the Creative Commons Attribution 4.0 License.
the Creative Commons Attribution 4.0 License.
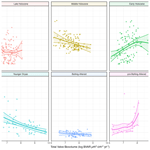
Long-term trends in diatom diversity and palaeoproductivity: a 16 000-year multidecadal record from Lake Baikal, southern Siberia
Vivian A. Felde
David W. Morley
Natalia Piotrowska
Patrick Rioual
Alistair W. R. Seddon
George E. A. Swann
Biological diversity is inextricably linked to community stability and ecosystem functioning, but our understanding of these relationships in freshwater ecosystems is largely based on short-term observational, experimental, and modelling approaches. Using a multidecadal diatom record for the past ca. 16 000 years from Lake Baikal, we investigate how diversity and palaeoproductivity have responded to climate change during periods of both rapid climate fluctuation and relative climate stability. We show dynamic changes in diatom communities during the past 16 000 years, with decadal shifts in species dominance punctuating millennial-scale seasonal trends. We describe for the first time in Lake Baikal a gradual shift from spring to autumnal diatom communities that started during the Younger Dryas and peaked during the Late Holocene, which likely represents orbitally driven ecosystem responses to long-term changes in seasonality. Using a multivariate classification tree, we show that trends in planktonic and tychoplanktonic diatoms broadly reflect both long-term climatic changes associated with the demise of Northern Hemisphere ice sheets and abrupt climatic changes associated with, for example, the Younger Dryas stadial. Indeed, diatom communities are most different before and after the boundary between the Early and Middle Holocene periods of ca. 8.2 cal kyr BP, associated with the presence and demise of Northern Hemisphere ice sheets respectively. Diatom richness and diversity, estimated using Hill's species numbers, are also shown to be very responsive to periods characterized by abrupt climate change, and using knowledge of diatom autecologies in Lake Baikal, diversity trends are interpreted in terms of resource availability. Using diatom biovolume accumulation rates (BVARs; µm3 cm−2 yr−1), we show that spring diatom crops dominate palaeoproductivity for nearly all of our record, apart from a short period during the Late Holocene, when autumnal productivity dominated between 1.8–1.4 cal kyr BP. Palaeoproductivity was especially unstable during the Younger Dryas, reaching peak rates of 18.3 × 103 µm3 cm−2 yr−1 at ca. 12.3 cal kyr BP. Generalized additive models (GAMs), which explore productivity–diversity relationships (PDRs) during pre-defined climate periods, reveal complex relationships. The strongest statistical evidence for GAMs were found during the Younger Dryas, the Early Holocene, and the Late Holocene, i.e. periods of rapid climate change. We account for these differences in terms of climate-mediated resource availability, and the ability of endemic diatom species in Lake Baikal to adapt to extreme forms of living in this unique ecosystem. Our analyses offer insight into how productivity–diversity relationships may develop in the future under a warming climate.
- Article
(3549 KB) - Full-text XML
- BibTeX
- EndNote
Understanding the role that biological diversity plays in ecosystem stability and function is an important challenge in ecological research (Tilman et al., 1997; McCann, 2000; Loreau et al., 2001; Isbell et al., 2015; Hagen et al., 2021). An ecosystem with higher biodiversity is assumed to be more stable, due to a number of factors, including the presence of species which have considerable plasticity, such that they have wide responses to disturbances (McCann, 2000; Luethje and Snyder, 2021), and the “insurance effect” (Yachi and Loreau, 1999), where species redundancy plays an important stabilizing role. Biodiversity loss can lead to reduced ecosystem functioning (Cardinale et al., 2012), which poses serious threats to ecosystem health in general, and human well-being in particular (e.g. Chivian, 2003). While the mechanisms behind how diversity controls functioning are not completely understood, dominant species with distinct functional roles are important (Winfree et al., 2015). For example, primary production, a key ecosystem function which helps regulate the global carbon cycle, is tied to species diversity (Tilman et al., 1997; Cardinale et al., 2009; Tilman et al., 2012). However, our understanding of productivity–diversity relationships (PDRs) is largely based on short-term observational (Dodson et al., 2000; Ptacnik et al., 2008; Korhonen et al., 2011), experimental (McGrady-Steed et al., 1997; Interlandi and Kilham, 2001; Winfree et al., 2015), and modelling approaches (Aoki, 2003), with very few long-term studies undertaken (Rusak et al., 2004). This constitutes an important gap in our knowledge because in terms of climate change, productivity–diversity relationships and resource use efficiency (Gross and Cardinale, 2007; Ptacnik et al., 2008) will be fundamentally different over long (e.g. climate and landscape evolution) and short (e.g. pulse disturbances such as climate disturbance events; Kéfi et al., 2019) timescales.
Palaeoecological approaches provide a potential solution to this challenge, since they can reveal ecological dynamics in response to environmental change which unfold only over very long timescales (National Research Council, 2005; Seddon et al., 2014). For example, diatoms are some of the most important primary producers in lake ecosystems, and their sub-fossilized remains provide a record of community variations through time. In addition, because the species composition, biovolume, and concentration of diatom valves can be directly measured on sediment sequences, there is a unique opportunity to investigate how community dynamics, diversity, and the stability of ecosystem functions such as productivity change over long-timescales. In particular, because the last 16 kyr have been punctuated by a series of large-scale and abrupt climatic shifts, such records open the door for an investigation into the links between biodiversity and the stability of ecosystem functioning associated with climate disturbance events.
Here we investigate the relationship between diatom diversity and ecosystem functioning in Lake Baikal, an ancient lake with a long continuous record with evidence of only very recent human perturbation (Izmest'eva et al., 2016; Roberts et al., 2018) restricted to its coastline (Kravtsova et al., 2014; Timoshkin et al., 2016). We focus on aquatic productivity as a measure of ecosystem function, because of the direct link between diatoms and primary production in the modern lake (Kozhova and Izmest'eva, 1998).
As yet we do not assume to know the precise nature of the potential productivity–diversity relationships that may have occurred in Lake Baikal over such long timescales. We do know, however, that local diversity will be influenced by a range of chemical, biological, and physical factors such as nutrient availability, species interactions, and disturbance events such as rapid climate change. Our approach therefore is to explore productivity–diversity relationships over specific timescales independently determined from palaeoclimate studies. We might hypothesize, for example, that productivity and diversity will co-vary linearly during rapid warming because increased energy results in increased metabolic rates in the system, but that this relationship might change during periods of relative climate stability. We investigate the range of possible response functions based on those identified by Smith (2007), whether they be negative or positive, whether they be humped or U-shaped, flat or just random (Smith, 2007).
One of the most relevant independent climate studies for this time period is by Tarasov et al. (2009), who modelled pollen-inferred palaeotemperature for neighbouring Lake Kotokel (Fig. 1) for the past 15 kyr. Their reconstructions indicate a period of rapid warming (during the Bølling–Allerød interstadial), rapid cooling (during the Younger Dryas stadial), and relative temperature stability of the Holocene in southern Siberia. However, given that the Holocene has recently been formally sub-divided into three ages or sub-epochs (Walker et al., 2018), it is possible to compare productivity–diversity relationships during the Younger Dryas and the Bølling–Allerød with the Greenlandian (Early), Northgrippian (Middle), and Meghalayan (Late) ages (sub-epochs) of the Holocene (henceforth referred to as Early, Middle, and Late Holocene). Our approach is to coax history to conduct experiments (Deevey, 1969) in order to deepen our understanding of (very) long-term biodiversity–ecosystem functioning (Loreau et al., 2001) through the exploration of relationships between diatom diversity and productivity, and to test hypotheses related to how rapid climate change may disrupt these relationships on sub-orbital timescales. We do this by
-
reconstructing trends in diatom communities, diatom diversity, and palaeoproductivity in Lake Baikal at a multidecadal resolution for the past ca. 16 kyr, and
-
hypothesizing that relationships between productivity and diversity will differ during periods of rapid climate change and periods of relative climate stability.
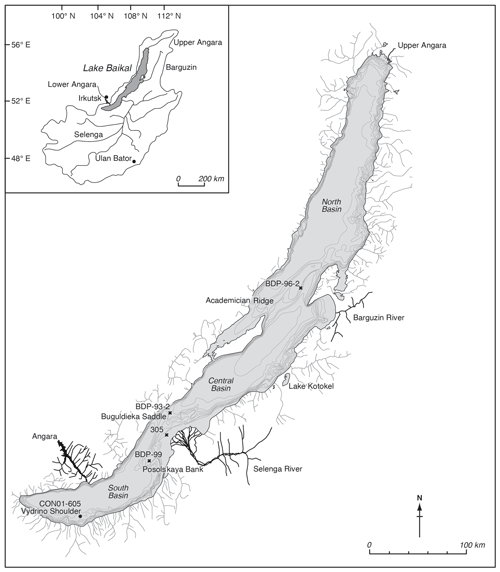
Figure 1Map of Lake Baikal and its catchment, with locations of the different cores used or mentioned in this paper, including the coring location on the Vydrino Shoulder, where our study was undertaken. Also shown is Lake Kotokel, which has provided independent climate reconstructions used in this study (Tarasov et al., 2009).
2.1 Study site
Lake Baikal is situated in southern Siberia at the forest–steppe ecotone (Fig. 1) and is the world's oldest, deepest, and most voluminous lake. The lake is a World Heritage Site, due to its diverse flora and fauna; it contains over 2500 species, of which over 75 % are thought to be endemic (Galazii, 1989). Its sediments have accumulated for over 25 million years, and decades of research have exploited this sedimentary record to reconstruct environmental change (Williams et al., 2001; Mackay, 2007). Sedimentary cores were obtained in 2001 from the research vessel Vereschagin, from the Vydrino Shoulder (51.585∘ N, 104.855∘ E; water depth 675 m), an underwater high (between 500–800 m) off the south-eastern coast of Lake Baikal (Fig. 1). The Vydrino Shoulder was selected because it is an area of stable, fine-grained sedimentation relatively undisturbed by tectonic activity and reworking (Charlet et al., 2005). The cores obtained included a 1.73 m trigger core (CON01–605–3a), a 10.45 m piston core (CON01–605–3), and a 2.50 m box core (CON01–605–5). These records were supplemented with a short gravity core taken from an ice platform in 2001 (CON01–105–6) from the same region.
2.2 Dating
Radiocarbon dates for the Vydrino sequence were obtained by accelerated mass spectrometry (AMS) from pollen and spore concentrates (Piotrowska et al., 2004; Demske et al., 2005). The age model is based on 12 AMS 14C pollen dates from the box core (CON01–605–5) (Piotrowska et al., 2004) and an additional five AMS 14C pollen dates from the piston core (CON01–605–3) (Demske et al., 2005). Full details are given in Mackay et al. (2011). Radiocarbon dates were calibrated using an IntCal20 radiocarbon calibration curve (Reimer et al., 2020), and age–depth modelling was done using “Bacon2.2” (Blaauw and Christen, 2011). No reservoir effect was applied to the calibration because the organic material used for dating, i.e. pollen and spore concentrates, is assumed to be uncontaminated by old carbon.
