the Creative Commons Attribution 4.0 License.
the Creative Commons Attribution 4.0 License.
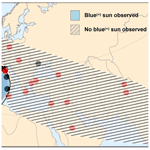
The blue suns of 1831: was the eruption of Ferdinandea, near Sicily, one of the largest volcanic climate forcing events of the nineteenth century?
Christopher Garrison
Christopher Kilburn
David Smart
Stephen Edwards
One of the largest climate forcing eruptions of the nineteenth century was, until recently, believed to have taken place at the Babuyan Claro volcano, in the Philippines, in 1831. However, a recent investigation found no reliable evidence of such an eruption, suggesting that the 1831 eruption must have taken place elsewhere. We here present our newly compiled dataset of reported observations of a blue, purple and green sun in August 1831, which we use to reconstruct the transport of a stratospheric aerosol plume from that eruption. The source of the aerosol plume is identified as the eruption of Ferdinandea, which took place about 50 km off the south-west coast of Sicily (37.1∘ N, 12.7∘ E), in July and August 1831. The modest magnitude of this eruption, assigned a volcanic explosivity index (VEI) of 3, has commonly caused it to be discounted or overlooked when identifying the likely source of the stratospheric sulfate aerosol in 1831. It is proposed, however, that convective instability in the troposphere contributed to aerosol reaching the stratosphere and that the aerosol load was enhanced by addition of a sedimentary sulfur component to the volcanic plume. Thus, one of the largest climate forcing volcanic eruptions of the nineteenth century would effectively have been hiding in plain sight, arguably “lowering the bar” for the types of eruptions capable of having a substantial climate forcing impact. Prior estimates of the mass of stratospheric sulfate aerosol responsible for the 1831 Greenland ice core sulfate deposition peaks which have assumed a source eruption at a low-latitude site will, therefore, have been overstated. The example presented in this paper serves as a useful reminder that VEI values were not intended to be reliably correlated with eruption sulfur yields unless supplemented with compositional analyses. It also underlines that eye-witness accounts of historical geophysical events should not be neglected as a source of valuable scientific data.
- Article
(10486 KB) - Full-text XML
- BibTeX
- EndNote
Volcanic eruptions that produce sulfate aerosols in the stratosphere are important climate forcing events (Robock, 2000). Ranked in order of the mass of stratospheric sulfate aerosol produced, the most important climate forcing volcanic eruptions of the nineteenth century are as follows: Tambora, in Indonesia, in 1815 (120 Tg); an unidentified eruption in 1809 (59 Tg); Cosegüina, in Nicaragua, in 1835 (40 Tg); Krakatoa, in Indonesia, in 1883 (27 Tg); and another unidentified eruption in 1831 (17 Tg) (Gao et al., 2008). The combination of the 1831 eruption and the 1835 Cosegüina eruption contributed to delaying the end of the Little Ice Age and, hence, the onset of modern anthropogenic warming, until 1850 (Brönnimann et al., 2019). Until recently, the 1831 eruption had commonly been assumed to be an eruption of Babuyan Claro, in the Philippines, which had notionally been assigned a volcanic explosivity index (VEI) of 4 (Zielinski, 1995; Global Volcanism Program, 2013; Arfeuille et al., 2014; Toohey and Sigl, 2017). However, Garrison et al. (2018) found no reliable evidence of such an eruption in 1831, suggesting that the climate forcing eruption must have taken place elsewhere.
Observations of a blue, purple and green sun occurred around the world in August 1831 (Arago, 1832; Kiessling, 1888; Symons et al., 1888). The sun is white when viewed from above Earth's atmosphere. At Earth's surface, however, its observed colour varies due to scattering and absorption by atmospheric gases and aerosols (Bohren and Huffman, 2004). A sufficiently dense aerosol of solid particles or liquid droplets with a radius of about 0.5 µm, and a refractive index of about 1.5 may alter the observed colour of the sun to a pronounced blue, purple or green (La Mer and Kerker, 1953; Penndorf, 1953; Van de Hulst, 1981; Porch, 1989; Horvath et al., 1994; Ehlers et al., 2014; Wullenweber et al., 2021). Such an aerosol is occasionally produced by a volcanic eruption, such as the 1880 eruption of Cotopaxi in Ecuador (Whymper, 1884) or the 1883 eruption of Krakatau in Indonesia (Symons et al., 1888), or by a forest fire, such as the 1950 Chinchaga fire in Canada (Bull, 1951; Wilson, 1951). For as long as an aerosol maintains these parameters whilst being transported in the atmosphere, it will produce a sequence of observations of a blue, purple or green sun at different dates and places. Consequently, a sequence of reported observations of a blue, purple or green sun may be used to reconstruct the atmospheric transport of the aerosol responsible, potentially tracing it back to its source (Symons et al., 1888).
Here, we present our newly compiled dataset of reported observations of a blue, purple and green sun in August 1831, which we use to reconstruct the transport of a stratospheric aerosol plume from the 1831 eruption. We are thus able to constrain the location of the eruption to a mid-latitude site between 30 and 45∘ N. Using additional reports where a blue, purple or green sun was not seen, despite active observation, we are also able to constrain its longitude and, hence, identify it as the eruption of Ferdinandea, which took place about 50 km off the south-west coast of Sicily (37.1∘ N, 12.7∘ E) in July and August 1831. The modest magnitude of this eruption, assigned a VEI of 3 (Global Volcanism Program, 2013), i.e. 10 times smaller in terms of the volume of ejected material than a VEI of 4, has commonly caused it to be discounted or overlooked when identifying the likely source of the stratospheric sulfate aerosol in 1831 (Camuffo and Enzi, 1995; Zielinski, 1995; Robertson et al., 2001, Arfeuille et al., 2014; Toohey and Sigl, 2017). However, we hypothesize that convective instability in the troposphere contributed to aerosol reaching the stratosphere and that the aerosol load was enhanced by addition of a sedimentary sulfur component to the volcanic plume.
