lahn) in today's Rhineland-Palatinate noted that the summer 1642 was calm and the people in Diez harvested exceptionally good crops (HS21).
The Baden wine chronicles allow a comparison of the quality of the wines produced each year during the 17th century. The 1630s and 1640s clearly appear to be very harmful for wines. Of the 1630s, only the years 1631 and 1638 produced “good” wine, while the others produced “bad” or “sour” wine. Between 1640 and 1650, just 4 years (i.e., 1644–1647) provided “good” wine. For 1641, the Baden chroniclers indicate that the vine suffered late frost in May and then early frost in September (Garnier, 2010).
In neighboring France, the grape harvest between 1640 and 1643 began a full month later than usual and wheat prices surged from 12 to 20 pounds (per setier, that is about 7 L) between 1641 and 1642 (Baulant, 1968), indicating poor cereal harvests, especially also in eastern France. A source from Dijon described summer 1640 as chilly, resulting in a grape harvest that was comparably late. Poor temperatures also resulted in high grain prices on the Île de France. The year of 1641 saw considerable precipitation during winter and rather low summer temperatures. In 1642, the grape harvest was again late in Dijon and very late around Paris, while grain prices increased rapidly in the Île de France, probably the result of cold conditions prevailing in 1642 (Parker, 2013).
Grape harvest dates in Besançon (France) are intermittent in the 1630s and 1640s, only available for 1631, 1633, 1635, 1638–1642, 1646, and 1649. In these years, harvests occurred late between 1 and 15 October (Garnier et al., 2011). Years without harvest dates are not necessarily explained by meteorological factors. Most correspond with the arrival of the Swedish and French armies in Franche-Comté (1632, 1641, 1644, 1639, 1644) or can be related to the quartering of Swiss mercenaries (1634), damage to vines by “German soldiers” of the Duke of Lorraine (1637, 1647), and the plague (1630 and 1635). Only 3 years without harvest (1636, 1648, and 1650) are attributed directly to meteorological conditions by the city clerks: more exactly to frequent rainfall episodes between June and October. Abnormally rainy conditions were obviously sufficient to justify pro serenitate processions during which people prayed for cessation of the rain (Garnier, 2010).
Paris has excellent climate observations between 1630 and 1640 in municipal acts. Parisian aldermen (échevins) met almost weekly to deliberate on city affairs. Among them, climatic conditions, more particularly extreme events, were systematically discussed. This is easy to understand since droughts, floods, or even freezing of the Seine had an almost immediate impact (in 1 or 2 d) on prices in Parisian markets. Indeed, until the mid-19th century and the arrival of the railroad, bulk goods such as wheat and wood used by city dwellers for heating and cooking were mainly transported by waterways (Garnier, 2015). The municipal acts also provide other indicators for droughts, mentioning the drying up of the water sources and public fountains supplying the main hospital and conditions that prevented hydraulic mills from making flour. Between 1630 and 1650, Paris thus suffered five very severe droughts (some long like those of 1631–1632 and 1646–1647), but the drought of 1639 was undoubtedly the most dramatic of the 17th century (Garnier, 2019). Between 1630 and 1650, freezing of the Seine affected Paris six times (1635, 1636, 1637, 1644, 1645, and 1646). In the latter case, it is likely that the freezing was favored by the drought which preceded it in summer and the autumn, so that when the cold arrived, low water levels facilitated ice formation that lasted nearly 3 weeks.
6.2 Northern Europe
In the 17th century, most of modern-day Sweden, Finland, Estonia, and northern Latvia belonged to the Swedish Realm, a “great power” of its time. Like in the Holy Roman Empire, contemporary documents point to severe weather and resulting agricultural hardship in the early 1640s. Estonian and Livonian sources report unusual heat and drought in summer 1640 to a degree that grain crops were damaged (Tarand et al., 2013), whereas the following two summers were cold and rainy (Soom, 1940). Grain ripening was badly delayed and the first autumn frosts in early September 1641 destroyed a great part of the harvest. The winters 1640–1641 and 1641–1642 were notably cold (Tarand and Nordli, 2001). Crop failures with consequent food shortage troubled Estonia in 1641 and 1642 but did not likely escalate into an acute subsistence crisis (Seppel, 2008). In the territory of present-day Sweden, bad crop failure was reported in 1641, especially in northern Sweden (Leijonhufvud, 2001). In Stockholm, at the heart of the realm, grain prices were 13 % to 20 % higher in 1641–1643 than the 1630s average (Edvinsson and Söderberg, 2010). Southern Sweden suffered a “violent fever or burning disease epidemic [likely typhoid fever and typhus, which are hunger-related diseases], which killed both rich and poor” especially in late spring 1642 (HS22).
Within the Swedish Realm, the area of modern-day Finland was likely the most affected by the weather anomalies of the 1640s. Already in 1632–1635 the region suffered from local poor harvests, and the year 1633 witnessed a country-wide crop failure (Johanson, 1924). In August 1638, a week-long frost killed the crops and allegedly “hand-deep” snow covered the ground (Johanson, 1924). Like in Estonia, cold conditions in summer 1641 delayed the ripening of grains, and frosts in early September killed the grain (Mårtensson, 1952). Grain tithes from western Finland indicate that the 1641 harvest was half that of normal (Huhtamaa and Helama, 2017); harvests remained poor in the following year, with yields only reaching approximately two-thirds of the mean (Huhtamaa and Helama, 2017). Unlike in Sweden and Estonia, the crop failure in 1641 triggered severe hunger in Finland. The economic consequences of these harvest losses can be seen in many regions, with every fifth or fourth farmstead marked as “deserted” in the administrative records (Huhtamaa et al., 2021). The high percentage and spatial extent of abandoned and acutely impoverished farmsteads in the mid-1640s point to a severe agricultural crisis, similar to the early 1600s when the eruption of Huaynaputina in 1600 (White et al., 2022) triggered harvest failures, famine and farm desertion (Huhtamaa et al., 2021). Whereas in the Swedish Realm the year 1640 was hot and dry, unusual cold and constant rain ruined the hay in Iceland in 1640, such that farmers resorted to dried fish as cattle fodder (Ogilvie, 1984).
In Ireland, winter 1641–1642 was particularly severe. Numerous contemporary documents describe the severity of cold. Particularly important are the approximately 8000 surviving statements of mainly Protestant interviewees (or “deponents”) recounting their experiences during the 1641 Rebellion, as attested to British government authorities, beginning with the work of the Commission for Distressed Subjects in December 1641. In these documents we hear survivors (often widows from Ulster and Leinster) describing “cold snowy weather” and “the extremity of the winter” (HS23). This unusual cold exacerbated the consequences of the rebelling Gaelic and “Old English” Catholics, who killed or turned Protestant residents out of their residences, in addition to their stripping and forced expulsion (Fig. 7). Thus, Katherin Cooke of Armagh recalled “such frost [and] snow that the deponent[']s children and divers [many] other children … present at that battle would[,] where they saw the warm blood of any fall on the ground[,] thread therein with their bare feet to keep them from freezing and starving such was the extremity of the weather” (HS24). Another deponent recalled: “Whereupon the deponent and his wife and 5 small children going away were stripped of all their clothes … one poor daughter of his seeing him … grieve for their general miseries in way of comforting said she was not cold nor would [she] cry although presently after … she died by that cold and want: and the first night this deponent and his wife creeping for shelter into a poor crate were glad to lie upon their children to keep in them heat and save them alive” (HS25). Other sources also provide visual depictions (often with propagandistic intent), such as that from James Cranford's, The Teares of Ireland (1642; Fig. 7), the caption from which reads: “English Protestantes striped naked and turned into the mountaines in the frost, and snowe, whereof many hundreds are perished to death, and many liynge dead in diches and savages [mainly Irish Catholics] upbraided them saynge now are ye wilde Irish as well as wee”. These conditions have, to date, been associated with explosive volcanism by Ludlow et al. (2013) and more specifically the eruption cluster starting in 1636 by Ludlow and Crampsie (2018, 2019).
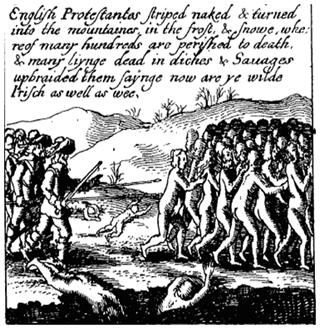
Figure 7Illustration (perhaps by Bohemian artist Wenceslaus Hollar) of alleged atrocities perpetrated by Irish Catholics during the winter of 1641/42; one of multiple such images accompanying the text in Presbyterian clergyman James Cranford's propagandistic The Teares of Ireland (London, 1642). We thank Jane Ohlmeyer for highlighting this image.
6.3 China and Japan
The Compendium of Chinese Meteorological Records of the Last 3000 Years (hereafter the Compendium; Zhang, 2004) is the key reference for past weather and climate anomalies for the territory of present-day China. The Compendium is compiled from 7930 volumes of the state annals and local chronicles, supplemented by representative personal diaries. For the Ming and Qing Dynasties (1368–1911), records can be considered precise at the county level and reported place names have been linked to the corresponding modern county. Here, we adapt a simple sorting and counting approach to digitize documentary records (Gao et al., 2017) and to quantify the occurrence of droughts or other disastrous events in each county Gao et al., 2021a).
At the time of the 1640s eruptions, China was suffering one of the most disastrous droughts in its known history, called the Chongzhen Great Drought (Chen et al., 2020). Extraordinarily dry conditions (Fig. 8) resulted in severe societal and economic consequences during the final years of the Ming Dynasty (1638–1644), perhaps ultimately contributing to its collapse. Drought started to emerge in the provinces of Shandong, Henan, and Shaanxi along the Yellow River Basin in 1638, with 91 counties reporting drought. Reports rose to 109 counties in 1639 and 303 in 1640, almost 6 times the average annual drought count during the 17th century. Indeed, 1640 may have been the single driest year in the region since at least 1470 (Zhang and Lin, 1992). The drought expanded from the Yellow and Huai River valleys to the middle and low reaches of the Yangtze River in 1641 (Fig. 9). Local sources report that from the Huai Valley to the Northern Metropolitan Region, “all the bark had been stripped from the trees and people [even] dug up corpses for food”. In the early months of 1641, unusually heavy snowfall was then recorded in southeastern China, while conditions remained very dry with infestations of locusts in northern China from where they propagated rapidly to the Yangtze River valley to form a large-scale locust plague (Song, 1992; Liu et al., 2018). The drought and locust plague resulted in an estimated reduction of 20 %–50 % in per capita grain production (Zheng et al., 2014), triggering a sharp increase in rice prices that intensified widespread famine (Fig. 8).
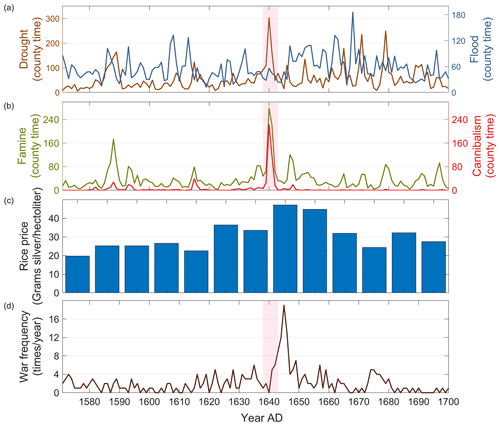
Figure 8Chinese weather anomalies, food shortages and warfare, 1571 to 1700: (a) drought and flood data as well as incidents of (b) famine and cannibalism as reported in the Compendium of Chinese Meteorological Records of the Last 3000 Years (Zhang, 2004); (c) rice prices (Liu, 2015) underline the food scarcity of the early 1640s, a potential factor in favoring (d) elevated war frequencies during the period (Zhang et al., 2006).