2.3 Diatom analysis
Diatoms amount to between 50 %–90 % of the phytoplankton biomass during spring bloom under ice and after ice break-up (Popovskaya et al., 2015; Panizzo et al., 2017). The spring bloom contributes a significant proportion of overall annual primary productivity (Popovskaya, 2000). With the onset of summer warming and surface water stratification, diatoms are succeeded by non-siliceous autotrophic picoplankton and other green algae (Fietz et al., 2005; Belykh et al., 2006). During autumn turnover, a smaller diatom bloom dominates primary production. Nitrogen and phosphorus co-limit photic zone productivity in Lake Baikal (Satoh et al., 2006; O'Donnell et al., 2017), with rates of deep-water nutrient supply increasing markedly since the mid-19th century (Swann et al., 2020).
Diatoms are siliceous, so they are generally well preserved in sedimentary environments. We prepared diatom samples for microscopy from sediments sampled every 5 mm from the composite sequence derived from the gravity, trigger, and piston cores detailed above. Unlike standard diatom preparation analyses (Battarbee et al., 2001), no chemical treatments were needed, although we enabled diatom concentrations to be calculated through the addition of divinylbenzene microspheres (Mackay et al., 1998). Diatom taxa were identified to species level or lower using a range of Russian and other floras, detailed in Mackay et al. (1998). With few exceptions, at least 300 valves from each sample were counted using oil immersion phase-contrast light microscopy at ×1000 magnification. Planktonic and tychoplanktonic diatoms account for on average ca. 90 % of all diatoms counted, and here we detail diatom compositional change for planktonic and tychoplanktonic species only, as these were used to determine palaeoproductivity estimates below. The diatom taxonomical nomenclature was updated according to the global online database AlgaeBase (https://www.algaebase.org/; last access: 2 November 2021).
Detrended correspondence analysis (DCA) and canonical correspondence analysis (CCA) were used to investigate the total amount of turnover, variation, and compositional changes of the diatom assemblages over time. The analyses were performed on Hellinger transformed data to dampen the effects of highly abundant taxa (Legendre and Gallagher, 2001). Multivariate classification tree (MCT) analysis was used to explore how much of the variation in diatom assemblages can be explained by the different climatic periods identified above. MCT is considered a powerful method when there is a non-linear relationship between response and explanatory variables, when there are missing values in the data, or when there are higher level interactions between explanatory variables (Borcard et al., 2018). The minimum-sized tree was chosen based on the 1 standard error rule, which is the minimum sized tree when the cross-validated relative error (CVRE) is the minimum CVRE value plus 1 standard error (SE) of all the CVRE values (Borcard et al., 2018). The number of multiple cross-validations was set to 100, and the number of k groups was set to the number of rows in the dataset. The counts were normalized prior to the analysis, and since MCT is in Euclidean space it transforms into the chord distances (Orlóci, 1967) between samples. Ordination analyses were done using R (R Core Team, 2021) and the R package vegan (Oksanen et al., 2020). The MCT was done using the R package mvpart (De'ath, 2014). Stratigraphical profiles were constructed using C2 Data Analysis Version 1.7.7 (Juggins, 2014).
2.4 Palaeoproductivity
The palaeoecological significance of diatom concentrations can be of limited value unless they can be converted into either diatom flux rates (e.g. Battarbee et al., 2001) or estimates of biovolume (Hillebrand et al., 1999). Diatom flux rates rely on a robust chronology (as determined in this study), while biovolume estimates require representative size measurements for the dominant taxa. We estimate palaeoproductivity through community biomass calculations derived from diatom biovolume accumulation rates (BVARs; µm3 cm−2 yr−1) for all major planktonic and tychoplanktonic species (Interlandi and Kilham, 2001). Biovolumes (µm3) were calculated using the median of measured linear dimensions of 25 valves and geometric shape guidelines (Hillebrand et al., 1999). Species used for biovolume calculations accounted for on average over 80 % of total relative abundance, and therefore we assume this is a robust estimate of palaeoproductivity.
2.5 Diatom richness, diversity, and evenness
Richness, diversity, and evenness were estimated using Hill's species numbers N0, N1, and N2 using diatom count data of the planktonic and tychoplanktonic flora only. We do not include benthic taxa because they represent a different habitat, far from the core location. Their persistent presence in the core (on average 10 %) is caused by secondary transport processes rather than species competing for the same resources as the planktonic diatoms themselves. Hill's species numbers give easily interpretable numbers by maintaining the doubling effect and provide information on three levels depending on how rare and abundant taxa are weighted in each sample (Hill, 1973; Jost, 2010; Gotelli and Ellison, 2013; Chao et al., 2014). Species richness is estimated using Hill's N0, the expected number of taxa where rare and abundant taxa have similar weights. Species diversity is estimated using Hill's N1, which is the expected number of equally common taxa, with less weight on rare taxa, and N2, which provides the expected number of equally abundant taxa but puts weight on the numerically dominant taxa. The estimates are represented as the expected number of diatoms based on the smallest sample size (n=150) to avoid biases related to different sample sizes. To take account of variable sediment accumulation rates (SARs), Hill's measures of richness and diversity were further divided by accumulation rates over time, so these measures become estimated Hill's numbers per cm2 yr−1. To detect variation in abundance changes over time (evenness) we also include the ratio of (Birks et al., 2016), i.e. the proportion of very abundant species to the number of common species identified. When the ratio is 1, it indicates that all taxa were equally abundant. The ratio is calculated based on the Hill's numbers after taking account of SAR.
2.6 Palaeoproductivity–diversity relationships
Relationships between palaeoproductivity and N2 diversity were investigated during pre-identified time intervals (Tarasov et al., 2009; Walker et al., 2018) to test the hypothesis that PDRs will differ during periods of rapid climate change and periods of relative climate stability. Defined climate periods are the pre-Bølling–Allerød (15.9–14.7 cal kyr BP), the Bølling–Allerød (14.7–12.9 cal kyr BP), the Younger Dryas (12.9–11.7 cal kyr BP), and the three recently designated sub-epochs of the Holocene (Early (11.7–8.2 cal kyr BP), Middle (8.2–4.2 cal kyr BP), and Late (4.2–0 cal kyr BP). We used generalized additive models (GAMs) to explore PDRs because we have no prior knowledge of the expected relationships, and because of the likelihood that the relationships were non-linear or showing different complex patterns within the different time periods. We used N2 diversity as the response variable and diatom BVAR as the explanatory variable. N2 is a continuous variable so we used a gamma distribution with a log link. Diatom BVAR as a variable was skewed so it was log-transformed prior to the analyses, and climatic period was included as a factor variable that allowed for different smoothings for each period. The smoothings were fitted using thin-plate regression splines, and the model was fitted using the restricted maximum likelihood approach. Prior to analyses, extreme outliers (BVARlog <6 µm3 cm−2 yr−1) were removed to reveal GAM relationships more clearly. This led to only 8 out of 521 samples being removed in total: three samples, two samples, and one sample removed from the Late, Middle, and Early Holocene sub-epochs respectively, and a further two from the pre-Bølling–Allerød period. Models were then refitted.
The model is specified as follows:
where i represents each sample, j is each climate period, and a1 is the intercept term for the periods. The term f1(Productivityi, Periodj) represents a factor-smooth interaction function in the GAM model fitted using thin-plate splines. Since the data are time ordered, the data points may not be independent, and we added a correlation term to the model attempting to reduce effects of potential temporal autocorrelation. However, adding different correlation structures did not improve the model or affect the residual variation, and we therefore removed them to keep the simplest model. The analysis was done using the mgcv R package (Wood, 2011).
3.1 Chronology
The calibrated ages for our profile span the last 15.91 cal kyr BP (where kyr = thousands of years, BP = before present, i.e. 1950 CE, common era) (Fig. 2). Sediment accumulation rates ranged between 32–184 yr cm−1 (mean 62 yr cm−1).
3.2 Diatoms
The temporal resolution of species compositional change was ca. 30 years for the past ca. 16 kyr (Fig. 3). DCA axis 1 sample scores show a strong, largely unidirectional trend since the start of the Holocene. Turnover in the planktonic and tychoplanktonic assemblages over the whole time period is 2.71 standard deviation units, reflecting the observation that several species present during the early part of our record are also present during the latter part of our record. The total inertia (variation) measured by CCA is 1.82, and of this the constraining time variable explains 10.6 % of the variation (eigenvalue = 0.193). The ratio of eigenvalues of the constrained axis 1 over the unconstrained axis 2 is 1.29 indicating that the time gradient is explaining an important component of the variation.
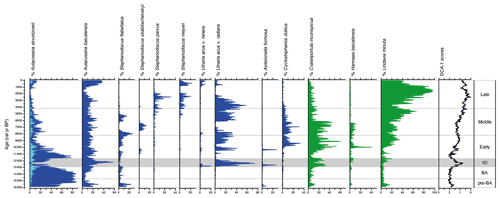
Figure 3Relative abundances of sub-fossil planktonic and tychoplanktonic diatoms spanning the past ca. 16 kyr from Vydrino Shoulder. Diatoms which grow mainly before summer stratification are shown in blue, while those that mainly grow after summer stratification are shown in green. DCA axis 1 sample scores summarize the major trend in species turnover.
During the early stages of the pre-Bølling–Allerød period, N0 and N2 values were initially relatively high (Fig. 4), before declining as the tychoplanktonic Aulacoseira skvortzowii dominated the assemblage (Fig. 3). Dominance by A. skvortzowii persists into the Bølling but is gradually reduced by increasing abundances of the planktonic Aulacoseira baicalensis during the later Allerød. DCA axis 1 ordination scores show very little change during the pre-Bølling–Allerød and Bølling–Allerød interstadial, indicative of a rather stable assemblage, with few equally abundant species reflected in low evenness scores (). MCT is not able to discriminate well between diatom assemblages of the pre-Bølling–Allerød and Bølling–Allerød interstadial (Fig. 5).
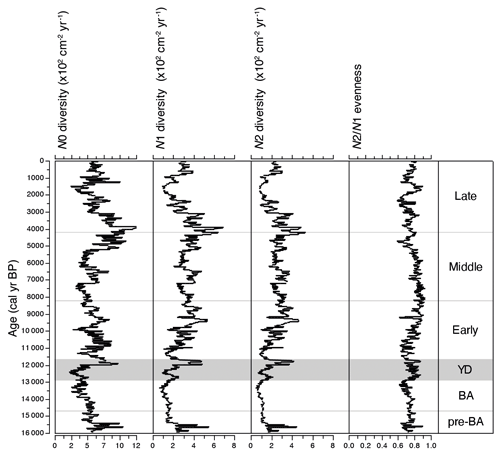
Figure 4Comparison of Hill's richness (N0), diversity (N1 and N2), and evenness ( ratio) trends over the past ca. 16 kyr. Richness and diversity values were further divided by sediment accumulation rates over time, so these measures become estimated Hill's numbers per cm−2 yr−1.