For simplicity, we use the term “blue(+)” to include the colours blue, purple and green. A literature search was undertaken to collect as many reported observations of a blue(+) sun in 1831 as possible. The observations compiled by Arago (1832), Kiessling (1888) and Symons et al. (1888) were traced, as far as possible, to their primary sources. Additional primary sources were identified using a combination of “structured” searches (e.g. reviewing obviously relevant national and local newspapers, scientific journals, and collections of published accounts of travel and residence) and “unstructured” searches (e.g. keyword searches of digital archives). A minimum requirement was that a blue(+) sun was observed at least once during the day and that the date and place of the observation was recorded. To determine the boundaries of the region in which a blue(+) sun was observed, the search also extended to reports recording active observation but without a blue(+) sun having been seen (“null” observations). Most of the source materials studied were originally written in western European languages, although at least some were originally written in Arabic, Mandarin and Russian. Online search engines facilitated access to relevant historical materials and translation tools. Supplemental searches of non-digital archives at, for example, the Observatoire de Paris, the Osservatorio Astronomico di Palermo and the British Library were also undertaken. In the course of the search, reported observations of other unusual atmospheric optical phenomena in 1831 were noted and collected and will be referred to in this paper as appropriate.
3.1 Reported observations
Thirty one primary sources reporting observations of a blue(+) sun are summarized in Appendix A. The text of three representative reports is reproduced in Table 1. Fifteen of these sources had been identified in Arago (1832), Kiessling (1888) and Symons et al. (1888), but the remaining sixteen are newly identified here. Seventeen primary sources reporting null observations are also summarized in Appendix B. The original language of the sources in Appendices A and B is English (60 %), French (17 %), Italian (8 %), German (6 %), Spanish (4 %), Catalan (2 %) and Arabic (2 %). By type, they comprise newspaper reports (35 %), published accounts of travel and residence (25 %), observational (meteorological) registers published in newspapers or scientific journals (19 %), communications to learned societies published in scientific journals (15 %), letters to newspapers (4 %), and other published records (2 %).
The reported blue(+) sun observations took place between 3 August 1831 (source [A1]) and around 28 August 1831 (source [A31]) in Europe, the Caribbean, the north Atlantic, the USA and China (Fig. 1). The sites were located at latitudes between 19 and 47∘ N, although about 85 % were restricted to between 30 and 45∘ N (Figs. 1, 2). The locations of the sites are evidently biased toward regions which had comparatively high population densities in 1831 and, moreover, populations which were likely to report observations in a form which remains accessible today, in particular in Europe and on the eastern coast of the USA. Carpenter (1884) reports that a blue sun was seen “at Washington”, in the USA, in “October, especially October 12… [and]… October 13… ” 1831. However, this appears to be the result of an erroneous conflation between the date and place of an observation on 12 and 13 August 1831 in Alexandria, Virginia, about 10 km from Washington (source [A18]), and the publication of this observation in the October edition of Niles' Weekly Register (1831).
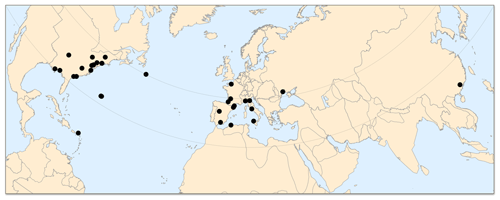
Figure 1Location of blue(+) sun observations reported in August 1831 (Appendix A). The latitude band shown extends from 30 to 45∘ N. National borders are shown as correct for 1831 (based on data from Mathematica v. 12.0, Wolfram Research).
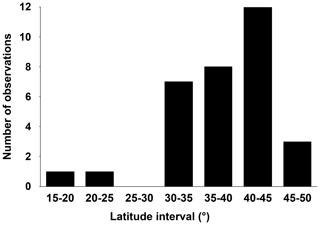
Figure 2Number of blue(+) sun observations reported in August 1831 in different latitude bands (Appendix A).
Additional observations of blue(+) sunlight in China in the summer of 1831 are reported in a Mandarin-language compendium of meteorological records (Zhang, 2004). However, this compendium is itself based on an earlier compendium, and the primary sources are not available. These observations have accordingly not been included in the present analysis.
3.2 A sequence of observations of a blue(+) sun
The reported observations of a blue(+) sun in 26 of the 31 sources (Appendix A) form a connected sequence. The earliest observation in the sequence is that reported in Palermo, Sicily, Italy (38.1∘ N, 13.4∘ E), at 18:00 LT on 8 August 1831 (source [A5]; Fig. 3a). Over the following 9 d, the locations of the observations move westward, from Europe to the USA, as well as spreading to the north and south, largely across the 30–45∘ N latitude band, although occasionally further north (to about 50∘ N; source [A12]) and further south (to about 20∘ N; source [A19]) (Figs. 3b–j, 4). The observations reported around 28 August 1831 (source [A31]) plausibly extend the connected sequence further westward to China (Fig. 4).
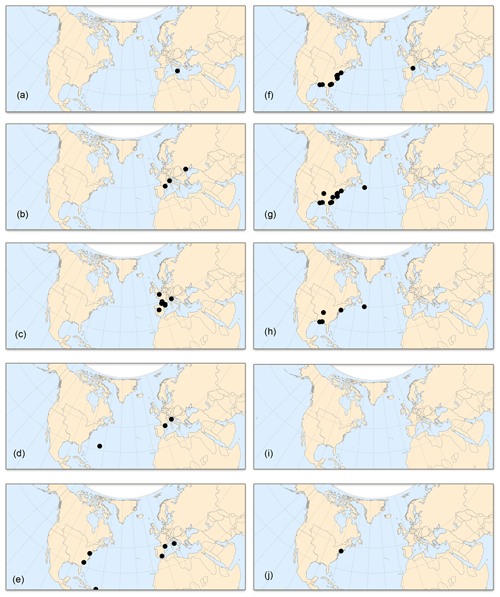
Figure 3(a–j) Progression of observations of a blue(+) sun reported between 8 and 17 August 1831 (Appendix A). The sequence of observations runs day by day from 8 August (a) to 12 August (e) and from 13 August (f) to 17 August (j). No reported observations of a blue(+) sun have been identified on 16 August (i).