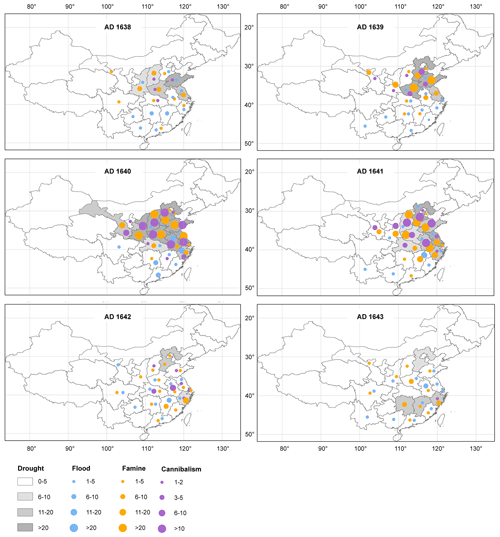
Figure 9Weather anomalies and social disasters, 1638 to 1643, in China (Gao et al., 2017). Background shading indicates recorded drought event numbers each year per province (the darker the shade, the more severe the drought damage). Blue circles represent abnormal flood event frequencies each year per province (larger circles equal higher frequencies). Pink and red circles present the number of recorded famine and cannibalism events, respectively.
The severity of the drought was such that part of the Grand Canal in Shandong Province dried out completely, an incident without documented parallels over the past 3000 years. Water flow of the Yellow and Yangtze Rivers as well as lake levels in Zhejiang, Shaanxi, Shandong, Henan, Shanxi, and Hebei provinces dropped and eventually dried up in 1640–1641 (Zhang, 2004). Shen et al. (2007) have suggested that the intensity of the Chongzhen Great Drought (1638–16.44) was comparable to that of 1997, in which about 700 km of the lower Yellow River dried up, stopping outflow to the Yellow Sea for more than 300 d. The Chongzhen Great Drought was likely the outcome of natural climate variability potentially intensified by the Komaga-take and Mount Parker eruptions. Chen et al. (2020) ascribe this intensification to a volcanically induced decrease in land–sea thermal contrast and a negative soil moisture response combined with a weakening and eastward retreat of the West Pacific Subtropical High.
In many provinces, purported incidents of cannibalism occurred, and a widespread epidemic was likely triggered or at least compounded by malnutrition and weakened immune systems. By 1641, 29 counties within Shandong Province reported this pestilence. Conditions are described in the Gazettes of Tangye County: “No rain again in spring, a bucket [a unit used to measure grain, about 7.5 kg in the Ming Dynasty] of rice worth more than a thousand QIAN [a unit used to measure money, about 3.125 g in copper], cannibalism among family members, corpses buried for weeks were dug out for food. Pestilence raged in summer, no household escaped; … eight to nine out of ten people died of pestilence, hunger and robbery … it was indeed a situation that has never been seen or heard of” (Zhang, 2004, vol. 2, p. 1612).
Between 1641 and 1646, rebellion and warfare reached historical highs (Zhang et al., 2006; Zheng et al., 2014). The year 1645 ranks first in war frequency for the period 850–1911 (Fig. 8), with 16 wars in central China and 6 rebellion wars elsewhere in the country. With some parallels to Ireland (Ludlow and Crampsie, 2019), the counties in which uprisings occurred were most frequently those where extreme weather was most severe, suggesting that regional drought severity played a critical role in fueling or worsening peasant uprisings (Zheng et al., 2014).
Japan was likewise affected by a famine already underway at the time of the
Komaga-take and Mount Parker eruptions in 1640 and 1641. The so-called Great Kan'ei Famine ( or Kan'ei kikin) started in 1640 and lasted until 1643, with
up to 100 000 people and innumerous livestock dying from starvation and
disease (Yamaguchi and Sasaki, 1971; Asao, 1975; Nagakura, 1982). The Kan'ei
kikin was the first major famine to occur in Japan after the rapid urban and
demographic growth of the late-16th and early-17th centuries that made many more Japanese dependent upon others for their food supply (Atwell,
1986). The eruption of Komaga-take on 31 July 1640 must thus be
ascribed a compounding role to an already imminent crisis: heavy ashfall
resulted in plant poisoning near the volcano and triggered local crop
failure over 2 years. In 1641, the situation worsened as
incessant rainfall and unusual cold propelled another poor harvest in the
northeast (Yamamoto, 1989; Atwell, 2001). Winter 1641–1642 was cold
as well and summer 1642 was cool, diminishing harvests once again
in northeastern Japan, whereas floods and droughts destroyed harvests
elsewhere in the country (Atwell, 2001).
In 1642, “the corpses of those who had starved to death filled the streets while the peasants, artisans, and merchants who begged for food were numerous. None of these people had any clothes [and could only] wrap straw and other matting around their bodies for protection” (Kanei nikki zōho in Asao, 1975). By June 1642, starving peasants started to abandon their lots to the degree that the governor ordered the abolition of tobacco plantations and strongly restricted alcohol production to favor food crops. Regardless, death tolls increased in winter 1642–1643 such that farmers performed infanticide of children below 7 years (Drixler, 2013) and lent out older children, often to pimps, in Fukushima Prefecture. The famine ceased only later in 1643 when crop yields rebounded.
Volcanic eruptions have been demonstrated to influence climate by ejecting gases and solid particles into the atmosphere (Robock, 2000; Timmreck, 2012; Sigl et al., 2015), where sulfur-containing gases are converted into aerosol particles (Zanchettin et al., 2012). These volcanic aerosol particles influence Earth's radiative balance by (i) scattering incoming solar radiation back to space, a process that will cool the troposphere and the surface, and by (ii) absorbing long-wave solar and terrestrial radiation, leading to a local stratospheric warming (Fischer et al., 2007; Christiansen, 2008; DallaSanta et al., 2019). At the same time, volcanic eruptions are also known to affect global precipitation patterns (Iles et al., 2013; Rao et al., 2017; Zuo et al., 2019), including a weakening of the East African Monsoon (Khodri et al., 2017; Manning et al., 2017; Zambri et al., 2017) and/or the East Asian Monsoon (Zambri et al., 2017; Dogar and Sato, 2019).
7.1 Worsening climatic conditions and advancing glaciers in the European Alps
The early decades of the 17th century mark the start of prolonged limited sunspot activity known as the Maunder Minimum (1645–1715). This period also marks a phase during which climatic conditions were worsening, with colder conditions also translating into advancing glaciers. In the Alps, contemporary sources report the disappearance of fields, farmsteads and entire villages as various glaciers reached their furthest extent in historical times (Nussbaumer and Zumbühl, 2012): the Mer de Glace (Mont Blanc, France), for instance, had one of its Little Ice Age maxima in 1644 after advancing by more than 200 m between 1641 and 1644. This points to a sustained, positive mass balance of the glacier, implying a multi-year succession of cold and wet conditions. By contrast, the Lower Grindelwald Glacier (Switzerland) reached its Little Ice Age maximum at some time between 1600–1606 and 1636–1645, with a second peak in 1855/6. The Upper Grindelwald Glacier had its largest extension between 1593 and 1630. Indeed, various glaciers across the European Alps reached valley floors at the beginning or during the first decades of the 17th century (Sigl et al., 2018).
In 1642, officials of the village of Chamonix (France) spoke of the daily progression of the glacier and evoked the avalanches of snow and ice that continued to carry away houses and livestock. The same was true for the Argentière glacier (France), which threatened to consume the church of the same name. The Glacier des Bois (France) exhibited the most impressive advance and in 1644 threatened to block and transform the Chamonix valley itself into a lake. Alpine mountain communities coped as best they could. Believing glaciers to be evil, they invoked divine protection, at first resorting to rogations to implore God's help. Faced with the ineffectiveness of this strategy, they then requested the intervention of the Bishop of Geneva, who in 1644 led a procession there. Thereafter, the ice slowly receded (Le Roy Ladurie, 2004).
7.2 Political instability, religious disputes, warfare, and abandonment of farms
The early decades of the 17th century were characterized by major warfare and widespread religious and dynastic disputes. The most widely known is the above-described Thirty Years' War (1618–1648), consisting of a series of battles centered in and around present-day Germany, resulting in one of the deadliest wars of Europe. While at first restricted to battles among Catholic and Protestant states of the Holy Roman Empire, fighting became part of a wider European struggle after 1635, with the Swedish Realm, Dutch Republic, and France on the one side and Spain and the Austrian Habsburgs (including large parts of the Holy Roman Empire) on the other. Estimates of military and civilian deaths range from 4.5 to 12 million, of which only 450 000 died in the fighting (Outram, 2002; Parker, 2008; Wilson, 2009). The vast majority, however, died from disease, starvation, or violence against civilians. Some sources suggest a mortality of up to 60 % of the population in certain areas of present-day Germany (Outram, 2002), while in the Palatinate and Württemberg, losses are estimated at between 70 % and 90 %, and in Alsace they average 60 % (Outram, 2002). These deaths must be considered the result of combined severe weather conditions (induced or at least often compounded by volcanism), epidemics, military violence, and famines. It is difficult to determine which was the most decisive factor, particularly given their likely interdependencies, but the scale of the combined impact is less in doubt. Evidence suggests that mortal sickness in military camps and peasant communities far exceeded death in action, perhaps in the proportion of 5 to 1 (Outram, 2002).
The entry of France into “open war” in 1635 brought a considerable increase in taxation, contributing to revolts almost every year for 10 years, mainly in the south and southwest of France. The movements of troops served as a vector for the plague which spread widely. Famous for his hostility to d'Artagnan and his musketeers in the novel by Alexandre Dumas, Cardinal Richelieu is better known to historians for having created in 1638 the heaviest regular tax, in the form of the “wintering place” (quartiers d'hiver) for troops. This obliged subjects of the King of France to provide food and shelter for soldiers during winter. Requisitions for food and equipment such as mills, ovens, and means of transport were also often carried out in a context of pillage and brutality. A further cause of discontent was the tailles, comprising all direct taxes. These, moreover, were paid almost entirely by the peasants, and the Thirty Years' War was thereby waged heavily at the expense of peasant income in France. In 1648, the share of tailles in the revenue of the royal state approached 62 %. Popular riots among peasants and modest city dwellers thus appear to be a response to fiscal and military aggression of absolutist authorities, and the socio-economic “efficacy” of shocks to agricultural production arising from inclement weather in this period and region must be understood as operating against this background.
The multi-faceted associations among climate, warfare and famine are exemplified by the case of Finland, being part of the Swedish Realm in the early 17th century. The Swedish Realm intervened in the Thirty Years' War in 1630 and from the late 1630s its army consisted increasingly of Swedish and Finnish conscripts as infantry was raised by conscripting every 10th man among the adult male peasant population (Jespersen, 2016). The Realm's constant need for soldiers increasingly impacted agricultural production, as call-ups were annual during wartime. In the case of Finland, conscriptions were particularly heavy with at least 13 000 men recruited between 1638 and 1645 (Lappalainen, 1987). This number is considerable as the area held only an estimated 27 500 peasant households at this time. Consequently, nearly every second farm was missing an adult male from its agricultural workforce. Furthermore, the Swedish recruitment system created an additional financial burden, because to avoid being shipped to the European battlefields, peasants started to hire men to take their place in the conscriptions (Villstrand, 2000). Yet even if hired men, adult sons, or farmhands were recruited preferentially, heads of farmsteads were increasingly conscripted with time and as the number of available men decreased. By 1643, nearly 60 % of all recruited men from southern and central Finland were the masters of their farmsteads (Lång, 2003). The resulting labor shortfall undoubtedly affected grain production quantity and quality. Even if battles were fought far from Finland, livelihoods were rendered more vulnerable to environmental hazards, like the extreme cold of 1641. Thus, the synergistic interaction of warfare and extreme cold was key to the early 1640s agricultural crisis in Finland (Huhtamaa et al., 2021), and it is arguably a matter of semantics (and/or disciplinary norms) as to whether both are considered “causal” or “contextual” in this sense. The poor weather also diminished yields in Estonia and the main agricultural areas in Sweden, but being located further south than Finland, they were less affected by the unseasonal summer weather (Edvinsson et al., 2009), while Estonia (and men from other newly gained territories) also escaped the onerous conscription experienced by Finnish peasants.