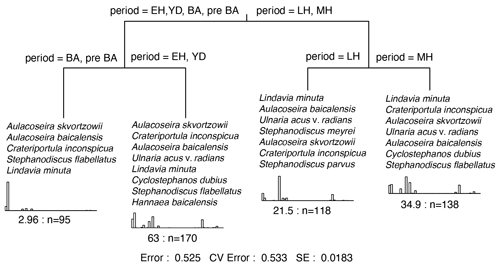
Figure 5Multivariate classification tree (MCT) for Lake Baikal planktonic and tychoplanktonic diatoms over the past ca. 16 kyr. Time periods used in multivariate classification tree analyses are the pre-Bølling–Allerød (pre-BA); the Bølling–Allerød (BA) interstadial; the Younger Dryas stadial; and the three recently ratified Early, Middle, and Late Holocene sub-epochs (Walker et al., 2018). The histograms show the transformed abundances of the discriminating taxa within each leaf. These are listed in decreasing order. The numbers under the histograms represent the relative error within each leaf, and n= the total number of samples. At the bottom is the summary of the residual error (RE), cross-validated error (CVRE), and standard error (SE). The r2 will be 1-RE.
The Younger Dryas stadial is characterized by major changes in the diatom assemblage – abundances of Aulacoseira (especially A. skvortzowii) decline, concomitant with a rapid succession of Ulnaria acus and Crateriportula inconspicua, that bloom in spring and autumn respectively (Fig. 3). These taxa give way to a short-lived peak in A. baicalensis dominance, before A. skvortzowii once again asserts its dominance by the end of the Younger Dryas, alongside notable increases in C. inconspicua and Lindavia minuta, resulting in increasing richness (N0) and diversity (N2) scores (Fig. 4). These changes are reflected in a rapid change in DCA axis 1 scores at the start of the Younger Dryas, with discernible changes in the evenness ratio indicative of the increasing importance of different species. The Early Holocene is characterized in the main by declining dominance of A. skvortzowii and increasing importance of autumn-blooming crops of C. inconspicua and Lindavia minuta. U. acus shows considerable fluctuations, with Hannaea baicalensis and Cyclostephanos dubius increasing in importance from ca. 10 cal kyr BP, driving increases in diatom N2 diversity and the evenness ratio.
Where the climatic periods are used as a supervised classification technique, MCT analysis reveals the highest variation between the Early and the Middle Holocene sub-epochs (Fig. 5). This transition also marks the time when the evenness ratio peaks and then declines to its lowest values found in our study during the Middle and Late Holocene (Fig. 4). During the Middle Holocene, several diatom species largely disappear from the stratigraphical record including Stephanodiscus flabellatus, Stephanodiscus skabitchevskii, H. baicalensis, and C. dubius, while C. inconspicua drops to low values for the remainder of the record (Fig. 3). In their place, U. acus and L. minuta increase in importance. The loss and gain of species through the Middle Holocene has resulted in a relatively high but stable N2 diversity, with peak diversity occurring at the boundary between the Middle and Late Holocene, ca. 4.2 cal kyr BP (Fig. 4). Despite relatively high abundances for the first time of Stephanodiscus parvus and Stephanodiscus meyeri, the Late Holocene sees L. minuta increasing to almost complete dominance of the diatom assemblage between 1.8–1.4 cal kyr BP, resulting in some of the lowest diatom diversity values for the study. During the most recent 500 years of our record, the assemblage is characterized by species commonly found in the modern–day lake communities, co-dominated by A. baicalensis, U. acus, and L. minuta (Fig. 3).
3.3 Palaeoproductivity
Diatom biovolume accumulate rate (BVAR) data show that spring diatom crops have dominated palaeoproductivity for much of the past ca. 16 kyr, apart from a short period during the late Holocene, 1.8–1.4 cal kyr BP, when autumnal diatom production increases to more than 10 times that of spring production (Fig. 6). BVARs were substantially higher and more unstable during the Younger Dryas stadial than the warm periods before and after it (Fig. 6). Peak palaeoproductivity for the whole study (18.3 × 103 µm3 cm−2 yr−1) was associated with successive peaks in U. acus and then A. baicalensis at ca. 12.3 cal kyr BP. BVAR then declined rapidly and remained relatively low for the remainder of the Younger Dryas and the start of the Holocene. Palaeoproductivity increased during the Early and Middle Holocene, before declining to its lowest rates during the Late Holocene (mean, 1.3 × 103 µm3 cm−2 yr−1).
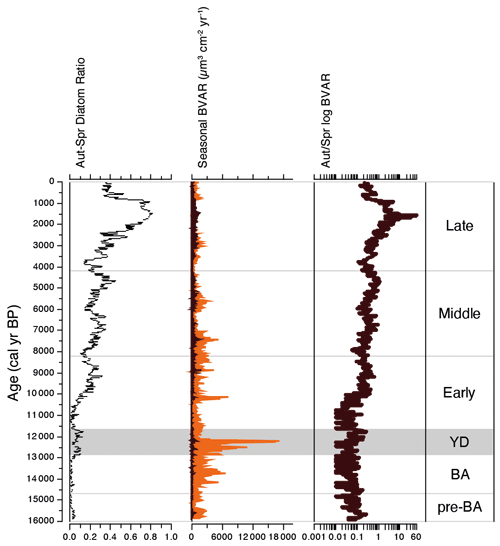
Figure 6Stratigraphical profile showing trends in palaeoproductivity over the past ca. 16 kyr: the proportion of diatoms that bloom in the autumn versus those that bloom in the spring; estimates of palaeoproductivity derived from spring (orange) and autumn (dark brown) diatom biovolume accumulate rates (BVAR µm3 cm−2 yr−1); and log-transformed ratio of autumnal/spring palaeoproductivity.
The shapes of the productivity–diversity relationships differ among the pre-defined climate periods, with some being negative (e.g. the Younger Dryas and the Middle Holocene periods), positive (e.g. the pre-Bølling–Allerød and the Early Holocene periods), and others more complex such as the Late Holocene, dependent on palaeoproductivity rates (Fig. 7). The relationship between palaeoproductivity and diversity during the Bølling–Allerød was flat (Smith, 2007). GAMs with very low p values suggest that we have higher confidence that PDRs existed during the Younger Dryas, the Early Holocene, and the Late Holocene in comparison to the other time periods (Table 1).
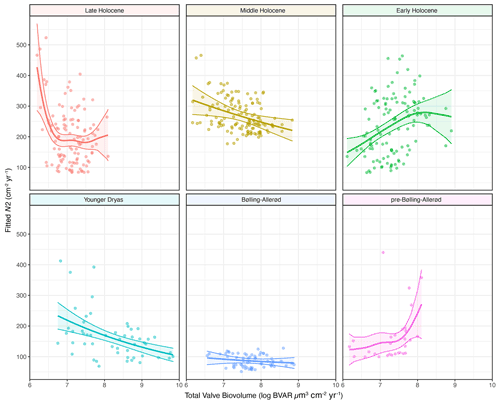
Figure 7Palaeoproductivity–diversity relationships explored using generalized additive models (GAMs) that allow different smoothings for the pre-defined climatic periods: pre-Bølling–Allerød, (Bølling–Allerød), Younger Dryas, Early Holocene, Middle Holocene, and Late Holocene. The dots represent the data points, the thick line is the fitted GAM response for each period, and the shaded areas represent the 95 % confidence intervals around the mean fitted response.
Table 1GAMs to investigate PDR trends during pre-defined climate periods. edf = effective degrees of freedom, and ref.df = reference degrees of freedom. The goodness-of-fit statistics show the adjusted r2=0.462, and the deviance explained is 53.2 %.
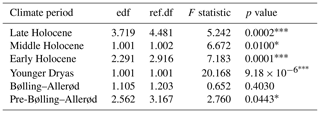
Significance codes: 0–0.001. 0.001–0.01. * 0.01–0.05. Where there is no asterisk, the significance code is between 0.05 and 1.
Over the past 16 kyr diatom trends in Lake Baikal have been dynamic, with decadal shifts in species dominance punctuating millennial-scale trends in seasonal communities. MCT analyses show that the main split in diatom composition occurs between assemblages associated with the Last Glacial–Interglacial Transition (LGIT; 16–8.2 cal kyr BP) and the Middle–Late–Holocene sub-epochs (8.2 cal kyr BP–present) (Fig. 5). This suggests that trends in community shifts in diatoms in Lake Baikal broadly reflect climatic changes associated with the demise of Northern Hemisphere ice sheets as the planet transgressed from glacial to interglacial environments (Denton et al., 2010). Here we focus on interpreting trends in diatom communities and diversity through time, before focussing on how productivity–diversity relationships (PDRs) vary over long timescales, including during periods of rapid climate change and periods of relative climate stability.
4.1 Last Glacial–Interglacial Transition
Due to high obliquity (Fig. 8j), the LGIT in the Lake Baikal region was intensely seasonal, resulting in very cold winters but relatively warm, moist summers (Bush, 2005). Cold winters during the late glacial resulted in substantially longer annual ice cover on Lake Baikal of up to 8–9 months, reduced hydrological input by as much as 40 % than the modern day, and restricted inputs of key nutrients such as P, N, and Si (Shimaraev and Mizandrontsev, 2006). Up to the Bølling–Allerød, diatom assemblages were dominated by spores of the endemic Aulacoseira skvortzowii (Fig. 3). A. skvortzowii is tychoplanktonic, having evolved a life cycle which utilizes both pelagic and littoral habitats; viable spores remain in coastal sediments down to a water depth of 25 m, where they can be resuspended by strong autumn storms back into the pelagic zone (Jewson et al., 2008) in time to bloom the following spring. A. skvortzowii grows best in water temperatures below 5 ∘C, and to avoid lethal increasing surface water temperatures, spore formation is triggered after ice break-up when phosphate concentrations fall below a threshold level of 15–23 µg L−1 (Jewson et al., 2008). Declining phosphate concentrations are caused by P uptake by other algae (e.g. green algae and picoplankton) as surface waters start to stratify. Pigment analyses have shown that green algae were abundant at this time in the south basin of Lake Baikal (Tani et al., 2002; Soma et al., 2007). Relatively low N2 plankton diversity and evenness values during much of the late glacial likely reflect P and Si limitation, resulting from overall lower nutrient concentrations due to the colder climate (Shimaraev and Mizandrontsev, 2006) but possibly also competition from other algae.
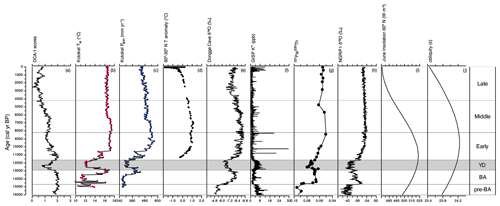
Figure 8Multi-archive data plotted alongside (a) DCA axis 1 sample scores of Lake Baikal diatoms, as a measure of turnover over the past ca. 16 kyr; (b) mean pollen–inferred annual precipitation from Lake Kotokel, with smooth line representing the mean three-point moving average (Tarasov et al., 2009); (c) mean pollen–inferred temperature of the warmest month from Lake Kotokel, with the smooth line representing the mean three-point moving average (Tarasov et al., 2009); (d) mean Northern Hemisphere temperature stack records for 60∘ latitude bands (30–90∘ N; Marcott et al., 2013); (e) δ18O values of Dongge Cave stalagmite D4 (Dykoski et al., 2005); (f) K+ ion concentrations (ppb) from GISP2 D core (Mayewski et al., 1997); (g) North Atlantic core GGC5 meridional circulation data; (h) δ18O values of NGRIP ice core (Rasmussen et al., 2006); (i) June insolation 60∘ N (W m−2; Berger and Loutre, 1991); (j) obliquity (ε).