At locations to the east of Sicily from Malta to India, including those across the 30–45∘ N latitude band, the sources identified reported null observations, i.e. despite observations being actively recorded, no blue(+) sun was seen (Appendix B; Fig. 5). (The apparently exceptional case of the observation reported in Odessa, Ukraine (source [A7]; Fig. 5) is discussed in Sect. 4.1.) The same was true for locations further north and north-west of Sicily from northern Italy, Switzerland, Hungary and Germany to the UK (Appendix B; Fig. 5). No reported observations could be found from locations to the south or south-west, across the Sahara Desert. On this basis, the eastern boundary of the region in which the observation of a blue(+) sun was reported in August 1831 can be delineated approximately by the A–A' curve in Fig. 5.
3.3 Reconstruction of aerosol transport
Given the geographical and temporal distribution of these blue(+) sun observations, we propose the following approximate reconstruction of the transport of the aerosol responsible. The aerosol source must have been located in the vicinity of Sicily, near the intersection of the A–A' curve with the centre of the 30–45∘ N latitude band (Fig. 5). It caused aerosol to be formed between 8 and 13 August 1831 at an altitude with atmospheric circulation from east to west, such that a plume of aerosol lengthened to the west of Sicily (Fig. 6a–f). On 13 August 1831, the aerosol plume extended between about 15∘ E and 90∘ W in longitude, over an area of about 14 000 000 km2 in the latitude band between 30 and 45∘ N (Fig. 6f; Table 2). Once the source had ceased to cause the formation of aerosol at that altitude, around 14 August 1831, the “detached” aerosol plume continued to be transported westward (Fig. 6g–j) eventually reaching China around 28 August 1831.
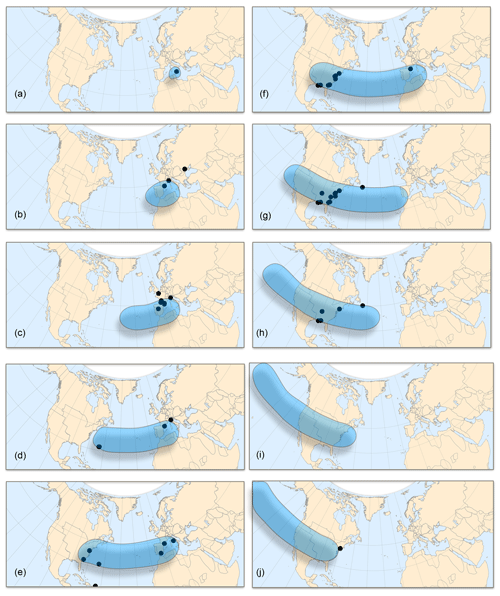
Figure 6(a–j) Approximate reconstruction of the generation and transport of the aerosol plume responsible for the blue(+) sun observations in August 1831. The sequence of observations runs day by day from 8 August (a) to 12 August (e) and from 13 August (f) to 17 August (j). The more minor portion of the aerosol plume which lies outside the 30–45∘ N latitude band is not shown.
3.4 Aerosol transport velocity
Calculating the elapsed time between the earliest and latest progressively further westward blue(+) sun observations from 8 to 17 August 1831 in the latitude band 40 ± 2∘ N (Appendix A) yields a transport rate of about 0.97∘ (long.) h−1 for the leading edge of the aerosol plume and about 0.73∘ (long.) h−1 for its trailing edge (Fig. 7). Taking the mean transport rate of 0.85∘ (long.) h−1 at 40∘ N yields a linear velocity of about 20 m s−1.
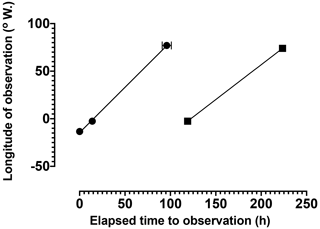
Figure 7Rate of westward transport of the leading (left) and trailing (right) edges of the aerosol plume, based on sources [A5], [A6], [A17] and [A18] (left) and sources [A6] and [A30] (right). Elapsed time is measured in hours from 18:00 LT (local time, UTC +1) in Sicily on 8 August 1831 (source [A5]).
3.5 Aerosol transport altitude
The prevailing winds in the mid-latitude upper troposphere in the Northern Hemisphere are westerly (Barry and Hall-McKim, 2014). As the aerosol plume transport took place from east to west, it must have been transported at an even higher altitude, above the tropopause. The source event type is most plausibly either a volcanic eruption or a very large forest or bush fire, either of which can inject aerosol into the stratosphere (Robock, 2000; Khaykin et al., 2020); super-eruptions with a VEI of 7 or more may even inject aerosol into the mesosphere (Costa et al., 2018). Given that a super-eruption in the vicinity of Sicily in August 1831 could not have gone un-recorded, however, it is reasonable to assume that the aerosol plume must have been transported in the stratosphere. An easterly stratospheric wind direction at around 40∘ N in July is also supported by zonal mean wind fields derived from twentieth-century data (Randel, 2003).