In Ireland, one of the most infamous rebellions against English rule erupted in October 1641 with Irish Catholics trying (unsuccessfully) to seize control of the English administration in Dublin but killing and displacing many Scots and English Protestant settlers in what evolved into the Irish Confederate Wars, 1641–1653 (also known as the Eleven Years' War, in Irish Cogadh na hAon-déag mBliana) (Ó Siochrú, 1999). This wider conflict was perhaps the most devastating in Irish history (Fig. 10), with direct deaths in conflict (including by infighting) considerably augmented by hunger, disease (including bubonic plague) and weather-related exposure (Dorney, 2014). Neither the total population of Ireland nor exact numbers of deaths between 1641 and 1653 are precisely known, but a finding of 15 %–20 % mortality countrywide has been credibly offered (Lenihan, 1997). The initial outbreak of rebellion in October 1641 was preceded by poor weather (plausibly compounded by the sequence of eruptions starting with Hekla in 1636), with consecutive years of poor harvests from 1639 to 1641 (Ohlmeyer, 1998; Gillespie, 1989). These climatic–agricultural pressures can, with care, be posited as one trigger for the rebellion's onset in 1641 (Ludlow and Crampsie, 2018), though a full understanding of the role of climate must ultimately grapple with its interdependencies with other economic, political, class, cultural, and religious factors.
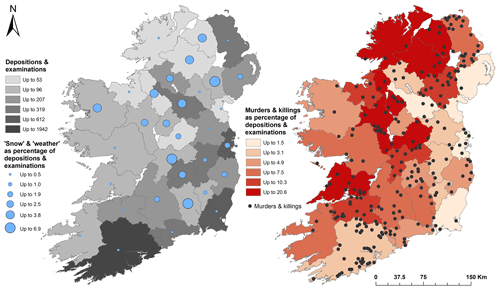
Figure 10Geography of reported severe weather and murders and killings during the 1641 Rebellion in Ireland. More specifically, the left panel shows total Depositions and related documents per county (gray shading) as listed on https://1641.tcd.ie/ (last access: 10 October 2015). The percentage of these referencing “snow” and severe “weather” are shown in graduated blue dots and broadly follow a climatologically credible pattern, with greater percentages in more northerly counties as well as the midlands, which lack the moderating influence of the Atlantic on temperatures. Right panel: murders and killings reported in the Depositions (taken from Smyth, 2013) are shown as black dots and expressed as a percentage of total Depositions per county (red shading). Gradation into intervals in both maps employs Jenks natural breaks. Figure redrawn after Ludlow and Crampsie (2019).
Any hypotheses offered must also pay careful attention to the respective timing of events. As an example, consider the impacts of the 1641 Rebellion as described in great detail in documents known as the Depositions (https://1641.tcd.ie, last access: 10 October 2015), comprising more than 8000 (mainly Protestant) witness statements that make multiple dramatic references to extreme cold and severe weather in the 1641/42 winter (Sect. 6.2). With the rebellion beginning in October, these specific attestations will, however, refer to the months after the initial onset of rebellion and cannot therefore be strictly credited with a role as an initial trigger (though they may represent an evolution of inclement weather responsible for the aforementioned poor harvests). Nonetheless, comparing the geography of reported killings in the Depositions (Smyth, 2013) with these references to (post-rebellion-onset) extreme weather per Irish county, whilst (crucially) controlling for variable witness numbers per county, reveals a statistically significant positive correlation (Spearman ρ=0.596, p=0.003, n=32: Ludlow and Crampsie, 2019). One credible part-explanation is that regional variance in the severity of weather also influenced the scale and severity of violence once it was underway and particularly as the rebellion spread among Catholic underclasses and those disposed of lands that had become increasingly marginalized and vulnerable under the preceding decades of British rule. Such participants may have been more likely to engage in violence (and in violence of greater severity) if they were more severely affected by the weather conditions attested to in the Depositions.
Even if true, this observation tells only part of the story, though it is one largely absent from understandings of the rebellion until recently. The geography of violence during the rebellion must certainly reflect many additional factors, some more immediate to the time of the rebellion and others longer evolving (e.g., Edwards, 2013). Of the latter, mounting indebtedness among Catholic landholders in the decades preceding the rebellion is important. To default on a loan frequently meant the forfeiture of one's lands, and loans were thereby made by wealthy creditors seeking to increase their land holdings in Ireland during this pivotal era of expanding colonization (“plantation”) by English and Scottish settlers (Ohlmeyer, 1998). Unsurprisingly, therefore, accounts from the Depositions outline how some Catholics sought to destroy loan agreements (bonds) amid the chaos of 1641/42 (Canny, 1995, 2001). But it is a mistake to consider such factors unrelated to the climate (and environment). While the environment has often been oversimplistically (e.g., mono-causally) attributed a role in human affairs, its effective omission from many (perhaps most) histories similarly promotes more simplistic (or incomplete) understandings. Human societies cannot exist (and their histories did not occur) independent of the environment and its variability. Indeed, this inseparability or entanglement can be illustrated by returning to the 1641 Rebellion. Ludlow and Crampsie (2019) have shown a repeated association between harvest crises (of which there were many; Gillespie, 1989, 2018) and poor weather in the decades preceding the rebellion. They have also shown an association between these crises and borrowing, needed to cover harvest-related income shortfalls in the decades running up to 1641 (Ohlmeyer, 1998). Extreme weather was thus also entangled in the longer-term processes of mounting indebtedness, consequent loss of hereditary lands, and growing grievance of Catholics towards Protestant settlers and elites that contributed to the rebellion.
7.3 Widespread droughts and rinderpest in China and Japan
Adverse weather had already affected Chinese agricultural production in the years leading up to the eruptions of the early 1640s, with some of the wealthiest regions suffering food shortage and disease by the late 1630s. This was also at least partly the result of the Chongzhen Great Drought, featuring the 5 worst years of consecutive drought (1637–1641) in known Chinese history (Lamb, 1970; Atwell, 2001; Zheng et al., 2014; Chen et al., 2020). Food costs soared and lawlessness increased substantially. In April 1644, Beijing fell to a rebel army invading the capital from the economically devastated northwest (Liu et al., 2018). Only 6 weeks later the city fell again, this time to Manchu invaders, marking the start of a new era in Chinese political history (Atwell, 1986).
Likewise, a succession of very cool summers in northern Japan and floods and
droughts elsewhere in the country drastically reduced grain yields in the
late 1630s and early 1640s, resulting in the Great Kan'ei Famine (Endō, 1982; Atwell,
1986). Notably, the famine occurred in a period when the center of Japanese
rice cultivation was in the process of shifting from the south and west to
the north and east, driven by the desire of lords in these regions to
specialize in food production to exploit growing urban populations but
thereby placing the crop at greater risk of cold damage (Arakawa, 1974;
Tani, 1978) and at times propelling grain prices to unprecedented levels.
The famine was enhanced by the large-scale internal migration after the
Shimabara Rebellion (, Shimabara no ran, 1637–1638) as well as the extreme impoverishment of low-ranking members of the samurai class. Worse, a
rinderpest epizootic broke out in Kyushu in 1638 and could not be contained
so that mass cattle mortality occurred across western Japan in 1640, with
the consequent scarcity of working animals further reducing yields (Atwell,
1986).
Extreme weather and poor harvests thus certainly exacerbated the disastrous situations in China and Japan. As Atwell (1986) and Zheng et al. (2014) have argued, however, a deeper understanding of the terrible “famines” of the late 1630s and early 1640s and the role of weather therein must appreciate the larger economic and social problems that had been festering in the two countries for some time, including excessive public expenditure, rapid urban growth, and intense economic competition. These factors proved notably socially and politically disruptive once agricultural productivity could not be maintained.
The eruption cluster between 1636 and 1646 occurred at a time when climate, from a human perspective, had already started to worsen and when the Maunder Minimum phase of low solar activity was about to set in (Usoskin et al., 2014, and Owens et al., 2017). The eruptions also occurred in a period during which some assumed decadal effects of the 1600 Huaynaputina eruption (White et al., 2022) might still have been in place. Indeed, the climatic impact of volcanic eruptions is increasingly thought to trigger dynamical responses in several internal modes of the global climate system (Robock, 2000; Timmreck, 2012), in which responses can persist on multi-decadal timescales (Zanchettin et al., 2016; Helama et al., 2021). Whether the 1600 Huaynaputina eruption – known for inducing massive cooling in the years following (Stoffel et al., 2015) – might have triggered a cascade of North Atlantic sea-ice–ocean feedbacks and ultimately led to a persistent regime shift in ocean circulation, an increase in Nordic seas sea ice extent on decadal timescales, a spin-up of the subpolar gyre, and a persistent, basin-wide cooling (Lehner et al., 2013; Schleussner and Feulner, 2013) merits further investigation (White et al., 2022). If correct, colder conditions would already have prevailed over Europe at the time of the eruptions of Hekla in 1636, Komaga-take on 31 July 1640, Mount Parker on 4 January 1641, and Shiveluch in 1646. Contemporary historical sources and tree-ring-based reconstructions confirm the occurrence of various years of unusual cold in the early 17th century. If sulfur injections as reflected in Greenland ice cores in 1636 and 1646 may have been too small to cause significant climatic perturbations alone, the volcanic gas emissions from Mount Parker in 1641 – and likely also that of Komaga-take in 1640 – certainly had an impact on the modes of internal climate variability as well as on (summer) temperatures (Stoffel et al., 2015; Guillet et al., 2017). However, in a context of generalized climate worsening and pre-existing widespread societal conflicts at a time of “general crisis” (Atwell, 1986, 2001; Parker, 1979; Parker and Smith, 1997), any direct attribution of volcanic impacts on Northern Hemisphere climate – and thereafter on societal developments – necessarily runs the risk of incorrectly assuming that correlation means causation.
Yet it is evident that meteorological extremes – including those volcanically induced – impacted societies, particularly when survival leaned on a fragile food surplus, itself heavily predicated on the cultivation of cereals. A late European winter frost or flood could have devastating effects on summer harvests and potentially propel elevated bread prices, as in the case of the French Revolution. Thus, with a growing abundance of well-dated proxy reconstructions of climate and volcanic forcing, recent studies have increasingly examined impacts of volcanism and extreme weather on historical events and societal developments (e.g., Zhang et al., 2011; Büntgen et al., 2011, 2020; Manning et al., 2017; Campbell and Ludlow, 2020; McConnell et al., 2020; Gao et al., 2021b). Other studies have in various ways highlighted the importance of conducting such investigations with an understanding of the relevant historical and societal contexts that are needed to avoid simplistic, mono-causal, or environmentally deterministic linkages between extreme weather or climate anomalies and historical events (Haldon et al., 2018, 2020; van Bavel et al., 2019; Chen et al., 2020; D'Arrigo et al., 2020; Degroot et al., 2021; Huhtamaa et al., 2021). Our present contribution has thus sought to provide a summary of both the climatic and societal contexts to guide further studies.