The major shift to warmer temperatures during the LGIT occurred with the onset of the Bølling–Allerød interstadial, ca. 14.7 cal kyr BP, linked to heat release from warm waters deep in the North Atlantic Ocean (Thiagarajan et al., 2014) which led to the resumption of the Atlantic meridional overturning circulation (AMOC) (McManus et al., 2004) (Fig. 8g). Mean pollen-inferred temperatures of the warmest month from neighbouring Lake Kotokel however (Fig. 8b), reveal a 5 ∘C increase only during the latter stages of the Bølling–Allerød (Tarasov et al., 2009). At the same time, pollen-inferred mean annual precipitation records show that rainfall increased from ca. 300 to ca. 460 mm yr−1 (Fig. 8c). This warmer, wetter Allerød led to extensive melting of glaciers along the shores of Lake Baikal (Horiuchi et al., 2004; Osipov and Khlystov, 2010), major expansion of boreal vegetation (Tarasov et al., 2009; Bezrukova et al., 2010; Reshetova et al., 2013), reduced ice duration on the lake, and increased nutrient transport (Shimaraev and Mizandrontsev, 2006). The impacts on diatom communities, however, were subtle, with diatom composition between the pre-Bølling–Allerød and Bølling–Allerød not distinguishable through MCT analyses (Fig. 5). Diversity and evenness show little variability, although relative abundances of a few species show distinct changes. For example, the heavily silicified endemic Aulacoseira baicalensis will have benefited from increased availability of dissolved silica (Jewson et al., 2010), while the endemic Stephanodiscus flabellatus may have benefited from elevated concentrations of phosphorus (Bradbury et al., 1994). The observed decline in A. skvortzowii spore formation may also be attributable to generally increased P availability in the south basin (Jewson et al., 2008).
The Younger Dryas stadial (12.9–11.7 cal kyr BP) is probably the most studied climate disturbance event of the late Quaternary (Broecker et al., 2010). It was caused by an influx of freshwater from Lake Agassiz into the Arctic Ocean (Tarasov and Peltier, 2005; Murton et al., 2010) which led to the decline in AMOC (Fig. 8g) (Bond et al., 2001; McManus et al., 2004), and concomitant cooler temperatures across the Northern Hemisphere (Stuiver et al., 1995) (Fig. 8h). Increased GISP2 K+ concentrations (Fig. 8f) indicate that the Siberian High intensified at the start of the Younger Dryas (Mayewski et al., 1997), which accounts for both an attenuated Asian summer monsoon (Fig. 8e) (Dykoski et al., 2005) and cooler and more arid climate conditions in the Lake Baikal region (Tarasov et al., 2009) (Fig. 8b, c). These cooler, more arid conditions led to a decline in hydrological input into Lake Baikal itself (Mackay et al., 2011) and a short-lived expansion of the tundra biome (Tarasov et al., 2009; Bezrukova et al., 2010).
Diatom responses to climate change within the Younger Dryas were instantaneous but complex, kick-started by the first appearance and rapid growth of Ulnaria acus (Fig. 3) (indicative of higher dissolved silica concentrations in the water column; Bradbury et al., 1994) and increasing abundance of C. inconspicua. However, the decline in N2 diversity only from ca. 12.6 cal kyr BP (Fig. 4) suggests that resources for diatom growth were not initially limiting. Occupying different seasonal niches, spring-blooming U. acus and autumnal-blooming C. inconspicua (Ryves et al., 2003) are unlikely to be directly competing for the same resources, in part because small centric diatoms do not utilize a lot of dissolved silica (Bradbury et al., 1994). Having several co-dominant species is reflected in the relatively high evenness scores for the Younger Dryas (Fig. 4), related to few resources being limiting (Interlandi and Kilham, 2001).
The decline in N2 diversity is linked to peak values for A. baicalensis (Fig. 3), competing directly with U. acus for dissolved silica. Diatom population changes in Lake Baikal play an important role in controlling availability of silicic acid for other species (Callander and Granina, 1995; Jewson et al., 2010; Shimaraev and Domysheva, 2013; Jewson et al., 2015). In particular, when populations of A. baicalensis are very high, the availability of silicic acid uptake for other species declines, leading to a drop in their populations (Jewson et al., 2010, 2015). Being a heavily silicified species, A. baicalensis needs a strong period of mixing (Huisman et al., 2004), which suggests that when abundant, turnover is strong, allowing the recycling of nutrients needed for peak palaeoproductivity (Fig. 6). Our diatom records demonstrate that species specially adapted to grow under the ice of Lake Baikal (Bondarenko et al., 2012) have flourished alongside autumnal-blooming species during this cold stadial, with, initially at least, few limiting resources.
The latter stages of the Younger Dryas are characterized by a marked increase in diatom richness and diversity associated with both increases in spring and autumn diatoms and a rapid decline in palaeoproductivity. Given that regional temperatures remain low and unchanged at this time (Tarasov et al., 2009), internal dynamics in the lake may be driving observed changes in diatom communities, mediating resource availability. Contemporary monitoring studies have shown that populations of A. baicalensis can undergo dramatic changes in population size linked to complex interactions including silica availability (Izmest'eva et al., 2006); after populations peak, cells are transported to the bottom sediments, taking with them silica that then becomes unavailable for new diatom growth (Jewson and Granin, 2015). But autumnal diatoms are still able to grow, as nutrients become available once more during autumnal turnover.
As detailed above, MCT analyses is unable to distinguish Younger Dryas and Early Holocene diatom communities (Fig. 5), two time periods characterized by significant millennial-scale variability (Bond et al., 2001; Fisher et al., 2002; Mayewski et al., 2004; Nesje et al., 2005; Wanner et al., 2014). Overall, the diatom flora is characterized by persistent spring-blooming species that need lots of dissolved silica, but also increasing abundance of autumnal blooming diatoms. A decline in species richness and diversity at the start of the Early Holocene may be indicative of fewer resources (Interlandi and Kilham, 2001), coincident at least with the widely expressed cool Preboreal Oscillation (Fisher et al., 2002) and lower river flow into Baikal's south basin (Mackay et al., 2011). After ca. 11.3 cal kyr BP diatom diversity and evenness increase for the next few thousand years, indicative of increasingly abundant resources, linked to warmer and wetter regional climates (Tarasov et al., 2009) and generally stronger seasonality. Co-limitation by several nutrients and light allow for greater numbers of planktonic species to co-exist (Burson et al., 2018), leading to the highest evenness scores at the Early–Middle Holocene boundary.
4.2 Middle–Late Holocene
Northern Hemisphere cooling, especially from ca. 5 cal kyr BP, is linked to declining summer insolation (Marcott et al., 2013) in conjunction with vegetation and snow–ice feedbacks on Earth's surface albedo. This cooling culminated in the neoglacial, which in central Asia resulted in increased aridity (Ganopolski et al., 1998) expressed in the Lake Baikal region as declining mean annual precipitation (Tarasov et al., 2009). The neoglacial also resulted in increased Siberian permafrost formation (Anisimov et al., 2002). Within Lake Baikal, we document a shift from spring to autumnal diatom communities that started during the Early Holocene, concomitant with the first decline in insolation, but then continues to get stronger during the Middle and Late Holocene as both precession and obliquity decline (Fig. 8). The slow unfolding of an increasingly important autumnal diatom community has relatively little impact on palaeoproductivity during much of the Holocene, although autumnal production after 10 cal kyr BP is slightly higher than it was before 10 cal kyr BP and continues to proportionally increase especially during the Late Holocene. This long-term change in diversity and palaeoproductivity likely represents an orbitally driven ecosystem response to long-term climate change, possibly linked to changes in seasonality and its impact on, for example, the ice cover dynamics and length of summer stratification in the lake.
L. minuta is currently the only pelagic diatom to bloom in substantial numbers during the autumn in Lake Baikal (although it can also grow in smaller numbers during spring turnover). Because L. minuta can tolerate surface water temperatures up to 11 ∘C, cells persist higher in the surface waters for longer during summer stratification, so that when stratification breaks down in the autumn and nutrients are regenerated, cells of L. minuta are retrained into the photic zone first, giving them a strong competitive advantage, leading to their dominance during autumn turnover (Jewson et al., 2015). Winter ice cover is a major force driving inter-seasonal connections in lakes that freeze over every year (Sommer et al., 2012; O'Reilly et al., 2015; Hampton et al., 2017), and Lake Baikal is no exception. The gradual decline in the proportion of spring diatoms may have resulted in increased resource availability for other diatoms (Interlandi and Kilham, 2001; Jewson et al., 2010, 2015). For example, monitoring studies have shown large autumnal populations of L. minuta during the 1950s CE when spring populations of A. baicalensis were very low (Antipova and Kozhov in Kozhov, 1963).
The build-up to peak dominance in L. minuta during the Late Holocene is accompanied by the successive decline in spring-blooming species, starting with U. acus, followed by A. baicalensis, species that both rely on the availability of dissolved silica (Jewson et al., 2010) and under-ice turbulence to remain in the photic zone (Granin et al., 2000). These shifts are reflected in rapidly declining N2 diversity scores since their peak at the Middle–Late Holocene boundary to their lowest values for the Holocene (Fig. 4). This decline is also coincident with a major shift in carbon dynamics in Lake Baikal, linked to increasing regional cooling and aridity (Mackay et al., 2017). The almost monospecific Late Holocene assemblage of L. minuta and resultant low N2 diatom diversity in the lake are concurrent with the Dark Ages Cold Period (DCAP) (1.8–1.4 cal kyr BP; CE 400–765) (Helama et al., 2017), a climatically cool period, linked to changes in ocean circulation (Bond et al., 2001) and solar and volcanic activity (Helama et al., 2017). The near-complete disappearance of A. baicalensis makes this period unique in our Lake Baikal record. However, we are not yet able to say conclusively why growth of A. baicalensis was so inhibited during the DCAP, leading to the dominance of L. minuta for several centuries. This period almost certainly represents an ecosystem response to abrupt extrinsic change occurring elsewhere in the Northern Hemisphere, super-imposed on longer-term changes in orbital parameters (Williams et al., 2011). It is possible that the smaller biovolume of L. minuta in comparison to A. baicalensis and U. acus may have conferred to it a competitive advantage as resources became increasingly limited (Burson et al., 2018). Alternatively, persistent deeper snow cover on the frozen lake could have acted to reduce light penetration through the ice, (i.e. light as a resource is severely limited) resulting in a decline in sub-surface turbulence and loss of spring diatom crops to deeper waters (Granin et al., 2000). It is possible that these responses are linked to orbitally driven seasonal changes such as cooler summers (Fig. 8). It is notable that the ecosystem function of primary production did not decline overall during this period, just the timing of peak production, with autumnal palaeoproductivity far exceeding spring productivity for the only time in our record during the DCAP (Fig. 6).