3.6 Aerosol optical depth and mass
In addition to altering the colour of the sun, the scattering and absorption of sunlight by atmospheric gases and aerosols dims its observed brightness (Bohren and Huffman, 2004). The sun has a visual magnitude (Schaefer, 1993). Adapting Stothers (1984a, b), the optical depth (τ) of an atmospheric aerosol (at zenith angle z=0∘, i.e. overhead) is related to the reduction in solar magnitude (ΔM) that it produces at an elevation angle (α) as follows:
where elevation angle (α) and zenith angle (z) are related as . Atmospheric refraction can be neglected for α> 15∘ (Stothers, 1984b; Schaefer, 1993).
Horvath et al. (1994) report that, due to the physiology of human colour perception, the colour of a blue(+) sun remains too bright to be perceived unless its light has been attenuated by a factor of at least 10−4 and that it is most readily perceived when its light is attenuated by a factor of between 10−5 and 10−6.6, equivalent to a reduction in magnitude of between ΔM= 12.5 and 16.5. Even if too bright to be perceived as such, a blue(+) sun will still be sufficiently reduced in magnitude to be able to be viewed with the naked eye without damage or discomfort at a reduction in magnitude of between, at the very least, about ΔM=3.4 (Stothers, 1984a, b) and, more likely, about ΔM=12 (Schaefer, 1993). At lower reductions in magnitude still, ΔM<3.4, the sun will be more normal in appearance and too bright to observe with the naked eye.
Thus, for suitable aerosol optical depth values (τ), three observational phases may be distinguished: at higher solar elevations, a sun of normal or near-normal appearance (for ΔM<3.4 and likely for some part of the range from ΔM=3.4 to ΔM=12); at lower solar elevations, a pale sun able to be viewed with the naked eye (for the remaining part of the range from ΔM=3.4 to ΔM=12); and at lower solar elevations still, a blue(+) sun able to be viewed with the naked eye (between ΔM=12.5 and ΔM=16.5).
These three observational phases are illustrated, for example, in two of the reports reproduced in Table 1 (sources [A10] and [A22]). It is noteworthy that the ratio between the brightness of the typical zenithal sun and a white object diffusely reflecting its light is about 80 000:1 (Minnaert, 1954). This presumably explains the otherwise paradoxical observation of blue(+) sunlight illuminating surfaces and objects when the sun is too bright to be observably blue(+) (source [A22]), as the associated attenuation factor of 10−4.9 is sufficiently close to meet the threshold requirement reported by Horvath et al. (1994).
Nine of the sources (Appendix A) report the local time at which a blue(+) sun was observed. The corresponding solar elevation angle (α) can be recovered from this local time, for example, using the National Oceanic and Atmospheric Administration (NOAA) Solar Calculator (available at https://gml.noaa.gov/grad/solcalc/, last access: 30 May 2021) (Appendix A). Therefore, using this solar elevation angle (α) and the range of reduction in solar magnitude associated with a blue(+) sun observation (ΔM=12.5 to ΔM=16.5), Eq. (1) yields a corresponding range of instantaneous aerosol optical depth values (τ) in each case (Fig. 8).
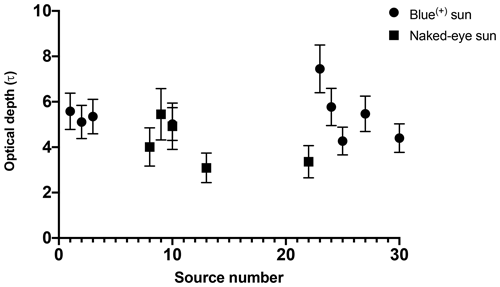
Figure 8Estimated instantaneous aerosol optical depth ranges. Those marked with a circle represent ranges derived from observations of a blue(+) sun, whereas those marked with a square represent ranges derived from observations of a naked-eye sun (either after having been observably blue(+) in the morning or before becoming observably blue(+) in the afternoon) (see Sect. 3.6).
Five of the sources (Appendix A) report the local time at which the sun was observed with the naked eye after having been observably blue(+) in the morning or before becoming observably blue(+) in the afternoon. The qualitative descriptions of the appearance of the sun in these latter reports, for example, as a “crystal globe” (source [A8]) or as “moon-like” (source [A10]), suggest the upper end of the 3.4 <ΔM <12 range in magnitude reduction, i.e. 8<ΔM <12. Likewise, recovering solar elevation angle (α) from local time and using this solar elevation angle (α) with the range of reduction in solar magnitude associated with such a naked-eye sun observation (ΔM=8 to ΔM=12), Eq. (1) yields a corresponding range of instantaneous aerosol optical depth values (α) in each case (Fig. 8).
Based on the observations reported in sources [A8]–[A30] between 8 and 17 August 1831, the mean instantaneous optical depth of the aerosol plume is estimated to be (Fig. 8).
Had the aerosol plume produced between 8 and 13 August 1831 been homogenous, however, it would have taken 6 d to pass over each site and reports of 6 consecutive days of identical blue(+) sun observations would have been expected. In fact, the number of consecutive days of blue(+) sun observations varied between 1 and 5 with a mean of about 1.8. Therefore, the aerosol plume cannot have been homogenous. We instead assume that it was sufficiently dense to produce observations of a blue(+) sun, with a mean optical depth , over only a fraction (approximately ) of its area. Although the remainder of the aerosol plume was evidently occasionally dense enough to produce ancillary observations of a pale sun (e.g. sources [A9], [A13], [A17] and [A18]), it will be neglected in comparison; taking into account background atmospheric Rayleigh scattering, Wullenweber et al. (2021) determined that in order for an aerosol which is capable of producing observations of a blue(+) sun to do so, it must have an optical depth τ>0.5.