At the same time a reactionary tendency exists to seek to minimize the role of climate (and more broadly the environment) in human affairs, whether by indiscriminately applying the label of environmental determinism to studies that acknowledge the complexity of human–environmental relationships and do not posit simplistic mono-causal influences of climate on society (e.g., see Moreland's, 2018, characterization of Manning et al., 2017, versus the assessment by van Bavel et al., 2019) or by adopting a stance (either explicitly or implicitly) in which climatic and human factors are considered in opposition as explanatory factors (e.g., Gillespie, 2020). Here we argue that the way forward is to instead recognize that all human history has taken place within socio-ecological systems created by continually evolving human interactions with a changing environment (Ludlow et al., 2022). Embedded within this perspective is an understanding that forces such as climate and extreme weather have the effects they have because of the systems humans have created. These systems must always pay at least some regard to the prevailing environment (lest everyone starve), but major impacts can still occur when extremes are poorly adapted for, being ill-recognized or beyond available experience, or deemed too costly or infrequent to merit fuller adaptation (decisions that are contingent upon prevailing societal structures, e.g., extremes may be poorly adapted to in stratified societies that insulate elites from food crises and allow other (political, religious, etc.) imperatives to take precedence).
In this manner, the climatic shocks wrought by episodes of explosive volcanism such as the cluster of eruptions between 1636 and 1646 can, in the study of their impacts and the responses they provoked or failed to provoke, act as “revelatory crises” (Solway, 1994). These can serve to reveal important aspects of the underlying cultural, geopolitical, and socio-economic contexts in which they occurred and which indeed facilitated their impacts but which might otherwise have remained unquestioned or latent (hidden from or impervious to the scrutiny of contemporaries and later scholars).
The reconstructed VSSI and SAOD data in Figs. 1 and 2 are available at https://doi.org/10.1594/WDCC/eVolv2k_v2 (Toohey and Sigl, 2017a). All codes used to reproduce Figs. 4, 5, and 6 can be found at https://doi.org/10.1038/s41598-020-63339-3 (Guillet et al., 2020a). Underlying data for gridded temperatures used to perform our analysis as well as our results have been uploaded to Zenodo and are accessible at https://doi.org/10.5281/zenodo.3724674 (Guillet et al., 2020b) and in the published literature cited in the references.
The supplement related to this article is available online at: https://doi.org/10.5194/cp-18-1083-2022-supplement.
MaS and ChCo designed the study. ChCo and SG provided paleoclimate reconstructions. MiS and JM provided ice core records. ChCo, SH, and SG provided tree-ring data, whereas FL, HH, EG, SG, AC, KK, ChCa, and CG provided historical observations. All authors provided societal impacts and adaptation analysis as well as figures, discussed methods and results, and commented on the paper.
At least one of the (co-)authors is a member of the editorial board of Climate of the Past. The peer-review process was guided by an independent editor, and the authors also have no other competing interests to declare.
Publisher's note: Copernicus Publications remains neutral with regard to jurisdictional claims in published maps and institutional affiliations.
This article is part of the special issue “Interdisciplinary studies of volcanic impacts on climate and society”. It is not associated with a conference.
Markus Stoffel, Christophe Corona, and Sébastien Guillet received funding from the Swiss National Science Foundation through the SNSF Sinergia CALDERA project (grant agreement no. CRSII5_183571). Michael Sigl received funding from the European Research Council under the European Union's Horizon 2020 research and innovation program (grant agreement no. 820047). Francis Ludlow received funding from the EU Horizon 2020 Marie Sklodowska-Curie CLIMCONFLICT project (grant no. 70918) and the Irish Research Council's Starting Laureate Award CLICAB project (IRCLA/2017/303). Michael Sigl received funding from the Geoscience Department and the NFR TOPPFORK project “VIKINGS” (grant no. 275191) at the University of Oslo. Chaochao Gao received funding from the Natural Science Foundation of China (grant no. 41875092). Arlene Crampsie received funding from Irish Research Council project COALESCE/2019/43. This paper benefited from discussion facilitated by the “Volcanic Impacts on Climate and Society” (VICS) Working Group of PAGES, funded by the Swiss Academy of Sciences and the Chinese Academy of Sciences.
This research has been supported by the Schweizerischer Nationalfonds zur Förderung der Wissenschaftlichen Forschung (grant no. CRSII5_183571).
This paper was edited by Jürg Luterbacher and reviewed by Joseph Manning and one anonymous referee.
Abbott, P. M., Plunkett, G., Corona, C., Chellman, N. J., McConnell, J. R., Pilcher, J. R., Stoffel, M., and Sigl, M.: Cryptotephra from the Icelandic Veiðivötn 1477 CE eruption in a Greenland ice core: confirming the dating of volcanic events in the 1450s CE and assessing the eruption's climatic impact, Clim. Past, 17, 565–585, https://doi.org/10.5194/cp-17-565-2021, 2021.
Arakawa, H. (Ed.): Kikin [Famine], Kyōikusha, Tokyo, Japan, 1974.
Asao, N. (Ed.): Sakoku [The Closed Country], Shōgakkan, Tokyo, Japan, 1975.
Atwell, W. S.: Some observations on the “seventeenth-century crisis” in China and Japan, J. Asian Stud., 45, 223–244, https://doi.org/10.2307/2055842, 1986.
Atwell, W. S.: Volcanism and short-term climatic change in East Asian and World history, c. 1200-1699, J. World Hist., 12, 29–98, https://doi.org/10.1353/jwh.2001.0002, 2001.
Brázdil, R., Valášek, H., and Kotyza, O.: Meteorological records of Michel Stüeler of Krupka and their contribution to the kn,owledge of the climate of the Czech Lands in 1629–1649, in: Czech Geography at the Dawn of the Millenium, edited by: Drbohlav, D., Kalvoda, J., and Voženílek, V., Palacky University, Olomouc, Czech Geographic Society, 95–112, ISBN: 80-244-0858-9, 2004.
Baulant, M.: Wheat prices in Paris from 1431 to 1788, Ann. Hist. Sci. Soc., 23, 520–540, https://doi.org/10.3406/ahess.1968.421930, 1968.
Booth, B. B. B., Dunstone, N. J., Halloran, P. R., Andrews, T., and Bellouin, N.: Aerosols implicated as a prime driver of twentieth-century North Atlantic climate variability, Nature, 484, 228–232, https://doi.org/10.1038/nature10946, 2012.
Brehm, N., Bayliss, A., Christl, M., Synal, H. A., Adolphi, F., Beer, J., Kromer, B., Muscheler, R., Solanki, S. K., Usoskin, I., Bleicher, N., Bollhalder, S., Tyers, C., and Wacker, L.: Eleven-year solar cycles over the last millennium revealed by radiocarbon in tree rings, Nat. Geosci., 14, 10–15, https://doi.org/10.1038/s41561-020-00674-0, 2021.
Brönnimann, S., Franke, J., Nussbaumer, S. U., Zumbühl, H. J., Steiner, D., Trachsel, M. Hegerl, G. C., Schurer, A., Worni, M., Malik, A., Flückiger, J., and Raible, C. C.: Last phase of the Little Ice Age forced by volcanic eruptions, Nat. Geosci., 12, 650–656, https://doi.org/10.1038/s41561-019-0402-y, 2019.
Brown, S. K., Crosweller, H. S., Sparks, R. S. J., Cottrell, E., Deligne, N. I., Guerrero, N. O., Hobbs, L., Kiyosugi, K., Loughlin, S. C., Siebert, L., and Takarada, S.: Characterisation of the Quaternary eruption record: analysis of the Large Magnitude Explosive Volcanic Eruptions (LaMEVE) database, Journal of Applied Volcanology, 3, 5, https://doi.org/10.1186/2191-5040-3-5, 2014.
Büntgen, U., Tegel, W., Nicolussi, K., McCormick, M., Frank, D., Trouet, V., Kaplan, J. O., Herzig, F., Heussner, K.-U., Wanner, H., Luterbacher, J., and Esper, J.: 2500 years of European climate variability and human susceptibility, Science, 331, 578–582, https://doi.org/10.1126/science.1197175, 2011.
Büntgen, U., Arseneault, D., Boucher, É., Churakova (Sidorova), O. V., Gennaretti, F., Crivellaro, A., Hughes, M. K., Kirdyanov, A. V., Klippel, L., Krusic, P. J., Linderholm, H. W., Ljungqvist, F. C., Ludescher, J., McCormick, M., Myglan, V. S., Nicolussi, K., Piermattei, A., Oppenheimer, C., Reinig, F., Sigl, M., Vaganov, E. A., and Esper, J.: Prominent role of volcanism in Common Era climate variability and human history, Dendrochronologia, 64, 125757, https://doi.org/10.1016/j.dendro.2020.125757, 2020.
Canny, N.: What really happened in Ireland in 1641, in: Ireland from independence to occupation, 1641–1660, edited by: Ohlmeyer, J. H., Cambridge University Press, Cambridge, 24–42, ISBN: 9780521434799, 1995.
Canny, N. (Ed.): Making Ireland British, 1580–1650, Oxford University Press, Oxford, United Kingdom, ISBN: 9780198200918, 2001.
Campbell, B. M. S. and Ludlow, F.: Climate, disease and society in late-medieval Ireland, P. Roy. Irish Acad., 120C, 159–252, https://doi.org/10.3318/priac.2020.120.13, 2020.
Chen, K., Ning, L., Liu, Z., Liu, J., Yan, M., Sun, W., Yuan, L., Lv, G., Li, L., Jin, C., and Shi, Z.; One drought and one volcanic eruption influenced the history of China: The late Ming Dynasty mega-drought, Geophys. Res. Lett., 47, e2020GL088124, https://doi.org/10.1029/2020GL088124, 2020.
Christiansen, B.: Volcanic eruptions, large-scale modes in the Northern Hemisphere, and the El Niño–Southern Oscillation, J. Climate, 21, 910–922, https://doi.org/10.1175/2007JCLI1657.1, 2008.
Christiansen, B. and Ljungqvist, F. C.: Challenges and perspectives for large-scale temperature reconstructions of the past two millennia, Rev. Geophys., 55, 40–96, https://doi.org/10.1002/2016RG000521, 2017.
Cowtan, K. and National Center for Atmospheric Research Staff (Eds): The Climate Data Guide: Global surface temperatures, BEST: Berkeley Earth Surface Temperatures, https://climatedataguide.ucar.edu/climate-data/global-surface- temperatures-best-berkeley-earth-surface-temperatures (last access: 9 May 2022), 2019.
Cranford, J. (Ed.): The Teares of Ireland, Iohn Rothwell, London, United Kingdom, 1642.
Crowley, T.: Causes of climate change over the past 1000 years, Science, 289, 270–277, https://doi.org/10.1126/science.289.5477.270, 2000.
Crowley, T. J. and Unterman, M. B.: Technical details concerning development of a 1200 yr proxy index for global volcanism, Earth Syst. Sci. Data, 5, 187–197, https://doi.org/10.5194/essd-5-187-2013, 2013.
DallaSanta, K., Gerber, E. P., and Toohey, M.: The circulation response to volcanic eruptions: The key roles of stratospheric warming and Eddy interactions, J. Climate, 32, 1101–1120, https://doi.org/10.1175/JCLI-D-18-0099.1, 2019.
D'Arrigo, R., Klinger, P., Newfield, T., Rydval, M., and Wilson, R.: Complexity in crisis: The volcanic cold pulse of the 1690s and the consequences of Scotland's failure to cope, J. Volcanol. Geoth. Res., 389, 106746, https://doi.org/10.1016/j.jvolgeores.2019.106746, 2020.
David, C. P. C., Dulce, R. G., Nolasco-Javier, D. D., Zamoras, L. R., Jumawan, F. T., and Newhall, C. G.: Changing proportions of two pumice types from the June 15, 1991 eruption of Mount Pinatubo, in: Fire and Mud: Eruptions und Lahars of Mount Pinatubo, Philippines, edited by: Newhall, C. G. and Punongbayan, R. S., University of Washington Press, Seattle, United States, 681–685, 1996.
Davis, H. B. (Ed.): Generals in Khaki, Pentland Press, Raleigh N.C., United States, ISBN: 1571970886, 1998.