4.3 Palaeoproductivity–diversity relationships
Productivity–diversity relationships (PDRs) in aquatic ecosystems are complex (Aoki, 2003; Smith, 2007), while also being scale-dependent (Chase and Leibold 2002; Korhonen et al., 2011) and time-dependent (Rusak et al., 2004). Our initial hypotheses set out to test if these relationships differ between periods of relative climate stability and periods of rapid climate change. This is borne out, as the shapes of the relationships are very different across the different climate periods (Fig. 7). The strongest evidence for GAMs (with the lowest p values; Table 1) were found during both the Younger Dryas and Early Holocene, periods punctuated by pervasive millennial-scale variability, and the Late Holocene period, characterized by cold events linked to overlapping drivers such as solar minima and volcanic eruptions (Helama et al., 2017), amplified by centennial-scale oceanic variability (Renssen et al., 2006). PDRs were different during each of the pre-Bølling–Allerød, the Bølling–Allerød and the Middle Holocene periods, but statistical support for GAMs was much weaker during these periods of relative climate stability (Table 1). During the Bølling–Allerød the PDR is effectively flat (Smith, 2007), because N2 diversity is restricted to very low values and changes little across the magnitude of the productivity gradient, perhaps due to as yet few available resources for several species to co-exist. During the Middle Holocene, N2 diversity values are again more restricted than during either the Early or Late Holocene periods, and N2 are rarely low (Fig. 7), likely linked to optimal resource availability allowing several species to co-exist (Burson et al., 2018) at similar abundances (Fig. 4) during both spring and autumn (Fig. 3).
The negative PDR during the Younger Dryas likely reflects a complex set of processes linked to both ecosystem responses to abrupt cooling (e.g. increased ice duration but stronger turnover in the lake; Shimaraev and Mizandrontsev, 2006) and a reduction in resource heterogeneity due to severe limitation by a few resources or even a single resource (Interlandi and Kilham, 2001). Productivity in Lake Baikal is dependent on a sustained supply of nutrients from deeper waters to the photic zone, stimulated by shifting wind dynamics and enhanced deep ventilation (Swann et al., 2020). While an increased supply of Si ensured initial resources for Ulnaria and Aulacoseira growth, Si will ultimately have become unavailable to most other diatoms, due to massive increases in the abundances of A. baicalensis (Fig. 3) (Jewson et al., 2010, 2015), leading to declines in diversity while productivity peaked. During the Early Holocene, however, there is a positive PDR, especially when palaeoproductivity levels are initially lower. We suggest that rapid melting of glaciers and increased fluvial input (Osipov and Khlystov, 2010), as well as opening up of new habitats as ice-cover duration on the lake declined, contributed to an increased supply of nutrients and habitat availability, such that both diversity and productivity were able to increase from the very low values at the start of the Holocene period. It is notable that during the Early Holocene the ratio of autumnal to spring species increases markedly, indicative of autumnal turnover niches opening up, allowing increased growth of C. inconspicua and L. minuta (Fig. 3).
During the Late Holocene, however, a negative PDR is apparent only when palaeoproductivity is very low at the transition between the Middle and Late Holocene periods (Fig. 7). Low palaeoproductivity at ca. 4.2 cal kyr BP may be linked to a period of regional cooling and aridity (Mackay et al., 2017) concurrent with weaker Asian summer monsoons (Dixit et al., 2014). It is at this time that new Stephanodiscus species become established in the lake (S. meyeri and S. parvus) alongside existing diatom communities, hence higher N2 diversity. Stephanodiscus species tend to reflect higher phosphorus than silicon loading rates (Kilham et al., 1986) and may be a community response to a cooler, more arid climate (Bradbury et al., 1994) as experienced during the Late Holocene neoglacial. However, for the majority of the Late Holocene there is little relationship between palaeoproductivity and diversity, indicative of abundant resources allowing for many planktonic species to co-exist.
4.4 Comparisons to other studies within Lake Baikal
It has long been recognized that Quaternary biogenic silica and diatom concentrations in Lake Baikal sediments mirror changes in insolation (Khursevich et al., 2001), such that very low concentrations characterize glacial periods, likely due to a number of factors including lower productivity and higher rates of diatom dissolution, as well as dilution due to increased inputs of clastic material (Mackay, 2007). The Vydrino LGIT record has an almost identical diatom assemblage to those identified for the same time period in long cores extracted from elsewhere in the lake, including both the Posolskaya Bank (BDP-99) and Academician Ridge (BDP–96–2) (Khursevich et al., 2005) (Fig. 1). In another study of Quaternary Lake Baikal diatoms, this time from the Buguldieka Saddle (Fig. 1), Edlund (2006) found that although earlier glaciations also contained few diatoms, the “Sartan glaciation”, i.e. Marine Isotope Stage 2, still contained at least 10 species of planktonic diatoms, and an assemblage again very similar to our Vydrino sequence. Bradbury et al. (1994) produced a similar but much lower resolution record for diatom changes spanning the past 15 kyr from station 305 off the southern margins of the Selenga Delta (Fig. 1), where both the assemblage and sequence of diatoms are similar to Vydrino. However, while observations and conclusions drawn in this study related to PDRs are likely applicable to other regions of this vast lake, one of the reasons why Lake Baikal contains a diverse endemic diatom flora is down to its size and heterogeneity of habitats (Jewson et al., 2015). Thus while species declines may be evident in one region, those same species may well persist in other regions of the lake.
This study provides important insights into our understanding of productivity–diversity relationships (PDRs) in aquatic ecosystems. We show that diatom communities and palaeoproductivity are sensitive to extrinsic drivers of climate change. These drivers operate at different timescales, from abrupt events during the Younger Dryas resulting in (negative) coupling between palaeoproductivity and diversity, to slower changes in boreal insolation and seasonality, leading to varied PDRs. These differences are likely related to resource availability and limitation (or not) of specific nutrients, linked to climate and surface water turnover. We therefore provide an important perspective to complement existing short-term observational (Dodson et al., 2000) and experimental (Winfree et al., 2015) approaches. PDRs in aquatic systems should not be expected to occur in one direction (Smith, 2007) but are very much dependent on other factors such as external disturbances, resource availability, species interactions and abiotic constraints on ecosystem function. Even given the sheer volume of Lake Baikal, diatom responses to abrupt events can be almost instantaneous, showing how tightly coupled ecology and climate have been in the past.
Over sub-orbital timescales, our records suggest that ecosystem function in Lake Baikal is rather resilient to natural disturbance. Following the concept of operation criteria as defined by Jovanovska et al. (2016), after disturbance or “press” events like the Younger Dryas, diatom communities and palaeoproductivity return to pre-disturbance states. Moreover, rather than leading to the demise of certain species, new species are actually ushered into the palaeorecord, likely due to increased resources (Fig. 3). And even when a regime shift looks possible with respect to the increasing dominance of an autumnal flora as the Holocene unfolded, in the past 1000 years a spring-dominated flora has returned. These observations may hold insights into observed diversity changes occurring in recent decades, linked to global warming (Roberts et al., 2018). However, what our record cannot provide information on is the resilience of the lake's flora to multiple stressors such as human-driven climate change and anthropogenic nutrient enrichment, which is currently impacting the lake's littoral communities.
R code to calculate diatom diversity and investigate the diversity–palaeoproductivity relationships can be accessed and downloaded from the GitHub repository: https://github.com/Vivian-Felde/Diatom_diversity_Vydrino_Lake_Baikal/tree/v.1.0.0 (last access: 3 February 2022) (public release 3 February 2022) (https://doi.org/10.5281/zenodo.6124051, Felde, 2022).
The planktonic and tychoplanktonic diatom counts used in this study are available for download from NOAA's National Centers for Environmental Information https://doi.org/10.25921/q6v1-5q02 (Mackay et al., 2022).
The study was conceived by AWM and PR. Diatom counting was undertaken by DWM. Age modelling was done by NP. Data analysis was undertaken by VAF, AWRS, and AWM. AWM led the paper writing with significant contributions from AWRS and GEAS. All authors made comments on earlier drafts of the paper.
The contact author has declared that neither they nor their co-authors have any competing interests.
Publisher’s note: Copernicus Publications remains neutral with regard to jurisdictional claims in published maps and institutional affiliations.
We thank the UCL Geography Cartography Unit who helped prepare the figures. And we especially thank the two reviewers and Gavin Simpson whose observations and comments have helped to improve the focus of the study immensely. Anson W. Mackay would like to say a special thanks to the editorial team of CP for guiding the study's publication over an extended period of time due to illness. The flexibility and understanding shown should be a model for other journals to follow.
This research has been supported by EU Fifth Framework Programme for Research and Technological Development (grant no. EVK2-CT-2000-0057), UK Natural Environment Research Council (grant no. NE/J010227/1), and the Norwegian Research Council (IGNEX grant no. 249894/F20).
This paper was edited by Keely Mills and reviewed by two anonymous referees.
Anisimov, O. A., Velichko, A. A., Demchenko, P. F., Eliseev, A.V., Mokhov, I. I., and Nechaev, V.P.: Effect of climate change on permafrost in the past, present, and future, Izvestiya Atmospheric and Ocean Physics, 38, 25–39, 2002.
Aoki, I.: Diversity–productivity–stability relationship in freshwater ecosystems: Whole-systemic view of all trophic levels, Ecol. Res., 18, 397–404, 2003.
Battarbee, R. W., Jones, V. J., Flower, R. J., Cameron, N.J ., Bennion, H., Carvalho, L., and Juggins, S.: Diatoms, in: Tracking environmental change using lake sediments Vol. 3: Terrestrial, Algal, and Siliceous Indicators, edited by: Smol, J. P., Birks, H. J., and Last, W. M., Springer, Dordrecht, 155–202, ISBN 978-90-481-6048-8, 2001.
Belykh, O. I., Ekaterina, G., Sorokovikova, T., Saphonova, I. A., and Tikhonova, V: Autotrophic picoplankton of Lake Baikal: Composition, abundance and structure, Hydrobiologia, 568, 9–17, 2006.
Berger, A. and Loutre, M. F.: Insolation values for the climate of the past 10 million years, Quaternary Sci. Rev., 10, 297–317, 1991.
Bezrukova, E. V., Tarasov, P. E., Solovieva, N., Krivonogov, S. K., and Riedel, F.: Last glacial–interglacial vegetation and environmental dynamics in southern Siberia: Chronology, forcing and feedbacks, Palaeogeogr. Palaeocl., 296, 185–198, 2010.
Birks H. J. B., Felde V. A., Bjune A. E., Grytnes J.-A., Seppä H., and Giesecke, T.: Does pollen-assemblages richness reflect floristic richness? A review of recent developments and future challenges, Rev. Palaeobot. Palynol., 228, 1–25, https://doi.org/10.1016/j.revpalbo.2015.12.011, 2016.
Blaauw, M. and Christen, J. A.: Flexible paleoclimate age-depth models using an autoregressive gamma process, Bayesian Anal., 6, 457–474, 2011.
Bond, G., Kromer, B., Beer, J., Muscheler, R., Evans, M., Showers, W., Hoffmann, S., Lotti-Bond, R., Hajdas, I., and Bonani, G.: Persistent solar influence on North Atlantic climate during the Holocene, Science, 294, 2130–2136, 2001.
Bondarenko, N. A., Belykh, O. I., Golobokova, L. P., Artemyeva, O. V., Logacheva, N. F., Tikhonova, I. V., Lipko, I. A., Kostornova, T. Y., Parfenova, V. V., Khodzher, T. V., Ahn, T. S., and Zo, Y. G.: Stratified distribution of nutrients and extremophile biota within freshwater ice covering the surface of Lake Baikal, J. Microbiol., 50, 8–16, 2012.