Adapting Stothers (1984b, 1996), the mass M of a homogenous atmospheric aerosol over an area A is related to its optical depth (τ) (at z= 0∘) as follows:
where the aerosol particles all have radius r, density ρ and extinction efficiency Q. To account for the inhomogeneity in this case, we treat the area of the aerosol plume over which it was sufficiently dense to produce observations of a blue(+) sun. Using appropriate values for parameters r, ρ, Q, τ, A and f (Table 2), Eq. (2) yields an aerosol plume mass kg Tg.
This is a minimum value for the total mass of stratospheric aerosol produced by the source. It does not include the portion of the aerosol plume outside the 30–45∘ N latitude band, which might be approximately estimated to be 15 % (Fig. 2). Further, assuming that the four earlier observations of a blue(+) sun in the 30–45∘ N latitude band reported from North Africa on 3 August 1831 (source [A1]) and the north-eastern USA on 4 August 1831 (sources [A2]–[A4]) were caused by aerosol produced by the same source, they would be consistent with two smaller bodies of stratospheric aerosol having been produced between 31 July 1831 and 2 August 1831 (sources [A1]–[A3] in Fig. 8).
3.7 Aerosol source
The 1831 eruption of Ferdinandea (also known as “Campi Flegrei Mar Sicilia” and “Graham Island”) occurred about 50 km off the south-west coast of Sicily (Gemmellaro, 1831; Washington, 1909; Dean, 1980; Global Volcanism Program, 2013). Starting from a submarine base approximately 150 m b.s.l. (below sea level), it produced a volcanic island that first rose above sea level around 16 July 1831 and subsequently grew to about 60 m high and 2 km in circumference by the time the eruption ceased around 16 August 1831 (Dean, 1980; Spatola et al., 2018). Based on the close coincidence in place (“in the vicinity of Sicily”) and date (“between 31 July 1831 and 13 August 1831”), we identify the Ferdinandea eruption as the source of the stratospheric aerosol that was responsible for the blue(+) sun observations in August 1831. It is noteworthy that Riccò (1886) made a similar suggestion in the late nineteenth century.
The compositional dynamics of volcanic aerosol plumes can be complex (Mather et al., 2004). However, volcanogenic aerosol in the stratosphere is typically treated as being composed of sulfate droplets containing three-quarters sulfuric acid (H2SO4) and one-quarter water (H2O) (Zielinski, 1995; Toohey and Sigl, 2017). Therefore, in order to produce a minimum 10.1±2.5 Tg of stratospheric sulfate aerosol, the sulfur yield of the Ferdinandea eruption could not have been less than about 2.5±0.6 Tg.
4.1 The plausibility of the Ferdinandea eruption as the aerosol source
The Ferdinandea eruption is described as a small phreatomagmatic (“surtseyan”) eruption (Self et al., 1989). The remnant cone today lies underwater and has a volume of about 0.06 km3 (Spatola et al., 2018). The eruption has been assigned a VEI of 3, which is associated with a total volume of erupted tephra of the order of 0.1 km3 (Global Volcanism Program, 2013). Tephra typically has a density of about 1012 kg km−3 (Crosweller et al., 2012). In order to estimate a sulfur yield for this eruption from these parameters, the typical ocean island basalt affinity for the Sicily Straits Rift Zone (White et al., 2020) is assumed, as is the pre-eruptive melt sulfur content of 3000 ppm reported from Etna by Spilliaert et al. (2006), which marks the very upper end of the concentration range for this volcanic environment (Oppenheimer et al., 2011). These values yield a maximum sulfur yield of 0.3 Tg, which is about an order of magnitude smaller than our estimated minimum sulfur yield.
We hypothesize (hypothesis “H1”) that the release of the additional sulfur was the result of magma interacting with layers of sulfur-rich sedimentary deposits recently identified at the site of the Ferdinandea eruption (Spatola et al., 2018). These include Messinian evaporites (Spatola et al., 2018). Evaporitic sequences are typically associated with gypsum (CaSO4⋅2H2O), anhydrite (CaSO4) and halite (NaCl). The 1982 eruption of El Chichón, in Mexico, injected about 3.8 Tg of sulfur into the stratosphere (Krueger et al., 2008), as much as 2 orders of magnitude more than would have been expected on the basis of the volume and type of magma erupted alone (Luhr, 1984; Devine et al., 1984; Oppenheimer et al., 2011). The two possible sources for the additional sulfur have been identified as an evaporitic anhydrite-bearing sedimentary layer at the site of the El Chichón eruption and deeper subducted sulfide deposits (Rampino and Self, 1984; Duffield et al., 1984; Luhr, 2008). In the case of the Ferdinandea eruption, however, other sulfur-rich components may be relevant too. In some Sicilian Messinian evaporite contexts where hydrocarbons are present, sulfate-reducing microbial activity has resulted in conversion of the gypsum or anhydrite to more easily mobilizable hydrogen sulfide and native sulfur (Ziegenbalg et al., 2010). A hydrocarbon signature has been detected in gas emissions from an active fumarole field about 1 km from the submerged Ferdinandea cone, although concentrations of sulfur species were not determined (Coltelli et al., 2016).
This hypothesis (H1) is supported by eye-witness observations. During the eruption in July and August 1831, a very strong and unpleasant smell described as a “stink” of sulfur (“una puzza di zolfo”) or of sulfur and bitumen (“una puzza di zolfo e di bitume”) was reported in towns along or near the south-west coast of Sicily (Gemmellaro, 1831; Russo Ferrugia, 1831). The distance at which it was reported peaked at 100 km on 11 August 1831 (Russo Ferrugia, 1831), i.e. during the generation of the reconstructed stratospheric aerosol plume between 8 and 13 August 1831. Silver objects became tarnished at the same time (Gemmellaro, 1831; Russo Ferrugia, 1831), suggesting that sufficiently high atmospheric concentrations of hydrogen sulfide (and water vapour) reacted with the silver to form a blackened (“tarnished”) surface layer of silver sulfide (Inaba, 1996).