Degroot, D., Anchukaitis, K., Bauch, M., Burnham, J., Carnegy, F., Cui, J., de Luna, K., Guzowski, P., Hambrecht, G., Huhtamaa, H., Izdebski, A., Kleemann, K., Moesswilde, E., Neupane, N., Newfield, T., Pei, Q., Xoplaki, E., and Zappia, N.: Towards a rigorous understanding of societal responses to climate change, Nature, 591, 539–550, https://doi.org/10.1038/s41586-021-03190-2, 2021.
Delfin Jr., F. G., Newhall, C. G., and Martinez, M. L.: Geological and historical evidence for a 17th century eruption of Parker volcano, Mindanao, Philippine, Journal of the Geological Society of the Philippines, 52, 25–42, 1997.
Demarée, G. R.: Haarrauch, un trouble atmospherique ou un trouble environnemental et medical au XIX siècle, in: La brume et le brouillard dans la science, la littérature et les arts, edited by: Becker, K. and Leplatre, O., Hermann Editions, Paris, France, 129–143, ISBN: 978-2-7056-8803-5, 2014.
Dogar, M. M. and Sato, T.: Regional climate response of Middle Eastern, African, and South Asian monsoon regions to explosive volcanism and ENSO forcing, J. Geophys. Res.-Atmos., 124, 7580–7598, https://doi.org/10.1029/2019JD030358, 2019.
Dorney, J.: The Eleven Years War 1641-52 – A brief overview, https://www.theirishstory.com/2014/01/10/the-eleven-years-war-a-brief-overview/, last access: 15 February 2022.
Drixler, F. (Ed.): Mabiki Infanticide and Population Growth in Eastern Japan, 1660-1950, California University Press Scholarship Online, United States, https://doi.org/10.1525/california/9780520272439.001.0001, 2013.
Eddy, J. A.: The Maunder minimum, Science, 192, 1189–1202, https://doi.org/10.1126/science.192.4245.1189, 1976.
Edvinsson, R. and Söderberg, J.: The evolution of Swedish consumer prices 1290–2008, in: Historical Monetary and Financial Statistics for Sweden, Volume I: Exchange Rates, Prices, and Wages, 1277–2008, edited by: Edvinsson, R., Jacobson, T., and Waldenström, D., Sveriges Riksbank and Ekerlids, Stockholm, Sweden, 412–452, ISBN: 978-91-87391-29-3, 2010.
Edvinsson, R., Leijonhufvud, L., and Söderberg, J.: Väder, skördar och priser i Sverige, in: Agrarhistoria på många sätt: 28 studier om människan och jorden. Festskrift till Janken Myrdal på hans 60-årsdag, edited by: Liljewall, B., Flygare, I. A., Lange, U., Ljunggren, L., and Söderberg, J., Kungl. Skogs- och lantbruksakademien, Stockholm, Sweden, 115–136, ISSN: 1102-9048, 2009.
Edwards, D.: Out of the blue? Provincial unrest in Ireland before 1641, in: Ireland 1641: Contexts and Reactions, edited by: Ó Siochrú, M., and Ohlmeyer, J., Manchester University Press, Manchester, United Kingdom, 95–114, ISBN: 0719088178, 2013.
Endō, M. (Ed.): Kinsei seikatsushi nempyo [A chronological record of early-modern life], Yuizankaku, Tokyo, Japan, 1982.
Fischer, E. M., Luterbacher, J., Zorita, E., Tett, S. F. B., Casty, C., and Wanner, H.: European climate response to tropical volcanic eruptions over the last half millennium, Geophys. Res. Lett., 34, L05707, https://doi.org/10.1029/2006GL027992, 2007.
Gao, C. C., Oman, L., Robock, A., and Stenchikov, G. L.: Atmospheric volcanic loading derived from bipolar ice cores: Accounting for the spatial distribution of volcanic deposition, J. Geophys. Res., 112, D09109, https://doi.org/10.1029/2006JD007461, 2007.
Gao, C. C., Robock, A., and Ammann, C.: Volcanic forcing of climate over the past 1500 years: An improved ice core-based index for climate models, J. Geophys. Res., 113, D23111, https://doi.org/10.1029/2008JD010239, 2008.
Gao, C. C., Gao, Y., Zhang, Q., and Shi, C.: Climatic aftermath of the 1815 Tambora eruption in China, J. Meteorol. Res., 31, 28–38, https://doi.org/10.1007/s13351-017-6091-9, 2017.
Gao, C. C., Yan L. S., and Liu, F.: Hydroclimatic anomalies in China during the post-Laki years and the role of concurring El Niño, Advances in Climate Change Research, 12, 187–198, https://doi.org/10.1016/j.accre.2021.03.006, 2021a.
Gao, C. C., Ludlow, F., Matthews, A., Stine, A. R., Robock, A., Pan, Y., Breen, R., Nolan, B., and Sigl, M.: Volcanic climate impacts act as ultimate and proximate causes of Chinese dynastic collapse, Communications Earth & Environment, 2, 234, https://doi.org/10.1038/s43247-021-00284-7, 2021b.
Garnier, E. (Ed.): Les dérangements du temps, 500 ans de chaud et froids en Europe, Plon, Paris, France, ISBN: 978-2259208987, 2010 (in French).
Garnier, E.: A historic experience for a strengthened resilience. European societies in front of hydro-meteors 16th–20th centuries, in: Prevention of Hydrometeorological Extreme Events – Interfacing Sciences and Policies, edited by: Quevauviller, P., John Wiley & Sons, Chichester, 3–26, https://doi.org/10.1002/9781118629567, 2015.
Garnier, E.: Historic drought from archives: Beyond the instrumental record, in: Drought Science and Policy, edited by: Iglesias, A., John Wiley and Sons, Chichester, United Kingdom, 45–67, ISBN: 978-1-119-01707-3, 2019.
Garnier, E., Daux, V., Yiou, P., and Garcia de Cortazar, I.: Grapevine harvest dates in Besançon between 1525 and 1847: Social outcome or climatic evidence?, Climatic Change, 104, 703–727, https://doi.org/10.1007/s10584-010-9810-0, 2011.
Gillespie, R.: Meal and money: The harvest crisis of 1621–4 and the Irish economy, in: Famine: The Irish Experience, 900–1900: Subsistence Crises and Famines in Ireland, edited by: Crawford, E. M., J. Donald, Edinburgh, United Kingdom, 75–95, ISBN: 9780859762199, 1989.
Gillespie, R.: Economic life, 1550–1730, in: The Cambridge History of Ireland: Volume 2, Early Modern Ireland, 1550–1730, edited by: Ohlmeyer, J., Cambridge University Press, Cambridge, United Kingdom, 531–554, https://doi.org/10.1017/9781316338773, 2018.
Gillespie, R.: Climate, weather and social change in seventeenth-century Ireland, P. Roy. Irish Acad., 120C, 253–271, https://doi.org/10.3318/priac.2020.120.07, 2020.
Global Volcanism Program (GVP): Hokkaido-Komagatake (285020), in: Volcanoes of the World, v. 4.8.8, edited by: Venzke, E., Smithsonian Institution, https://doi.org/10.5479/si.GVP.VOTW4-2013, 2013a.
Global Volcanism Program (GVP): Parker (271011), in: Volcanoes of the World, v. 4.8.8, edited by: Venzke, E., Smithsonian Institution, https://doi.org/10.5479/si.GVP.VOTW4-2013, 2013b.
Gotthard, A. (Ed.): Der Dreißigjährige Krieg. eine Einführung, Böhlau Verlag, Cologne, Weimar, Wien, ISBN: 9783825245559, 2016.
Gray, L. J., Beer, J., Geller, M., Haigh, J. D., Lockwood, M., Matthes, K., Cubasch, U., Fleitmann, D., Harrison, G., Hood, L., Luterbacher, J., Meehl, G. A., Shindell, D., Geel, B. V., and White, W.: Solar influences on climate, Rev. Geophys., 48, RG4001, https://doi.org/10.1029/2009RG000282, 2010.
Grove, J. M. (Ed.): Recent Climatic Fluctuations The Little Ice Age, Methuen, London, ISBN 0-416-31540-2, 1988.
Guillet, S., Corona, C., Stoffel, M., Khodri, M., Lavigne, F., Ortega, P., Eckert, N., Selenniou, P., Daux, V., Churakova (Sidorova), O., Davi, N., Edouard, J.L., Yong, Z., Luckman, B. H., Myglan, V. S., Guiot, J., Beniston, M., Masson-Delmotte, V., and Oppenheimer, C.: Climate response to the Samalas volcanic eruption in 1257 revealed by proxy records, Nat. Geosci., 10, 123–128, https://doi.org/10.1038/ngeo2875, 2017.
Guillet, S., Corona, C., Ludlow, F. M., Oppenheimer, C., and Stoffel, M.: Climatic and societal impacts of a “forgotten” cluster of volcanic eruptions in 1108-1110 CE, Sci. Rep., 10, 6715, https://doi.org/10.1038/s41598-020-63339-3, 2020a.
Guillet, S., Corona, C., Ludlow, F., Oppenheimer, C., and Stoffel, M.: Climatic and societal impacts of a “forgotten” cluster of volcanic eruptions in 1108–1110 CE, in: Scientific Reports, Zenodo [data set], https://doi.org/10.5281/zenodo.3724674, 2020b.
Haldon, J., Mordechai, L., Newfield, T. P., Chase, A. F., Izdebski, A., Guzowski, P., Labuhn, I., and Roberts, N.: History meets palaeoscience: Consilience and collaboration in studying past societal responses to environmental change, P. Natl. Acad. Sci. USA, 115, 3210–3218, https://doi.org/10.1073/pnas.1716912115, 2018.
Haldon, J., Chase, A. F., Eastwood, W., Medina-Elizalde, M., Izdebski, A., Ludlow, F., Middleton, G., Mordechai, L., Nesbitt, J., and Turner II, B. L.: Demystifying Collapse: Climate, Environment, and Social Agency in Pre-Modern Societies, Millennium, 17, 33 pp., https://doi.org/10.1515/mill-2020-0002, 2020.
Hansen, J., Nazarenko, L., Ruedy, R., Sato, M., Willis, J., Del Genio, A., Koch, D., Lacis, A., Lo, K., Menon, S., Novakov, T., Perlwitz, J., Russell, G., Schmidt, G. A., and Tausnev, N.: Earth's energy imbalance: Confirmation and implications, Science, 308, 1431–1435, https://doi.org/10.1126/science.1110252, 2005.
Hegerl, G. C., Luterbacher, J., González-Rouco, F. J., Tett, S., Crowley, T., and Xoplaki, E.: Influence of human and natural forcing on European seasonal temperatures, Nat. Geosci., 4, 99–103, https://doi.org/10.1038/ngeo1057, 2011.
Helama, S., Stoffel, M., Hall, R. J., Jones, P. D., Arppe, L., Matskovsky, V. V., Timonen, M., Nöjd, P., Mielikäinen, K., and Oinonen, M.: Recurrent transitions to Little Ice Age-like climatic regimes over the Holocene, Clim. Dynam., 56, 3817–3833, https://doi.org/10.1007/s00382-021-05669-0, 2021.
Hevelius, J.: Selenographia sive lunae descriptio, Rar 8932, ETH-BIB, Zürich, 1647.
Holzhauser, H., Magny, M., and Zumbühl, H. J.: Glacier and lake-level variations in west-central Europe over the last 3500 years, Holocene, 15, 789–780, https://doi.org/10.1191/0959683605hl853ra, 2005.
Huhtamaa, H. and Helama, S.: Distant impact: tropical volcanic eruptions and climate-driven agricultural crises in seventeenth-century Ostrobothnia, Finland, J. Hist. Geogr., 57, 40–51, https://doi.org/10.1016/j.jhg.2017.05.011, 2017.