Borcard, D., Gillet, F., and Legendre, P.: Numerical ecology with R, 2nd edn., Use R! series, Springer International Publishing AG, Xv + 435 pp., ISBN 978-3-319-71404-2, 2018.
Bradbury, J. P., Bezrukova, Y. V., Chernyaeva, G. P., Colman, S. M., Khursevich, G., King, J. W., and Likoshway, Y. V.: A synthesis of post-glacial diatom records from Lake Baikal, J. Paleolimnol., 10, 213–252, 1994.
Broecker, W. S., Denton, G. H., Edwards, R. L., Cheng, H., Alley, R. B., and Putnam, A. E.: Putting the Younger Dryas cold event into context, Quaternary Sci. Rev., 29, 1078–1081, 2010.
Burson, A., Stomp, M., Greenwell, E., Grosse, J., and Huisman, J.: Competition for nutrients and light: testing advances in resource competition with a natural phytoplankton community, Ecology, 99, 1108–1118, 2018.
Bush, A. B. G.: and orbitally driven climate variability over central Asia through the Holocene, Quatern. Int., 136, 15–23, 2005.
Callander, E. and Granina, L.: Biogeochemical silica mass balance in Lake Baikal, Russia, in: Proceedings 8th International Symposium of Water–Rock Interaction, 1st edn., edited by: Kharaka, Y. K., Chudaev, O. V., Thordsen, J. J., Armannsson, H., Breit, G. N., Evans, W. C., and Keith, T. E. C., Balkema, Rotterdam, 341–344, https://doi.org/10.1201/9780203734049, 1995.
Cardinale, B. J., Hillebrand, H., Harpole, W. S., Gross, K., and Ptacnik, R.: Separating the influence of resource “availability” from resource “imbalance” on productivity–diversity relationships, Ecol. Lett., 12, 475–487, 2009.
Cardinale, B. J., Duffy, J. E., Gonzalez, A., Hooper, D. U., Perrings, C., Venail, P., Narwani, A., Mace, G. M., Tilman, D., Wardle, D. A., and Kinzig, A. P.: Biodiversity loss and its impact on humanity, Nature, 486, 59–67, 2012.
Chao, A., Gotelli, N. J., Hsieh, T. C., Sander, E. L., Ma, K. H., Colwell, R. K., and Ellison, A. M.: Rarefaction and extrapolation with Hill numbers: a framework for sampling and estimation in species diversity studies, Ecol. Monogr., 84, 45–67, 2014.
Charlet, F., Fagel, N., De Batist, M., Hauregard, F., Minnebo, B., Meischner, D., and SONIC Team: Sedimentary dynamics on isolated highs in Lake Baikal: evidence from detailed high-resolution geophysical data and sediment cores, Global Planet. Change, 46, 125–144, 2005.
Chase, J., and Leibold, M.: Spatial scale dictates the productivity–biodiversity relationship, Nature, 416, 427–430, 2002.
Chivian, E.: Biodiversity: its importance to human health. Interim Executive Summary, Centre for Health and the Global Environment, Harvard Medical School, Harvard, 2003.
Deevey, E.: Coaxing history to conduct experiments, Bioscience, 19, 40–43, 1969.
Demske, D., Heumann, G., Granoszewski, W., Nita, M., Mamakowa, K., Tarasov, P. E., and Oberhänsli, H.: Late glacial and Holocene vegetation and regional climate variability evidenced in high-resolution pollen records from Lake Baikal, Global Planet. Change, 46, 255–279, 2005.
Denton, G. H., Anderson, R. F., Toggweiler, J. R., Edwards, R. L., Schaefer, J. M., and Putman, A. E.: The Last Glacial Termination, Science, 328, 1652–1656, 2010.
Dixit, Y., Hodell, D. A., and Petrie, C. A.: Abrupt weakening of the summer monsoon in northwest India ∼4100 yr ago, Geology, 42, 339–342, 2014.
Dodson, S. I., Arnott, S. E., and Cottingham, K. L.: The relationship in lake communities between primary productivity and species richness, Ecology, 81, 2662–2679, 2000.
Dykoski, C. A., Edwards, R. L., Cheng, H., Yuan, D., Cai, Y., Zhang, M., Lin, Y., Qing, J., An, Z., and Revenaugh, J.: A high-resolution, absolute-dated Holocene and deglacial Asian monsoon record from Dongge Cave, China, Earth Planet. Sc. Lett., 233, 71–86, 2005.
Edlund, M. B.: Persistent low diatom plankton diversity within the otherwise highly diverse Lake Baikal ecosystem, Nova Hedwigia, 1310, 339–356, 2006.
Felde, V.: Vivian-Felde/Diatom_diversity_Vydrino_Lake_Baikal: Public release (v.1.0.0), Zenodo [code], https://doi.org/10.5281/zenodo.6124051, 2022.
Fietz, S., Sturm, M., and Nicklisch, A.: Flux of lipophilic photosynthetic pigments to the surface sediments of Lake Baikal, Global Planet. Change, 46, 29–44, 2005.
Fisher, T. G., Smith, D. G., and Andrews, J. T.: Preboreal oscillation caused by a glacial Lake Agassiz flood, Quaternary Sci. Rev., 21, 873–878, 2002.
Galazii, G. I.: State of the ecosystem of Lake Baikal and its catchment area: problems of conservation and rational use of resources, in: Conservation and Management of Lakes, edited by: Salánki, J. and Herodek, S., Symp. Biol. Hung., 38, 349–359, 1989.
Ganopolski, A., Kubatzki, C., Claussen, M., Brovkin, V., and Petoukhov, V.: The influence of vegetation-atmosphere-ocean interaction on climate during the mid-Holocene, Science, 280, 1916–1919, 1998.
Gotelli, N. J. and Ellison, A.: A Primer of Ecological Statistics, Sinauer Associates, Inc. Pub., Sunderland, Massachusetts, 510 pp., ISBN 0878932690, 2013.
Granin, N. G., Jewson, D. H., Gnatovsky, R. Yu., Levin, L. A., Zhdanov, A. A., Gorbunova, L. A., Tsekhanovsky, V. V., Doroschenko, L. M., and Mogilev, N. Yu: Turbulent mixing under ice and the growth of diatoms in Lake Baikal, Verh. Internat. Verein. Limnol., 27, 2812–2814, 2000.
Gross, K. and Cardinale, B. J.: Does species richness drive community production or vice versa? Reconciling historical and contemporary paradigms in competitive communities, Am. Nat., 170, 207–220, 2007.
Hagen, J. G., Vanschoenwinkel, B., and Gamfeldt, L.: We should not necessarily expect positive relationships between biodiversity and ecosystem functioning in observational field data, Ecol. Lett., 24, 2537–2548, https://doi.org/10.1111/ele.13874, 2021.
Hampton, S. E, Galloway, A. W. E., Powers, S. M., Ozersky, T., Woo, K. H., Batt, R. D., Labou, S. G., O'Reilly, C. M., Sharma, S., Lottig, N. R., Stanley, E. H., North, R. L., Stockwell, J. D., Adrian, R., Weyhenmeyer, G. A., Arvola, L., Baulch, H. M., Bertani, I., Bowman Jr., L. L., Carey, C. C., Catalan, J., Colom-Montero, W., Domine, L. M., Felip, M., Granados, I., Gries, C., Grossart, H.-P., Haberman, J., Haldna, M., Hayden, B., Higgins, S. N., Jolley, J. C., Kahilainen, K. K., Kaup, E., Kehoe, M. J., MacIntyre, S., Mackay, A. W., Mariash, H. L., McKay, R. M., Nixdorf, B,. Nõges, P., Nõges, T., Palmer, M., Pierson, D. C., Post, D. M., Pruett, M. J., Rautio, M., Read, J. S., Roberts, S. L., Rücker, J., Sadro, S., Silow, E. A., Smith, D. E., Sterner, R. W., Swann, G. E. A., Timofeyev, M. A., Toro, M., Twiss, M. R., Vogt, R. J., Watson, S. B., Whiteford, E. J., and Xenopoulos, M. A.: Ecology under lake ice, Ecol. Lett., 20, 98–111, 2017.
Helama, S., Jones, P. D., and Briffa, K. R.: Dark Ages Cold Period: a literature review and directions for future research, Holocene, 27, 1600–1606, 2017.
Hill, M. O.: Diversity and evenness: A unifying notation and its consequences, Ecology, 54, 427–432, 1973.
Hillebrand, H., Dürselen, C. D., Kirschtel, D., Pollingher, U., and Zohary, T.: Biovolume calculations for pelagic and benthic microalgae, J. Phycol., 35, 403–424, 1999.
Horiuchi, K., Matsuzaki, H., Osipov, E., Khlystov, O., and Fujii, S.: Cosmogenic 10Be and 26Al dating of erratic boulders in the southern coastal area of Lake Baikal, Siberia, Nucl. Instrum. Meth. B, 223, 633–638, 2004.
Huisman, J., Sharples, J., Stroom, J. M., Visser, P. M., Kardinaal, W. E. A., Verspagen, J. M., and Sommeijer, B.: Changes in turbulent mixing shift competition for light between phytoplankton species, Ecology, 85, 2960–2970, 2004.
Interlandi, S. J. and Kilham, S. S.: Limiting resources and the regulation of diversity in phytoplankton communities, Ecology, 82, 1270–1282, 2001.
Isbell, F., Craven, D., Connolly, J., Loreau, M., Schmid, B., Beierkuhnlein, C., Bezemer, T. M., Bonin, C., Bruelheide, H., De Luca, E., Ebeling, A., Griffin, J. N., Guo, Q., Hautier, Y., Hector, A., Jentsch, A., Kreyling, J., Lanta, V., Manning, P., Meyer, S. T., Mori, A. S., Naeem, S., Niklaus, P. A., Polley, W., Reich, P. B., Roscher, C., Seabloom, E. W., Smith, M. D., Thakur, M. P., Tilman, D., Tracy, B. F., van der Putten, W. H., can Ruijven, J., Weigelt, A., Weisser, W. W., Wilsey, B., and Eisenhauer, N.: Biodiversity increases the resistance of ecosystem productivity to climate extremes, Nature, 526, 574–577, 2015.
Izmest'eva, L. R., Moore, M. V., and Hampton, S.E.: Seasonal dynamics of common phytoplankton in Lake Baikal, in: Proceedings of Samara RAS (Russian Academy of Sciences) Scientific Centre, 8, 191–196, 2006 (in Russian).
Izmest'eva L. R, Moore, M. V., Hampton, S. E., Ferwerda, C. J., Gray, D. K., Woo, K. H., Pislegina, H. V., Krashchuk, L. S., Shimaraeva, S. V., and Silow, E. A.: Lake-wide physical and biological trends associated with warming in Lake Baikal, J. Great Lakes Res., 42, 6–17, 2016.
Jewson, D. H. and Granin, N. G.: Cyclical size change and population dynamics of a planktonic diatom, Aulacoseira baicalensis, in Lake Baikal, Eur. J. Phycol., 50, 1–19, https://doi.org/10.1080/09670262.2014.979450, 2015.