A VEI of 3 is associated with “possible” stratospheric injection rather than the “definite” stratospheric injection associated with a VEI of 4 (Newhall and Self, 1982; Global Volcanism Program, 2013). We hypothesize (hypothesis “H2”) that injection of the volcanic aerosol into the stratosphere by the Ferdinandea eruption was supported by favourable meteorological conditions. The 2018 eruption of Anak Krakatau, an island volcano on the rim of the Krakatau volcano, in Indonesia, was similar in style and magnitude to that of Ferdinandea (Table 3; Fig. 9a, b). It is likewise assigned a VEI of 3 (Global Volcanism Program, 2013). Crucially, sustained phreatomagmatic activity during the eruption produced a column with an updraught in which the vertical velocity was enhanced by convective instability (Prata et al., 2020). Thus, for 6 continuous days, a plume of positively buoyant aerosol was able to reach an altitude which varied between 16 and 18 km a.s.l. (above sea level), at times above the local tropopause at 16.8±0.8 km (Prata et al., 2020).
Table 3Comparison of the eruptions of Ferdinandea and Anak Krakatau. References: Dean (1980), Retalis and Cartalis (1997), Global Volcanism Project (2013), Spatola et al. (2018), Gouthier and Paris (2019), and Prata et al. (2020).
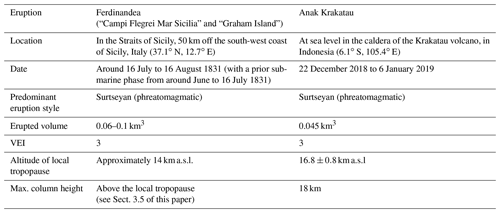
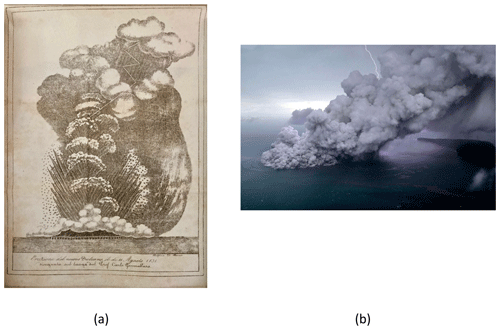
Figure 9(a). Sea-level sketch of the Ferdinandea eruption on 11 August 1831 drawn by Gemmellaro (1831). Characteristic features of phreatomagmatic activity are portrayed: pyroclastic material is being explosively ejected in successive cypress-tree- or cock's-tail-like forms at the base of a rising column of steam and ash (Francis and Oppenheimer, 2004); a “base surge” is visible. The column appears to be sheared downwind from the observer. A volcanic lightning discharge in the upper part of the eruption column is visible. (b) Photograph of the Anak Krakatau eruption taken from a light aircraft on 23 December 2018. (Image reproduced with permission: Nurul Hidayat/Antara Foto Agency/Reuters.) Characteristic features of phreatomagmatic activity are again visible (Francis and Oppenheimer, 2004) including a “base surge”. The column is inclined up and to the right of the observer. A volcanic lightning discharge is again visible.
Anticyclones with subsiding air typically inhibit deep convection in high summer in the central Mediterranean region, even though large amounts of convective instability may be present in the mid-troposphere above an inversion (Taszarek et al., 2018). The mean height of the tropopause over the south-eastern Mediterranean in the summer is approximately 14 km (Retalis and Cartalis, 1997). We hypothesize (H2) that phreatomagmatic activity during the Ferdinandea eruption was sufficiently sustained, at times, to produce a column with updraughts enhanced by environmental convective instability above any inversion, such that positively buoyant aerosol was able to breach the tropopause, similar to the Anak Krakatau eruption.
This hypothesis (H2) is also supported by eye-witness observations. Approaching the site of the eruption on 5 August 1831, i.e. just prior to the generation of the reconstructed stratospheric aerosol plume between 8 and 13 August 1831, Smythe (1831) reported from a distance of 55 km that: “… a stupendous column of white steam was observed, rising majestically far above the western horizon, splendidly illumined (sic) by the setting sun…”. At that time, on 6 August 1831, continuous episodes of violent “cypress-tree-like” phreatomagmatic explosions were separated by quiescent periods of 2–3 h (Smythe, 1831). By 11 August 1831, however, phreatomagmatic activity had significantly intensified: Gemmellaro (1831) reported that the continuous episodes lasted between 30 and 45 min (Fig. 9) and were separated by quiescent periods of only 2–3 min. Stratospheric injection may also have been achieved earlier in the eruption, even if it did not lead to the formation of an aerosol with the parameters necessary to produce observations of a blue(+) sun. On 22 July 1831, the eruption column reportedly subtended an angle of at least 20∘ at a distance of 50 km from the site of the eruption, suggesting that the (visible) column was at least 18 km high (Hoffmann, 1831; Symons et al., 1888).
Profiles of temperature, humidity and winds in the atmospheric column in the central Mediterranean in late July and early August 1831 will be required to test the hypothesis (H2) that environmental convective instability increased the height of the eruption column, permitting aerosol injection above the tropopause. Although no observations comparable to modern radiosonde ascents exist for this period, a proxy is provided by the recent extension of global atmospheric reanalysis datasets to the early nineteenth century, for example, the Twentieth Century Reanalysis (20CR) versions 2 and 3 (Compo et al., 2011; Slivinski et al., 2019).