Huhtamaa, H., Stoffel, M., and Corona, C.: Recession or resilience? Long-range socioeconomic consequences of the 17th century volcanic eruptions in the far north, Clim. Past Discuss. [preprint], https://doi.org/10.5194/cp-2021-147, in review, 2021.
Iles, C. E., Hegerl, G. C., Schurer, A. P., and Zhang, X.: The effect of volcanic eruptions on global precipitation, J. Geophys. Res.-Atmos., 118, 8770–8786, https://doi.org/10.1002/jgrd.50678, 2013.
Jensen, B. J. L., Pyne-O'Donnell, S., Plunkett, G., Froese, D. G., Hughes, P. D. M., Sigl, M., McConnell, J. R., Amesbury, M. J., Blackwell, P. G., van den Bogaard, C., Buck, C. E., Charman, D. J., Clague, J. J., Hall, V. A., Koch, J., Mackay, H., Mallon, G., McColl, L., and Pilcher, J. R.: Transatlantic distribution of the Alaskan White River ash, Geology, 42, 875–878, https://doi.org/10.1130/G35945.1, 2014.
Jespersen, L.: Fiscal and military developments, in: The Cambridge History of Scandinavia. Volume 2: 1520–1870, edited by: Kouri, E. I. and Olesen, J. E., Cambridge University Press, Cambridge, United Kingdom, 326–342, ISBN 978-1-316-65544-3, 2016.
Johanson, V. F. (Ed.): Finlands agrarpolitiska historia: en skildring av det finländska lantbrukets ekonomiska betingelser. Från 1600-talet till år 1870, Suomen Maataloustieteellinen Seura, 1924.
Jungclaus, J. H., Bard, E., Baroni, M., Braconnot, P., Cao, J., Chini, L. P., Egorova, T., Evans, M., González-Rouco, J. F., Goosse, H., Hurtt, G. C., Joos, F., Kaplan, J. O., Khodri, M., Klein Goldewijk, K., Krivova, N., LeGrande, A. N., Lorenz, S. J., Luterbacher, J., Man, W., Maycock, A. C., Meinshausen, M., Moberg, A., Muscheler, R., Nehrbass-Ahles, C., Otto-Bliesner, B. I., Phipps, S. J., Pongratz, J., Rozanov, E., Schmidt, G. A., Schmidt, H., Schmutz, W., Schurer, A., Shapiro, A. I., Sigl, M., Smerdon, J. E., Solanki, S. K., Timmreck, C., Toohey, M., Usoskin, I. G., Wagner, S., Wu, C.-J., Yeo, K. L., Zanchettin, D., Zhang, Q., and Zorita, E.: The PMIP4 contribution to CMIP6 – Part 3: The last millennium, scientific objective, and experimental design for the PMIP4 past1000 simulations, Geosci. Model Dev., 10, 4005–4033, https://doi.org/10.5194/gmd-10-4005-2017, 2017.
Katsui, Y. and Chimoto, Y.: The 1640 eruption and its products of Komagatake Volcano, Hokkaido. Sec. Series Bull. Volcanol. Soc. Japan, 30, p. 94, https://doi.org/10.18940/kazanc.30.2_94_1, 1985.
Katsui, Y. and Ishikawa, T.: Eruptive history, studies of eruption products, disaster map and evaluation of disasters. Characteristic features of damage due to volcanic eruptions, preparation of hazard map, and prediction of hazard, Rep. Spec. Proj. Sci. Stud. Nat. Disaster, A-56-1, 23–29, 1981.
Katsui, Y., Yokoyama, I., Fujita, T., and Ehara, S. (Eds.): Komagatake, Report of the Volcanoes in Hokkaido, Part 4. Committee Prevention Natural Disasters Hokkaido, Sapporo, Japan, 1975.
Katsui, Y., Shinosawa, T., Chimoto, Y., and Yamada, H.: Historical pyroclastic flows at Hokkaido Komagatake, Rep. Spec. Proj. Sci. Stud. Nat. Disaster, 61, 91–113, 1986.
Khodri, M., Izumo, T., Vialard, J., Janicot, S., Cassou, C., Lengaigne, M., Mignot, J., Gastineau, G., Guilyardi, E., Lebas, N., Robock, A., and McPhaden, M.J.: Tropical explosive volcanic eruptions can trigger El Niño by cooling tropical Africa, Nat. Commun., 8, 1–13, https://doi.org/10.1038/s41467-017-00755-6, 2017.
Kleemann, K.: Telling stories of a changed climate: The Laki fissure eruption and the interdisciplinarity of climate history, in: Communicating the Climate: From Knowing Change to Changing Knowledge, edited by: Kleemann, K. and Oomen, J., RCC Perspectives: Transformations in Environment and Society, 4, 33–42, https://doi.org/10.5282/rcc/8822, 2019.
Lamb, H. H.: Volcanic dust in the atmosphere; with a chronology and assessment of its meteorological significance, Philos. T. Roy. Soc. A., 266, 425–533, https://doi.org/10.1098/rsta.1970.0010, 1970.
Lång, J.: Hämeestä, Uudeltamaalta ja Varsinais-Suomesta vuosien 1626–1678 väenotoissa otetut uudet sotilaat ja isäntien osuus heistä, in: Suomen maatalouden historia 1, edited by: Rasila, V., Jutikkala, E., and Mäkelä-Alitalo, A., Finnish Literature Society, Helsinki, Finland, p. 204, ISBN 9517464819, 2003.
Lappalainen, J. T.: Valtiopäiviltä väenottopaikalle. Suomen jalkaväen rekrytointi 1638-1649, Tiede ja ase, 45, 62–84, https://journal.fi/ta/article/view/47690 (last access: 9 May 2022), 1987.
Lavigne, F., Degeai, J. P., Komorowski, J. C., Guillet, S., Robert, V., Lahitte, P., Oppenheimer, C., Stoffel, M., Vidal, C. M., Surono, I. P., Wassmer, P., Hajdas, I., Hadmoko, D. S., and De Bélizal, E.: Source of the great A.D. 1257 mystery eruption unveiled, Samalas volcano, Rinjani Volcanic Complex, Indonesia, P. Natl. Acad. Sci. USA, 110, 16742–16747, https://doi.org/10.1073/pnas.1307520110, 2013.
Lehner, F., Born, A., Raible, C. C., and Stocker, T. F.: Amplified inception of European Little Ice Age by sea ice–ocean–atmosphere feedbacks, J. Climate, 26, 7586–7602, https://doi.org/10.1175/JCLI-D-12-00690.1, 2013.
Leijonhufvud, L. (Ed.): Grain tithes and manorial yields in early modern Sweden: Trends and patterns of production and productivity c. 1540–1680, Swedish University of Agricultural Sciences, Uppsala, Sweden, ISBN: 9157658293, 2001.
Lenihan, P.: War and population, Irish Economic and Social History, 24, 1–21, https://www.jstor.org/stable/24340829 (last access: 9 May 2022), 1997.
Le Roy Ladurie, E. (Ed.): Histoire humaine et comparée du climat, Fayard, Paris, France, ISBN: 978-2213619217, 2004.
Liu, Q., Li, G., Kong, D. Y., Huang, B. B., and Huang, Y. X.: Climate, disasters, wars and the collapse of the Ming Dynasty, Environ. Earth Sci., 77, 44, https://doi.org/10.1007/s12665-017-7194-4, 2018.
Liu, W. G. (Ed.): The Chinese Market Economy, 1000–1500, SUNY Press, Albany, New York, ISBN: 978-1438455686, 2015.
Ljungqvist, F. C., Seim, A., Krusic, P. J., González-Rouco, J. F., Werner, J. P., Cook, E. R., Zorita, E., Luterbacher, J., Xoplaki, E., Destouni, G., García Bustamante-Bustamante, E., Melo-Aguilar, C. A., Seftigen, K., Wang, J., Gagen, M. H., Esper, J., Fleitmann, D., Solomina, O., and Büntgen, U.: Warm-season temperature and hydroclimate co-variability across Europe since 850 CE, Environ. Res. Lett., 14, 084015, https://doi.org/10.1088/1748-9326/ab2c7e, 2019.
Lorrey, A., Fauchereau, N., Stanton, C., Chappell, P., Phipps, S., Mackintosh, A., Renwick, J., Goodwin, J., and Fowler A.: The Little Ice Age climate of New Zealand reconstructed from Southern Alps cirque glaciers: a synoptic type approach, Clim. Dynam., 42, 3039–3060, https://doi.org/10.1007/s00382-013-1876-8, 2014.
Luckman, B. H.: The Little Ice Age in the Canadian Rockies, Geomorphology, 32, 357–384, https://doi.org/10.1016/S0169-555X(99)00104-X, 2000.
Ludlow, F. and Crampsie, A.: Environmental history of Ireland, 1550-1730, in: Cambridge History of Ireland: Volume 2, Early Modern Ireland, 1550-1730, edited by: Ohlmeyer, J., Cambridge University Press, Cambridge, 608–637, ISBN: 1107540461, 2018.
Ludlow, F. and Crampsie, A.: Climate, debt and conflict: environmental history as a new direction in understanding early modern Ireland, in: Early Modern Ireland: New Sources, M, and Directions, edited by: Covington, S., Carey, V., and McGowan-Doyle, V., Routledge, London, United Kingdom, ISBN: 9780815373940, 2019.
Ludlow, F., Stine, A. R., Leahy, P., Murphy, E., Mayewski, P., Taylor, D., Killen, J., Baillie, M., Hennessy, M., and Kiely, G.: Medieval Irish chronicles reveal persistent volcanic forcing of severe winter cold events, 431-1649 CE, Environ. Res. Lett., 8, L024035, https://doi.org/10.1088/1748-9326/8/2/024035, 2013.
Ludlow, F., Kostick, C., and Morris, C.: Climate, violence and ethnic conflict in the Ancient World, in: The Cambridge world history of genocide, Volume 1, edited by Kiernan, B., Lemos, T. M., and Taylor, T., Cambridge University Press, Cambridge, United Kingdom, in press, 2022.
Luterbacher, J., Dietrich, D., Xoplaki, E., Grosjean, M., and Wanner, H.: European seasonal and annual temperature variability, trends, and extremes since 1500, Science, 303, 1499–1503, https://doi.org/10.1126/science.1093877, 2004.
Manning, J. G., Ludlow, F., Stine, A. R. Boos, W., Sigl, M., and Marlon, J.: Volcanic suppression of Nile summer flooding triggers revolt and constrains interstate conflict in ancient Egypt, Nat. Commun., 8, 900, https://doi.org/10.1038/s41467-017-00957-y, 2017.
Mårtensson, L. (Ed.): Sakregister till Allmogens besvär till år 1720, Stockholm, Sweden, 1952.
Masiokas, M. H., Rivera, A., Espizua, L. E., Villalba, R., Delgado, S., and Aravena, J. C.: Glacier fluctuations in extratropical South America during the past 1000 years, Palaeogeogr. Palaeocl., 281, 242–268, https://doi.org/10.1016/j.palaeo.2009.08.006, 2009.
Mann, M. E., Zhang, Z., Rutherford, S., Bradley, R. S., Hughes, M. K., Shindell, D., Ammann, C., Faluvegi, G., and Ni, F.: Global signatures and dynamical origins of the Little Ice Age and Medieval Climate Anomaly, Science, 326, 1256–1260, https://doi.org/10.1126/science.1177303, 2009.
Matthews, J. A. and Briffa, K. R.: The `Little Ice Age': Re-evaluation of an evolving concept, Geogr. Ann. A, 87, 17–36, https://doi.org/10.1111/j.0435-3676.2005.00242.x, 2005.