Jewson, D. H., Granin, N. G., Zhdanov, A. A., Gorbunova, L. A., Bondarenko, N. A., and Gnatovsky, R. Yu.: Resting stages and ecology of the planktonic diatom Aulacoseira skvortzowii in Lake Baikal, Limnol. Oceanogr., 53, 1125–1136, 2008.
Jewson, D. H., Granin, N. G., Zhdarnov, A. A., Gorbunova, L. A., and Gnatovsky, R. Y.: Vertical mixing, size change and resting stage formation of the planktonic diatom Aulacoseira baicalensis, Eur. J. Phycol., 45, 354–364, 2010.
Jewson, D. H., Granin, N. G., Gnatovsky, R. Y., Lowry, S. F., and Teubner, K., Coexistence of two Cyclotella diatom species in the plankton of Lake Baikal, Freshwater Biol., 60, 2113–2126, 2015.
Jost, L.: The relation between evenness and diversity, Diversity, 2, 207–232, 2010.
Jovanovska, E., Cvetkoska, A., Hauffe, T., Levkov, Z., Wagner, B., Sulpizio, R., Francke, A., Albrecht, C., and Wilke, T.: Differential resilience of ancient sister lakes Ohrid and Prespa to environmental disturbances during the Late Pleistocene, Biogeosciences, 13, 1149–1161, https://doi.org/10.5194/bg-13-1149-2016, 2016.
Juggins, S.: C2 version 1.7.7: Software for ecological and palaeoecological data, University of Newcastle, Newcastle, available at: https://www.staff.ncl.ac.uk/stephen.juggins/software/C2Home.htm (last access: 1 November 2021), 2014.
Kéfi, S., Domínguez-Garcia, V., Donohue, I., Fontaine, C., Thébault, E., and Dakos, V.: Advancing out knowledge of ecological stability, Ecol. Lett., 22, 1349–1356, 2019.
Khursevich, G. K., Karabanov, E. B., Prokopenko, A. A., Williams, D. F., Kuzmin, M. I., Fedenya, S. A., and Gvozdkov, A. A.: Insolation regime in Siberia as a major factor controlling diatom production in Lake Baikal during the past 800,000 years, Quatern. Int., 80–81, 47–58, 2001.
Khursevich, G. K., Prokopenko, A. A., Fedenya, S. A., Tkachenko, L. I., and Williams, D. F.: Diatom biostratigraphy of Lake Baikal during the past 1.25 Ma: new results from BDP-96-2 and BDP-99 drill cores, Quatern. Int., 136, 95–104, 2005.
Kilham, R., Kilham, S. S., and Hecky, R. E.: Hypothesized resource relationships among African planktonic diatoms, Limnol. Oceanogr., 31, 1169–1181, 1986.
Korhonen, J. J., Wang, J., and Soininen, J.: Productivity–diversity relationships in lake plankton communities, PloS One, 6, e22041, https://doi.org/10.1371/journal.pone.0022041, 2011.
Kozhov, M.: Lake Baikal and its life, W. Junk, The Hague, 344 pp., ISBN 978-90-6193-064-8, 1963.
Kozhova, O. M. and Izmest'eva, L. R.: Lake Baikal: evolution and diversity, Backhuys Publishers, Leiden, ISBN 90-5782-001-3, 1998.
Kravtsova, L. S., Izhboldina, L. A., Khanaev, I. V., Pomazkina, G. V., Rodionova, E. V., Domysheva, V. M., Sakirko, M. V., Tomberg, I. V., Kostornova, T. Y., Kravchenko, O. S., and Kupchinsky, A. B.: Nearshore benthic blooms of filamentous green algae in Lake Baikal, J. Great Lakes Res., 40, 441–448, 2014.
Legendre, P. and Gallagher, E. D.: Ecologically meaningful transformations for ordination of species data, Oecologia, 129, 271–280, 2001.
Loreau, M., Naeem, S., Inchausti, P., Bengtsson, J., Grime, J. P., Hector, A., Hooper, D. U., Huston, M. A., Raffaelli, D., Schmid, B., and Tilman, D.: Biodiversity and ecosystem functioning: current knowledge and future challenges, Science, 294, 804–808, 2001.
Luethje, M. and Snyder, J.: Climate-related morphological changes in Pantocsekiella (Mediophyceae) spanning 0–1.2 Ma in the Lake El'gygytgyn, northeastern Russia including Pantocsekiella elgygytgynensis sp. nov., Phytotaxa, 478, 67–91, 2021.
Mackay, A. W.: The paleoclimatology of Lake Baikal: a diatom synthesis and prospectus, Earth Sci. Rev., 82, 181–215, 2007.
Mackay, A. W., Flower, R. J., Kuzmina, A. E., Granina, L. Z., Rose, N. L., Appleby, P. G., Boyle, J. F., and Battarbee, R. W.: Diatom succession trends in recent sediments from Lake Baikal and their relation to atmospheric pollution and to climate change, Philos. T. R. Soc. B, 353, 1011–1055, 1998.
Mackay, A. W., Swann, G. E. A., Brewer, T. S., Leng, M. J., Morley, D. W., Piotrowska, N., Rioual, P., and White, D.: A reassessment of late glacial – Holocene diatom oxygen isotope record from Lake Baikal using a geochemical mass-balance approach, J. Quaternary Sci., 26, 627–634, 2011.
Mackay, A. W., Seddon, A. W. R., Leng, M. J., Heumann, G., Morley, D. W., Piotrowska, N., Rioual, P., Roberts, S., and Swann, G. E. A.: Holocene carbon dynamics at the forest-steppe ecotone of southern Siberia, Global Change Biol., 23, 1942–1960, 2017.
Mackay, A. W., Felde, V. A. F., Morley, J. J., Piotrowska, N., Rioual, P. R., Seddon, A. W. R. S., and Swann, G. E. A.: NOAA/WDS Paleoclimatology – Diatom diversity and palaeoproductivity data from Lake Baikal, Southern Siberia over the past 16 000 years, NOAA National Centers for Environmental Information [data set], https://doi.org/10.25921/q6v1-5q02, 2022.
Marcott, S. A., Shakun, J. D., Clark, P. U., and Mix, A. C.: A reconstruction of regional and global temperature for the past 11,300 years, Science, 339, 1198–1201, 2013.
Mayewski, P. A., Meeker, L. D., Twickler, M. S., Whitlow, S., Yang, Q., and Prentice, M.: Major features and forcing of high latitude Northern Hemisphere atmospheric circulation using a 110,000 year long glaciochemical series, J. Geophys. Res., 102, 26345–26366, 1997.
Mayewski, P. A., Rohling, E. E., Stager, J. C., Karlén, W., Maasch, K. A., Meeker, L. D., Meyerson, E. A., Gasse, F., van Kreveld, S., Holmgren, K., Lee-Thorp, J., Rosqvist, G., Rack, F., Staubwasser, M., Schneider, R. R., and Steig, E. J.: Holocene climate variability, Quaternary Res., 62, 243–255, 2004.
McCann, K. S.: The diversity-stability debate, Nature, 405, 228–233, 2000.
McGrady-Steed, J., Harris, P. M., and Morin, P. J.: Biodiversity regulates ecosystem predictability, Nature, 390, 162–165, 1997.
McManus, J. F., Francois, R., Gherardi, J.-M., Keigwin, L. D., and Brown-Leger, S.: Collapse and rapid resumption of Atlantic meridional circulation linked to deglacial climate changes, Nature, 428, 834–837, 2004.
Murton, J. B., Bateman, M. D., Dallimore, S. R., Teller, J. T., and Yang, Z.: Identification of Younger Dryas outburst flood path from Lake Agassiz to the Arctic Ocean, Nature, 464, 740–743, 2010.
National Research Council: The geological record of ecological dynamics: understanding the biotic effects of future environmental change, The National Academies Press, Washington DC, USA, https://doi.org/10.17226/11209, 2005.
Nesje, A., Jansen, E., Birks, H. J .B., Bjune, A. E., Bakke, J., Andersson, C., Dahl, S. O., Kristensen, D. K., Lauritzen, S., Lie, O., and Risebrobakken, B.: Holocene climate variability in the northern North Atlantic region: a review of terrestrial and marine evidence, Geophys. Monogr. Ser., 158, 289–322, https://doi.org/10.1029/158GM19, 2005.
O'Donnell, D. R., Wilburn, P., Silow, E. A., Yampolsky, L. Y., and Litchman, E: Nitrogen and phosphorus colimitation of phytoplankton in Lake Baikal: insights from a spatial survey and nutrient enrichment experiments, Limnol. Oceanogr., 62, 1383–1392, 2017.
Oksanen, J., Blanchet, F. G., Friendly, M., Kindt, R., Legendre, P., McGlinn, D., Minchin, P. R., O'Hara, R. B., Simpson, G. L., Solymos, P., Stevens, M. H. H., Szoecs, E., and Wagner, H.: vegan: Community Ecology Package, R package version 2.5-7, available at: https://CRAN.R-project.org/package=vegan (last access: 15 August 2021), 2020.
O'Reilly, C. M., Sharma, S., Gray, D. K., Hampton, S. E., Read, J. S., Rowley, R. J., Schneider, P., Lenters, J. D., McIntyre, P. B., Kraemer, B. M., Weyhenmeyer, G. A., Straile, D., Dong, B., Adrian, R., Allan, M.G., Anneville, O., Arvola, L., Austin, J., Bailey, J. L. , Baron, J. S., Brookes, J. D., de Eyto, E., Dokulil, M. T., Hamilton, D. P., Havens, K., Hetherington, A. L., Higgins, S. N., Hook, S., Izmest'eva, L. R., Joehnk, K. D., Kangur, K., Kasprzak, P., Kumagai, M., Kuusisto, E., Leshkevich, G., Livingstone, D. M., MacIntyre, S., May, L., Melack, J. M., Mueller-Navarra, D. C., Naumenko, M., Noges, P., Noges, T., North, R. P., Plisnier, P.-D., Rigosi, A., Rimmer, A., Rogora, M., Rudstam, L. G., Rusak, J. A., Salmaso, N., Samal, N. R., Schindler, D. E., Schladow, S. G., Schmid, M., Schmidt, S. R., Silow, E., Soylu, M. E., Teubner, K., Verburg, P., Voutilainen, A., Watkinson, A., Williamson, C. E., and Zhang, G.: Rapid and highly variable warming of lake surface waters around the globe, Geophys. Res. Lett., 42, 10773–10781, 2015.
Orlóci, L.: An agglomerative method for classification of plant communities, J. Ecol., 55, 193–205, 1967.
Osipov, E. Y. and Khlystov, O. M.: Glaciers and meltwater flux to Lake Baikal during the Last Glacial Maximum, Palaeogeogr. Palaeocl., 294, 4–15, 2010.
Panizzo, V. N., Swann, G. E. A., Mackay, A. W., Vologina, E., Alleman, L., Andre, L., Pashley, V. H., and Horstwood, M. S. A: Constraining modern day silicon cycling in Lake Baikal, Global. Biogeochem. Cy., 31, 556–574, 2017.