Zonal mean wind fields derived from twentieth century data suggest that easterly wind velocity (40∘ N, July) would not be expected to exceed about 10 ms−1 below an altitude of approximately 20 km (30 hPa) in the low stratosphere and that a velocity of about 20 ms−1 would only be expected to be reached at an altitude of approximately 35 km (8 hPa) in the mid-stratosphere (Randel, 2003). A particular focus for this hypothesis testing will therefore examine whether the aerosol is likely to have reached only the low stratosphere, in which case the easterly aerosol transport velocity estimated in Sect. 3.4 (20 ms−1) would be inconsistent with the easterly wind velocity suggested for the lower stratosphere by the zonal mean wind field data (10 ms−1) by a factor of 2, or whether it could have reached the mid-stratosphere.
A reanalysis-based reconstruction of atmospheric circulation will also permit investigation of whether aerosol transport in different wind directions from the eruption site at different altitudes was responsible for the only blue(+) sun observation otherwise unaccounted for, which reportedly occurred on 9 August 1831 in Odessa, Ukraine (source [A7]; Fig. 5), as well as ancillary reports of unusual haze or fog elsewhere in the Mediterranean or Europe (e.g. sources [A1], [A5], [B8], [B13] and [B15]).
4.2 Comparison with independent datasets
Assuming that the sulfate aerosol plume was transported in the stratosphere, it could have been expected to produce occasional observations of a fiery twilight glow from 8 August 1831 onward. Such twilight glows are often referred to as “volcanic sunsets” and are characteristic of volcanogenic sulfate aerosols in the stratosphere (Meinel and Meinel, 1991). The stratospheric altitude is sufficiently high for the sulfate aerosol to continue to scatter (“reflect”) sunlight, so long as it is not blocked by tropospheric clouds, even when the sun is well below the horizon (Symons et al., 1888; Meinel and Meinel, 1991). Thus, stratospheric aerosol to the east of an observer may produce the observation of a twilight glow before dawn, whereas stratospheric aerosol to the west may produce the observation of a twilight glow after sunset.
Consistent with this expectation, nine of the sources that report observations of a blue(+) sun between 8 and 17 August 1831 also report observations of a fiery twilight glow whose locations move from east to west in conjunction with the sites of the blue(+) sun observations (Appendix A). In addition, a longer sequence of twilight glow observations was reported at Palermo between August and October 1831 (source [A5]). The sequence appears to have a periodicity of about 18 d. This suggests that, although the stratospheric aerosol plume only maintained the parameters necessary to produce observations of a blue(+) sun over about three-quarters of one circuit of Earth (path A–B–C–D in Fig. 10), it nevertheless remained dense enough thereafter to continue to produce observations of a twilight glow over two further circuits of Earth (path A–B–C–D–A in Fig. 10 and Fig. 11). A periodicity of 18 d is equivalent to a transport rate of 20∘ (long.) d−1 or about 0.84∘ (long.) h−1, very close to the aerosol plume transport rate (0.85∘ (long.) h−1) estimated in Sect. 3.4 on the basis of the blue(+) sun observations.
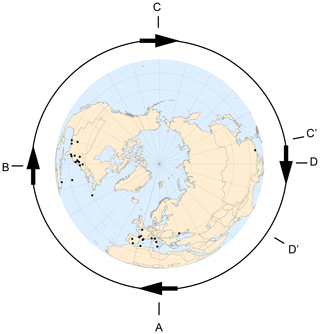
Figure 10Aerosol transport path A–B–C–D–A around Earth in 1831. The locations of the blue(+) sun observations reported in August 1831 (Appendix A) are shown. The last reported observation of a blue(+) sun occurred around 28 August 1831 in China (source [A31]) near point C', whereas the first observations where no blue(+) sun was reported occurred in India and Pakistan (sources [B1]–[B4]) near point D'.
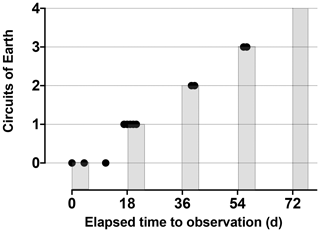
Figure 11Twilight glow observations reported in Palermo, Sicily, Italy, between August and October 1831 (source [A5]). Elapsed time is calculated in days from the twilight glow observation on 8 August 1831 (source [A5]). The periodicity of the observations is consistent with the aerosol responsible completing one circuit of Earth around transport path A–B–C–D–A (Fig. 10) in approximately 18 d.
Six of the null observation sources also report observations of a twilight glow in August 1831 (Appendix B), appearing to reflect the initial transport of the stratospheric aerosol plume or the two smaller precursory bodies of stratospheric aerosol (e.g. sources [B12], [B13] and [B14]) and/or the return of the aerosol plume after a first (e.g. sources [B5] and [B14]) or second circuit (e.g. source [B16]) of Earth.
As the stratospheric sulfate aerosol plume continued to be transported, it would have dispersed to form a more homogenous stratospheric sulfate aerosol. Assuming that sulfate aerosol typically has a stratospheric residence time with an e-folding timescale (i.e. the time taken to decline by a value ) of about 1 year (Robock, 2000), approximately 63 % of it would have returned to the troposphere after 1 year and 86 % after 2 years. Under the premise that the earliest possible sulfate deposition on the Greenland ice sheet from the Ferdinandea eruption could have taken place via a direct tropospheric route from July or August 1831 and that significant deposition via the stratospheric route could continue for no more than 2 years (Robock, 2000), it would be expected that an increase in sulfate deposition would be detected in Greenland ice cores between July or August 1831 and around August 1833. The magnitude of the expected increase should correspond to our estimated minimum sulfur yield for the Ferdinandea eruption of about 2.5±0.6 Tg.