McConnell, J. R., Sigl, M., Plunkett, G., Burke, A., Kim, W., Raible, C. C., Wilson, A. I., Manning, J. G., Ludlow, F. M., Chellman, N. J., Innes, H. M., Yang, Z., Larsen, J. F., Schaefer, J. R., Kipfstuhl, S., Mojtabavi, S., Wilhelms, F., Opel, T., Meyer, H., and Steffensen, J. P.: Extreme climate after massive eruption of Alaska's Okmok volcano in 43 BCE and effects on the late Roman Republic and Ptolemaic Kingdom, P. Natl. Acad. Sci. USA, 117, 15443–15449, https://doi.org/10.1073/pnas.2002722117, 2020.
Moreland, J. F.: AD536 – Back to nature?, Acta Archaeol., 89, 91–111, https://doi.org/10.1111/j.1600-0390.2018.12194.x, 2018.
Nagakura, T.: Kan'ei no kikin to bakufu no taiō [The Kan'ei famine and the bakufu's response], in: Edo jidai no kikin, edited by: Rekishi kōron, Yūzankaku, Tokyo, Japan, 1982.
Neukom, R., Steiger, N., Gómez-Navarro, J. J., Wang, J., and Werner, J. P.: No evidence for globally coherent warm and cold periods over the pre-industrial Common Era, Nature 571, 550–554, https://doi.org/10.1038/s41586-019-1401-2, 2019.
Nishimura, Y. and Miyaji, N.: Tsunami deposits from the 1993 Southwest Hokkaido earthquake and the 1640 Hokkaido Komagatake eruption, northern Japan, Pure Appl. Geophys., 144, 719–733, https://doi.org/10.1007/BF00874391, 1995.
Nussbaumer, S. U. and Zumbühl, H. J.: The Little Ice Age history of the Glacier des Bossons (Mont Blanc massif, France): a new high-resolution glacier length curve based on historical documents, Climatic Change, 111, 301–334, https://doi.org/10.1007/s10584-011-0130-9, 2012.
Nussbaumer, S. U., Zumbühl, H. J., and Steiner, D.: Fluctuations of the Mer de Glace (Mont Blanc area, France) AD 1500–2050: an interdisciplinary approach using new historical data and neural network simulations, Zeitschrift für Gletscherkunde und Glazialgeologie, 40, 5–140, 2007.
Ogilvie, A. E. J.: The impact of climate on grass growth and hay yield in Iceland: A.D. 1601 to 1780, in: Climate Changes on A Yearly to Millennial Basis, edited by: Morner, N.-A. and Karlen, W., Reidel, Dordrecht, 343–352, ISBN 978-94-015-7692-5, 1984.
Ohlmeyer, J.: The statute staple in early modern Ireland, History Ireland, 6, 36–40, 1998.
Ó Siochrú, M. (Ed.): Confederate Ireland, 1642–49: A Constitutional and Political Analysis, Four Courts Press, Dublin, Ireland, ISBN: 1851824006, 1999.
Outram, Q.: The demographic impact of early modern warfare, Soc. Sci. Hist., 26, 245–272, https://doi.org/10.1017/S0145553200012359, 2002.
Owens, M. J., Lockwood, M., Hawkins, E., Usoskin, I., Jones, G. S., Barnard, L., Schurer, A., and Fasullo, J.: The Maunder minimum and the Little Ice Age: an update from recent reconstructions and climate simulations, J. Space Weather Spac., 7, A33, https://doi.org/10.1051/swsc/2017034, 2017.
Parker, D. E., Wilson, H., Jones, P. D., Christy, J. R., and Folland, C. K.: The impact of Mount Pinatubo on world-wide temperatures, Int. J. Climatol., 16, 487–497, https://doi.org/10.1002/(SICI)1097-0088(199605)16:5<487::AID-JOC39>3.0.CO;2-J, 1996.
Parker, G. (Ed.): Europe in Crisis, 1598–1648, Fontana, Glasgow, United Kingdom, ISBN: 9780006356707, 1979.
Parker, G. (Ed.): The Thirty Years' War, Routledge, London, United Kingdom, https://doi.org/10.4324/9780203995495, 2006.
Parker, G. (Ed.): Crisis and catastrophe: the global crisis of the seventeenth century reconsidered, Am. Hist. Rev., 113, 1053–1079, https://doi.org/10.1086/ahr.113.4.1053, 2008.
Parker, G. (Ed.): Global Crisis: War, Climate Change and Catastrophe in the Seventeenth Century, Yale University Press, New Haven and London, United States and United Kingdom, ISBN: 9780300208634, 2013.
Parker, G. and Smith, L. M. (Eds.): The General Crisis of the Seventeenth Century, 2nd edn., Routledge, London and New York, United Kingdom and United States, ISBN: 9780415128827, 1997.
Pauling, A., Luterbacher, J., Casty, C., and Wanner, H.: Five hundred years of gridded high-resolution precipitation reconstructions over Europe and the connection to large-scale circulation, Clim. Dynam. 26, 387–405, https://doi.org/10.1007/s00382-005-0090-8, 2006.
Pfister, C. (Ed.): Klimageschichte der Schweiz 1525–1860. Das Klima der Schweiz von 1525 bis 1860 und seine Bedeutung in der Geschichte von Bevölkerung und Landwirtschaft, Haupt, Bern, Switzerland, ISBN: 9783258033198, 1984 (in German).
Rao, M. P., Cook, B. I., Cook, E. R., D'Arrigo, R. D., Krusic, P. J., Anchukaitis, K. J., LeGrande, A. N., Buckley, B. M., Davi, N. K. Leland, C., and Griffin, K. L.: European and Mediterranean hydroclimate responses to tropical volcanic forcing over the last millennium, Geophys. Res. Lett., 44, 5104–5112, https://doi.org/10.1002/2017GL073057, 2017.
Robock, A: Volcanic eruptions and climate, Rev. Geophys., 38, 191–219, https://doi.org/10.1029/1998RG000054, 2000.
Sasaki, T., Katayama, M., Otowa, M., and Amano, Y.: Volcanic ash in Oshima Peninsula, Report of the Hokkaido National Agricultural Experiment Station, 20, 255–286, 1970.
Schleussner, C. F. and Feulner, G.: A volcanically triggered regime shift in the subpolar North Atlantic Ocean as a possible origin of the Little Ice Age, Clim. Past, 9, 1321–1330, https://doi.org/10.5194/cp-9-1321-2013, 2013.
Schmidt, A. and Robock, A.: Volcanism, the atmosphere and climate through time, in: Volcanism and Global Environmental Change, edited by: Schmidt, A., Fristad, K. E., and Elkins-Tanton, L. T., Cambridge University Press, Cambridge, United Kingdom, 197–207, https://doi.org/10.1017/CBO9781107415683.017, 2015.
Schmidt, G. (Ed.): Die Reiter der Apokalypse: Geschichte des Dreissigjährigen Krieges, Verlag C.H. Beck, München, Germany, ISBN: 978-3-406-71836-6, 2018 (in German).
Seppel, M. (Ed.): Näljaabi Liivi- ja Eestimaal 17. sajandist 19. sajandi alguseni, University of Tartu Press, Tartu, Finland, ISBN: 9789949118847, 2008 (in Finnish).
Shen, C., Wang, W.-C., Hao, Z., and Gong, W.: Exceptional drought events over eastern China during the last five centuries, Clim. Change, 85, 453–471, https://doi.org/10.1007/s10584-007-9283-y, 2007.
Sigl, M., McConnell, J. R., Toohey, M., Curran, M., Das, S. B., Edwards, R., Isaksson, E., Kawamura, K., Kipfstuhl, S., Kruger, K., Layman, L., Maselli, O. J., Motizuki, Y., Motoyama, H., Pasteris, D. R., and Severi, M.: Insights from Antarctica on volcanic forcing during the Common Era, Nat. Clim. Change, 4, 693–697, https://doi.org/10.1038/nclimate2293, 2014.
Sigl, M., Winstrup, M., McConnell, J. R., Welten, K. C., Plunkett, G., Ludlow, F., Büntgen, U., Caffee, M., Chellman, N., Dahl-Jensen, D., Fischer, H., Kipfstuhl, S., Kostick, C., Maselli, O. J., Mekhaldi, F., Mulvaney, R., Muscheler, R., Pasteris, D. R., Pilcher, J. R., Salzer, M., Schupbach, S., Steffensen, J. P., Vinther, B. M., and Woodruff, T. E.: Timing and climate forcing of volcanic eruptions for the past 2,500 years, Nature, 523, 543–549, https://doi.org/10.1038/nature14565, 2015.
Sigl, M., Abram, N. J., Gabrieli, J., Jenk, T. M., Osmont, D., and Schwikowski, M.: 19th century glacier retreat in the Alps preceded the emergence of industrial black carbon deposition on high-alpine glaciers, The Cryosphere, 12, 3311–3331, https://doi.org/10.5194/tc-12-3311-2018, 2018.
Slawinska, J. and Robock, A.: Impact of volcanic eruptions on decadal to centennial fluctuations of Arctic Sea ice extent during the last millennium and on initiation of the Little Ice Age, J. Climate, 31, 2145–2167, https://doi.org/10.1175/JCLI-D-16-0498.1, 2018.
Smyth, W. J.: Towards a cultural geography of the 1641 rising/rebellion, in: Ireland 1641: Contexts and Reactions, edited by: Ó Siochrú, M. and Ohlmeyer, J., Manchester University Press, Manchester, United Kingdom, 71–94, https://doi.org/10.7765/9781784992033.00012, 2013.
Solomina, O., Pavlova, I., Curtis, A., Jacoby, G., Ponomareva, V., and Pevzner, M.: Constraining recent Shiveluch volcano eruptions (Kamchatka, Russia) by means of dendrochronology, Nat. Hazards Earth Syst. Sci., 8, 1083–1097, https://doi.org/10.5194/nhess-8-1083-2008, 2008.
Solomina, O. N., Bradley, R. S., Jomelli, V., Geirsdottir, A., Kaufman, D. S., Koch, J., McKay, N. P., Masiokas, M., Miller, G., Nesje, A., Nicolussi, K., Owen, L. A., Putnam, A. E., Wanner, H., Wiles, G., and Yang, B.: Glacier fluctuations during the past 2000 years, Quaternary Sci. Rev., 149, 61–90, https://doi.org/10.1016/j.quascirev.2016.04.008, 2016.
Solway, J. S.: Drought as a revelatory crisis: An exploration of shifting entitlements and hierarchies in the Kalahari, Botswana, Dev. Change, 25, 471–495, https://doi.org/10.1111/j.1467-7660.1994.tb00523.x, 1994.
Song, Z. H., Sun, G. L, Chen, R. P., Ding, Z. H., and Gao, J. G (eds.): Chronology of major natural disasters and anomalies in ancient China, Guangdong Education Publishing House, Guangzhou, China, ISBN 9787540621032, 1992 (in Chinese).
Soom, A. (Ed.): Die Politik Schwedens bezüglich des russischen Transithandels über die estnischen Städte in den Jahren 1636–1656, Õpetatud Eesti selts, Tartu, Finland, 1940 (in German).
Stoffel, M., Khodri, M., Corona, C., Guillet, S., Poulain, V., Bekki, S., Guiot, J., Luckman, B. H., Oppenheimer, C., Lebas, N., Beniston, M., and Masson-Delmotte, V.: Estimates of volcanic-induced cooling in the Northern Hemisphere over the past 1,500 years, Nat. Geosci., 8, 784–788, https://doi.org/10.1038/ngeo2526, 2015.
Stothers, R. B.: Stratospheric transparency derived from total lunar eclipse colors, 1665–1800, Pub. Astron. Soc. Pacific, 116, 886–893, https://doi.org/10.1086/425537, 2004.