Piotrowska, N., Bluszcz, A., Demske, D., Granoszewski, W., and Heumann, G.: Extraction and AMS radiocarbon dating of pollen from Lake Baikal sediments, Radiocarbon, 46, 181–187, 2004.
Popovskaya, G. I.: Ecological monitoring of phytoplankton in Lake Baikal, Aquat. Ecosyst. Health, 3, 215–225, 2000.
Popovskaya, G. I., Usol'tseva, M. V., Domysheva, V. M., Sakirko, M. V., Blinov, V. V., and Khodzher, T. V.: The spring phytoplankton in the pelagic zone of Lake Baikal during 2007–2011, Geogr. Nat. Resour., 36, 253–262, 2015.
Ptacnik, R., Solimini, A. G., Andersen, T., Tamminen, T., Brettum, P., Lepistö, L., Willén, E., and Rekolainen, S.: Diversity predicts stability and resource use efficiency in natural phytoplankton communities, P. Natl. Acad. Sci. USA, 105, 5134–5138, 2008.
Rasmussen, D. O., Andersen, K. K., Svensson, A. M., Steffensen, J. P., Vinther, B. M., Clausen, H. B., Siggaard-Andersen, M.-L., Johnsen, S. J., Larsen, L. B., Dahl-Jensen, D., Bigler, M., Rothlisberger, R., Fischer, H., Goto-Azuma, K., Hansson, M. E., and Ruth, U.: A new Greenland ice core chronology for the last glacial termination, J. Geophys. Res, 111, D06102, https://doi.org/10.1029/2005JD006079, 2006.
R Core Team: R: A Language and Environment for Statistical Computing, R Foundation for Statistical Computing, Vienna, Austria, 2021.
Reimer, P. J., Austin, W. E. N., Bard, E., Bayliss, A., Blackwell, P. G., Bronk Ramsey, C., Butzin, M., Cheng, H., Edwards, R. L., Friedrich, M., Grootes, P. M., Guilderson, T. P., Hajdas, I., Heaton, T. J., Hogg, A.G., Hughen, K. A., Kromer, B., Manning, S. W., Muscheler, R., Palmer, J. G., Pearson, C., van der Plicht, J., Reimer, R. W., Richards, D. A., Scott, E. M., Southon, J. R., Turney, C. S. M., Wacker, L., Adolphi, F., Büntgen, U., Capano, M., Fahrni, S. M., Fogtmann-Schulz, A., Friedrich, R., Köhler, P., Kudsk, S., Miyake, F., Olsen, J., Reinig, F., Sakamoto, M., Sookdeo, A., and Talamo, S.: The IntCal20 Northern Hemisphere radiocarbon age calibration curve (0–55 cal kBP), Radiocarbon, 62, 725–757, 2020.
Renssen, H., Goosse, H., and Muscheler, R.: Coupled climate model simulation of Holocene cooling events: oceanic feedback amplifies solar forcing, Clim. Past, 2, 79–90, https://doi.org/10.5194/cp-2-79-2006, 2006.
Reshetova, S. A., Bezrukova, E. V., Panizzo, V., Henderson, A., Ptitsyn, A. B., Daryin, A. V., and Kalugin, I. A.: Vegetation of Central Transbaikalia in the Late Glacial Period and Holocene, Geography and Natural Resources, 34, 172–178, 2013.
Roberts, S., Swann, G. E. A., McGowan, S., Panizzo, V., Vologina, E., Sturm, M., and Mackay, A. W.: Diatom evidence of 20th Century ecosystem change in Lake Baikal, Siberia, PLoS One, 13, e0208765, https://doi.org/10.1371/journal.pone.0208765, 2018.
Rusak, J. A., Leavitt, P. R., McGowan, S., Chen, G., Olson, O., Wunsam, S., and Cumming, B. F.: Millennial-scale relationships of diatom species richness and production in two prairie lakes, Limnol. Oceanogr., 49, 1290–1299, 2004.
Ryves, D. B., Jewson, D. H., Sturm, M., Battarbee, R. W., Flower, R. J., Mackay, A. W., and Granin, N. G.: Quantitative and qualitative relationships between planktonic diatom communities and diatom assemblages in sedimenting material and surface sediments in Lake Baikal, Siberia, Limnol. Oceanogr., 48, 1183–1192, 2003.
Satoh, Y., Katano, T., Satoh, T., Mitamura, O., Anbutsu, K., Nakano, S., Ueno, H., Kihira, M., Drucker, V., Tanaka, Y., Mimura, T., Watanabe, Y., and Sugiyama, M.: Nutrient limitation of the primary production of phytoplankton in Lake Baikal, Limnology, 7, 225–229, 2006.
Seddon, A. W., Mackay, A. W., Baker, A. G., Birks, H. J. B., Breman, E., Buck, C. E., Ellis, E. C., Froyd, C. A., Gill, J. L., Gillson, L., and Johnson, E. A.: Looking forward through the past: identification of 50 priority research questions in palaeoecology, J. Ecol., 102, 256–267, 2014.
Shimaraev, M. N. and Domysheva, V. M.: Trends in hydrological and hydrochemical processes in Lake Baikal under conditions of modern climate change, in: Climatic Change and Global Warming of Inland Waters, edited by: Colman, C. R., Kumagai, M., and Robarts, R. D., John Wiley & Sons Ltd, Chichester, UK, 43–66, 2013.
Shimaraev, M. N. and Mizandrontsev, I. B.: Changes in the Lake Baikal ecosystem during the Late Pleistocene and Holocene, Hydrobiologia, 568, 259–263, 2006.
Smith, V. H.: Microbial diversity-productivity relationships in aquatic ecosystems, FEMS Microbiol. Ecol., 62, 181–186, 2007.
Soma, Y., Tani, Y., Soma, M., Mitake, H., Kurihara, R., Hashomoto, S., Watanabe, T., and Nakamura, T.: Sedimentary steryl chlorin esters (SCEs) and other photosynthetic pigments as indicators of palaeolimnological change over the last 28,000 years from the Buguldeika Saddle of Lake Baikal, J. Paleolimnol., 37, 163–175, 2007.
Sommer, U., Adrian, R., Domis, L. D. S., Elser, J. J., Gaedke, U., Ibelings, B., Jeppesen, E., Lürling, M., Molinero, J. C., Mooij, W. M., van Donk, E., and Winder, M.: Beyond the plankton ecology group (PEG) model: mechanisms driving plankton succession, Annu. Rev. Ecol. Evol. Syst., 43, 429–448, 2012.
Stuiver, M., Grootes, P. M., and Braziunas, T. F.: The GISP2 δ18O climatic record of the past 16,500 years and the role of the sun, ocean, and volcanoes, Quaternary Res., 44, 341–354, 1995.
Swann, G. E. A, Panizzo, V. N., Piccolroaz, S., Pashley, V., Horstwood, M. S. A., Roberts, S., Vologina, E., Piotrowska, N., Sturm, M., Zhdanoc, A., Granin, N., Normal, C., McGowan, S., and Mackay, A. W: Changing nutrient cycling in Lake Baikal: the world's oldest lake, P. Natl. Acad. Sci. USA, 117, 27211–27217, 2020.
Tani, Y., Kurihara, K., Nara, F., Itoh, N., Soma, M., Soma, Y., Tanaka, A., Yoneda, M., Hirota, M., and Shibata, Y.: Temporal changes in the phytoplankton community of the southern basin of Lake Baikal over the last 24,000 years recorded by photosynthetic pigments in a sediment core, Org. Geochem., 33, 1621–1634, 2002.
Tarasov, L. and Peltier W. R.: Arctic freshwater forcing of the Younger Dryas cold reversal, Nature, 435, 662–665, 2005.
Tarasov, P. E., Bezrukova, E. V., and Krivonogov, S. K.: Late Glacial and Holocene changes in vegetation cover and climate in southern Siberia derived from a 15 kyr long pollen record from Lake Kotokel, Clim. Past, 5, 285–295, https://doi.org/10.5194/cp-5-285-2009, 2009.
Thiagarajan, N., Subhas, A. V., Southon, J. R., Eiler, J. M., and Adkins, J. F.: Abrupt pre-Bølling–Allerød warming and circulation changes in the deep ocean, Nature, 511, 75–78, 2014.
Tilman, D., Knops, J., Wedin, D., Reich, P., Ritchie, M., and Siemann, E.: The influence of functional diversity and composition on ecosystem processes, Science, 277, 1300–1302, 1997.
Tilman, D., Reich, P. B., and Isbell, F.: Biodiversity impacts ecosystem productivity as much as resources, disturbance, or herbivory, P. Natl. Acad. Sci. USA, 109, 10394–10397, 2012.
Timoshkin, O. A., Samsonov, D. P., Yamamuro, M., Moore, M. V., Belykh, O. I., Malnik, V. V., Sakirko, M. V., Shirokaya, A. A., Bondarenko, N. A., Domysheva, V. M., Fedorova, G. A., Kochetkov, A. I., Kuzmin, A. V., Lukhnev, A. G., Medvezhonkova, O. V., Nepokrytykh, A. V., Pasynkova, E. M., Poberezhnaya, A. E., Potapskaya, N. V., Rozhkova, N. A., Sheveleva, N. G., Tikhonova, I. V., Timoshkina, E. M., Tomberg, I. V., Volkova, E. A., Zaitseva, E. P., Zvereva, Y. M., Kupchinsky, A. B., and Bukshuk, N. A.: Rapid ecological change in the coastal zone of Lake Baikal (East Siberia): Is the site of the world's greatest freshwater biodiversity in danger?, J. Great Lakes Res., 42, 487–497, 2016.
Walker, M., Head, M. J., Berkelhammer, M., Björck, S., Cheng, H., Cwynar, L., Fisher, D., Gkinis, V., Long, A., Lowe, J., and Newnham, R.: Formal ratification of the subdivision of the Holocene Series/Epoch (Quaternary System/Period): two new Global Boundary Stratotype Sections and Points (GSSPs) and three new stages/subseries, Episodes, 41, 213–223, 2018.
Wanner, H., Mercolli, L., Grosjean, M., and Ritz, S. P.: Holocene climate variability and change: a database review, J. Geol. Soc. Lond., 172, 254–263, 2014.
Williams, D. F., Kuzmin, M. I., Prokopenko, A. A., Karabanov, E. B., Khursevich, G. K., and Bezrukova, E.V.: The Lake Baikal drilling project in the context of a global lake drilling initiative, Quatern. Int., 80, 3–18, 2001.
Williams, J. W., Blois, J. L., and Shuman, B. N.: Extrinsic and intrinsic forcing of abrupt ecological change: case studies from the late Quaternary, J. Ecol., 99, 664–677, 2011.
Winfree, R., Fox, J. W., Williams, N. M., Reilly, J. R., and Cariveau, D. P.: Abundance of common species, not species richness, drives delivery of a real-world ecosystem service, Ecol. Lett., 18, 626–635, 2015.
Wood, S. N.: Fast stable restricted maximum likelihood and marginal likelihood estimation of semiparametric generalized linear models, J. R. Stat. Soc. B, 73, 3–36, 2011.
Yachi, S. and Loreau, M.: Biodiversity and ecosystem productivity in a fluctuating environment: the insurance hypothesis, P. Natl. Acad. Sci. USA, 96, 1463–1468, 1999.