Stothers, R. B.: Stratospheric transparency derived from total lunar eclipse colors, 1801–1881, Pub. Astron. Soc. Pacific, 117, 1445–1450, https://doi.org/10.1086/497016, 2005.
Tani, N.: Agricultural crop damage by weather hazards and the countermeasures in Japan, in: Climatic Change and Food Production, edited by: Takahashi, K. and Yoshino, M., University of Tokyo Press, Tokyo, Japan, 197–215, ISBN: 0860082059, 1978.
Tarand, A. and Nordli, P. Ø.: The Tallinn temperature series reconstructed back half a millennium by use of proxy data, in: The Iceberg in the Mist: Northern Research in Pursuit of a “Little Ice Age”, edited by: Ogilvie, A. E. and Jónsson, T., Springer, Dordrecht, Netherlands, 189–199, https://doi.org/10.1007/978-94-017-3352-6_9, 2001.
Tarand, A., Jaagus, J., and Kallis, A. (Eds.): Eesti kliima minevikus ja tänapäeval, University of Tartu Press, Tartu, Finalnd, ISBN: 978-9949323241, 2013.
Thorarinsson, S.: Hekla: A Notorious Volcano, Almenna Bókafelagid, Reykjavík, 48 pl., 61 pp., 1970.
Timmreck, C.: Modeling the climatic effects of large explosive volcanic eruptions, WIREs Clim. Change, 3, 545–564, https://doi.org/10.1002/wcc.192, 2012.
Timmreck, C., Toohey, M., Zanchettin, D., Brönnimann, S., Lundstad, E., and Wilson, R.: The unidentified eruption of 1809: a climatic cold case, Clim. Past, 17, 1455–1482, https://doi.org/10.5194/cp-17-1455-2021, 2021.
Toohey, M. and Sigl, M.: Reconstructed volcanic stratospheric sulfur injections and aerosol optical depth, 500 BCE to 1900 CE, version 2, World Data Center for Climate (WDCC) [data set] at DKRZ, https://doi.org/10.1594/WDCC/eVolv2k_v2, 2017a.
Toohey, M. and Sigl, M.: Volcanic stratospheric sulfur injections and aerosol optical depth from 500 BCE to 1900 CE, Earth Syst. Sci. Data, 9, 809–831, https://doi.org/10.5194/essd-9-809-2017, 2017b.
Toohey, M., Krüger, K., and Timmreck, C.: Volcanic sulfate deposition to Greenland and Antarctica: A modeling sensitivity study, J. Geophys. Res.-Atmos., 118, 4788–4800, https://doi.org/10.1002/jgrd.50428, 2013.
Toohey, M., Krüger, K., Sigl, M., Stordal, F., and Svensen, H.: Climatic and societal impacts of a volcanic double event at the dawn of the Middle Ages, Clim. Change, 136, 401–412, https://doi.org/10.1007/s10584-016-1648-7, 2016.
Toohey, M., Krüger, K., Schmidt, H., Timmreck, C., Sigl, M., Stoffel, M., and Wilson, R.: Disproportionately strong climate forcing from extratropical explosive volcanic eruptions, Nat. Geosci., 12, 100–107, https://doi.org/10.1038/s41561-018-0286-2, 2019.
Tsuji, Y., Kato, K., Arai, K., Han, S., and Yamanaka, Y.: Characteristics of the Southwest Hokkaido Tsunami, Kaiyo Monthly, 7, 110–122, 1994.
Usoskin, I. G., Hulot, G., Gallet, Y., Roth, R., Licht, A., Joos, F., Kovaltsov, G. A., Thébault E., and Khokhlov, A.: Evidence for distinct modes of solar activity, Astron. Astrophys., 562, L10, https://doi.org/10.1051/0004-6361/201423391, 2014.
van Bavel, B. J. P., Curtis, D. R., Hannaford, M. J., Moatsos, M., Roosen, J., and Soens, T.: Climate and society in long-term perspective: Opportunities and pitfalls in the use of historical datasets, Wires Clim. Change, 10, e611, https://doi.org/10.1002/wcc.611, 2019.
van Dijk, E., Jungclaus, J., Lorenz, S., Timmreck, C., and Krüger, K.: Was there a volcanic induced long lasting cooling over the Northern Hemisphere in the mid 6th–7th century?, Clim. Past Discuss. [preprint], https://doi.org/10.5194/cp-2021-49, in review, 2021.
Verbeek, R.: The Krakatau eruption, Nature, 30, 10–15, https://doi.org/10.1038/030010a0, 1884.
Villstrand, N. E.: Adaptation or protestation: local community facing the conscription of infantry for the Swedish armed forces, 1620–1679, in: A Revolution From Above? The Power State of 16th and 17th Century Scandinavia, edited by: Jespersen, L., Odense University Press, Denmark, 253–314, ISBN: 978-8778384072, 2000.
White, S., Moreno-Chamarro, E., Zanchettin, D., Huhtamaa, H., Degroot, D., Stoffel, M., and Corona, C.: The 1600 CE Huaynaputina eruption as a possible trigger for persistent cooling in the North Atlantic region, Clim. Past, 18, 739–757, https://doi.org/10.5194/cp-18-739-2022, 2022.
Wiles, G. C., Barclay, D. J., and Calkin, P. E.: Tree-ring-dated `Little Ice Age' histories of maritime glaciers from western Prince William Sound, Alaska, Holocene, 9, 163–173, https://doi.org/10.1191/095968399671927145, 1999.
Wilson, P. H.: Dynasty, constitution, and confession: the role of religion in the Thirty Years War, Int. Hist. Rev., 30, 473–514, https://doi.org/10.1080/07075332.2008.10415483, 2008.
Wilson, P. H. (Ed.): The Thirty Years War: Europe's Tragedy, Harvard University Press, Harvard, United States, ISBN: 978-0674062313, 2009.
Winchester, S. (Ed.): The Day The World Exploded: August 27, 1883, Harper Collins, New York, United States, 2003.
Xoplaki, E., Luterbacher, J., Paeth, H., Dietrich, D., Steiner N., Grosjean, M., and Wanner, H.: European spring and autumn temperature variability and change of extremes over the last half millennium, Geophys. Res. Lett., 32, L15713, https://doi.org/10.1029/2005GL023424, 2005.
Yamada, S.: Studies on the history of volcanic eruptions of Alluvium epoch in Hokkaido on the basis of depositional features of the pyroclastics, Monogr. Assoc. Geol. Collab., 8, 40 pp., 1958.
Yamaguchi, K. and Sasaki, J. (Eds.): Bakuhan taisei [The Bakuhan system], Nihon Hyōronsha, Tokyo, Japan, ISBN: 978-0060838591, 1971.
Yamamoto, H. (Ed.): Kan'ei jidai, Yoshikaw, Tokyo, Japan, 1989.
Yoshimoto, M. and Ui, T.: The 1640 sector collapse of Hokkaido Komagatake volcano, northern Japan, Bull. Volc. Soc. Japan (Kazan), 43, 137–148, https://doi.org/10.18940/kazan.43.4_137, 1998.
Yoshimoto, M., Furukawa, R., Nanayama, F., Nishimura, Y., Nishina, K., Uchida, Y., Takarada, S., Takahashi, R., and Hirohisa, K: Subaqueous distribution and volume estimation of the debris-avalanche deposit from the 1640 eruption of Hokkaido-Komagatake volcano, southwest Hokkaido, Japan, J. Geol. Soc. Japan, 109, 595–606, https://doi.org/10.5575/geosoc.109.595, 2003.
Zambri, B., LeGrande, A. N., Robock, A., and Slawinska, J.: Northern Hemisphere winter warming and summer monsoon reduction after volcanic eruptions over the last millennium, J. Geophys. Res.-Atmos., 122, 7971–7989, https://doi.org/10.1002/2017JD026728, 2017.
Zanchettin, D., Timmreck, C., Graf, H. F., Rubino, A., Lorenz, S., Lohmann, K., Krüger, K., and Jungclaus, J. H.: Bi-decadal variability excited in the coupled ocean–atmosphere system by strong tropical volcanic eruptions, Clim. Dynam., 39, 419–444, https://doi.org/10.1007/s00382-011-1167-1, 2012.
Zanchettin, D., Bothe, O., Graf, H. F., Lorenz, S. J., Luterbacher, J., Timmreck, C., and Jungclaus, J. H.: Background conditions influence the decadal climate response to strong volcanic eruptions, J. Geophys. Res.-Atmos., 118, 4090–4106, https://doi.org/10.1002/jgrd.50229, 2013.
Zanchettin, D., Khodri, M., Timmreck, C., Toohey, M., Schmidt, A., Gerber, E. P., Hegerl, G., Robock, A., Pausata, F. S. R., Ball, W. T., Bauer, S. E., Bekki, S., Dhomse, S. S., LeGrande, A. N., Mann, G. W., Marshall, L., Mills, M., Marchand, M., Niemeier, U., Poulain, V., Rozanov, E., Rubino, A., Stenke, A., Tsigaridis, K., and Tummon, F.: The Model Intercomparison Project on the climatic response to Volcanic forcing (VolMIP): experimental design and forcing input data for CMIP6, Geosci. Model Dev., 9, 2701–2719, https://doi.org/10.5194/gmd-9-2701-2016, 2016.
Zhang, D. E. (Ed.): A Compendium of Chinese Meteorological Records of the Last 3000 Years, Jiangsu Education Press House, Nanjing, China, 2004 (in Chinese).
Zhang, D. D., Jim, C. Y., Lin, G. C., He, Y. Q., Wang, J. J., and Lee, H. F.: Climatic change, wars and dynastic cycles in China over the last millennium, Climatic Change, 76, 459–477, https://doi.org/10.1007/s10584-005-9024-z, 2006.
Zhang, D. D., Lee, H. F., Wang, C. Li, B. Pei, Q. Zhang, J., and An, Y.: The causality analysis of climate change and large-scale human crisis, P. Natl. Acad. Sci. USA, 108, 17296–17301, https://doi.org/10.1073/pnas.1104268108, 2011.
Zhang, J. C. and Lin, Z. G.: Climate of China, Wiley, New York. Wiley, and Scientific and Technical Publishers, Shanghai, ISBN 0471519138, 1992.
Zheng, J., Xiao, L., Fang, X., Hao, Z., Ge, Q., and Li, B.: How climate change impacted the collapse of the Ming dynasty, Climatic Change, 127, 169–182, https://doi.org/10.1007/s10584-014-1244-7, 2014.
Zuo, M., Zhou, T., and Man, W.: Wetter global arid regions driven by volcanic eruptions, J. Geophys. Res.-Atmos., 124, 13648–13662, https://doi.org/10.1029/2019JD031171, 2019.
- Abstract
- Introduction
- Mid-17th-century eruptions in the ice core records
- Sources of mid-17th-century eruptions
- Tree-ring-based summer temperature reconstruction for the mid-17th century
- Evidence for dust veils and volcanic aerosols over Europe in 1641 and 1642
- Weather anomalies and societal distress in the late 1630s and early 1640s
- Climate, volcanic eruptions, and societal crisis: correlations exist, but is there also causality?
- Final considerations
- Data availability
- Author contributions
- Author contributions
- Disclaimer
- Special issue statement
- Acknowledgements
- Financial support
- Review statement
- References
- Supplement
- Abstract
- Introduction
- Mid-17th-century eruptions in the ice core records
- Sources of mid-17th-century eruptions
- Tree-ring-based summer temperature reconstruction for the mid-17th century
- Evidence for dust veils and volcanic aerosols over Europe in 1641 and 1642
- Weather anomalies and societal distress in the late 1630s and early 1640s
- Climate, volcanic eruptions, and societal crisis: correlations exist, but is there also causality?
- Final considerations
- Data availability
- Author contributions
- Author contributions
- Disclaimer
- Special issue statement
- Acknowledgements
- Financial support
- Review statement
- References
- Supplement