Consistent with this expectation, Sigl et al. (2013) report a peak in sulfate deposition in a Greenland ice core between 1831.4±0.25 and 1833.7±0.25. Assuming a source eruption of Babuyan Claro, in the Philippines, Toohey and Sigl (2017) estimate that the sulfur yield of the eruption responsible for the peak was 12.98±3.41 Tg. However, if the source eruption had been located at a mid- or high-latitude site, instead of a low-latitude site, this estimate could be reduced by as much as 60 % (Toohey and Sigl, 2017). Presuming a source eruption at an unidentified site in the Northern Hemisphere (and using a different set of Greenland ice cores), Gao et al. (2008) estimate the sulfur yield of the eruption responsible for the peak to have been lower at about 4.2 Tg. Thus, our estimated minimum sulfur yield for the Ferdinandea eruption already represents about 60 % of the total eruption sulfur yield estimated by Gao et al. (2008).
Based on this close coincidence in the expected and actual sulfate deposition profile (and although more minor contributions from other sources cannot be ruled out), we identify the Ferdinandea eruption as the source of the climate forcing stratospheric sulfate aerosol in 1831.
One of the largest climate forcing volcanic eruptions of the nineteenth century took place in 1831 (Zielinski, 1995; Gao et al., 2008; Arfeuille et al., 2014; Toohey and Sigl, 2017). Here, we have used a newly compiled dataset of reported observations of a blue, purple and green sun in August 1831 to reconstruct the transport of a stratospheric aerosol plume from that eruption. Thus, we are able to constrain the location of the eruption to a mid-latitude site between 30 and 45∘ N. Those prior estimates of the mass of stratospheric sulfate aerosol responsible for the 1831 Greenland ice core sulfate deposition peaks which assumed a source eruption at a low-latitude site will, therefore, have been overstated (Zielinski, 1995; Arfeuille et al., 2014; Toohey and Sigl, 2017). For a given mass of stratospheric sulfate aerosol, Toohey et al. (2019) recently demonstrated that eruptions at mid- or high-latitudes can produce stronger hemispheric climate forcing than low-latitude eruptions.
Using additional reports where a blue, purple or green sun was not recorded, despite active observation, we are also able to constrain the longitude of the eruption and, hence, are able to identify it as the eruption of Ferdinandea, which took place about 50 km off the south-west coast of Sicily (37.1∘ N, 12.7∘ E) in July and August 1831. The eruption is assigned a VEI of 3 (Global Volcanism Program, 2013). Its modest magnitude has commonly caused it to be discounted or overlooked when identifying the likely source of the stratospheric sulfate aerosol in 1831 (Camuffo and Enzi, 1995; Zielinski, 1995; Robinson et al., 2001, Arfeuille et al., 2014; Toohey and Sigl, 2017). However, we argue here that the Ferdinandea eruption must be considered in the context of its geological and meteorological environment, rather than in isolation. We hypothesize (H1) that despite its modest magnitude, its magmatic system was nevertheless sufficient to trigger the release of sulfur from sedimentary deposits at the site of the eruption and (H2) that convective instability in the troposphere contributed to aerosol reaching the stratosphere, such that the Ferdinandea eruption did indeed result in the production of the stratospheric sulfate aerosol in 1831.
Our reconstruction of the transport of the stratospheric aerosol plume can be tested and improved upon with a search for further sources reporting the observation of a blue, purple or green sun in 1831. Our two hypotheses (H1, H2) as to the Ferdinandea eruption can be tested through further study of its magmatic system and geological context as well as with a reanalysis-based reconstruction of atmospheric circulation in July and August 1831. If we are correct, one of the largest climate forcing volcanic eruptions of the nineteenth century would effectively have been hiding in plain sight. This would arguably “lower the bar” for the types of eruptions capable of having a substantial climate forcing impact. This example serves as a useful reminder that VEI values were not intended to be reliably correlated with eruption sulfur yields unless supplemented with compositional analyses (Newhall and Self, 1982).
Analysis of reported observations of unusual atmospheric optical phenomena both in 1831 and in 1883 may support further investigation in a number of additional directions.
For example, the first observation of a blue(+) sun in the equivalent connected sequence following the onset of the most explosive phase of the 1883 Krakatau eruption occurred of the order of 1 d later (Symons et al., 1888). This suggests the rapid formation of a stratospheric aerosol whose size distribution is dominated by particles with a radius of the order of 0.5 µm (Table 2). The close coincidence between the substantial increase in the intensity of phreatomagmatic activity during the Ferdinandea eruption between 6 and 11 August 1831 and the first observation of a blue(+) sun in the connected sequence on 8 August 1831 (Sect. 4.1) suggests that a similarly rapid stratospheric aerosol formation occurred in that case too. Given that, for example, the stratospheric aerosol produced by the 1991 Pinatubo eruption took several months to grow to a typical size between 0.3 and 0.5 µm (Self et al., 1993), it would be interesting to consider the nature of the atypical microphysical processes that could be involved in these two rare cases.
Further, the durations of reported twilight glow observations in 1883 were used to constrain the altitude of the aerosol responsible (Symons et al., 1888; Meinel and Meinel, 1991). In the context of testing hypothesis H2, an analysis of the duration of the reported twilight glow observations mentioned in Sect. 4.2 as well as an analysis of a supplementary collected body of contemporary observations of twilight glows (and other unusual twilight phenomena) should provide independent evidence as to the altitude reached by the aerosol responsible in 1831.
Thus, the examples of 1831 and 1883 again underline that eye-witness accounts of historical geophysical events should not be neglected as a source of valuable scientific data (Guidoboni, 2010; Pyle and Barclay, 2020). The further before the nineteenth century an event took place, the more difficult it is likely to be to be able to collect primary sources reporting any associated observations of a blue, purple or green sun, or of other unusual atmospheric optical phenomena such as twilight glows. Nevertheless, where an adequate collection of primary sources can be collated, it can likewise be expected to be helpful in terms of, for example, reconstructing the circulatory state of the stratosphere (Hamilton and Sakazaki, 2018) or constraining the latitude, longitude and date of the source event which produced the aerosol responsible and the altitude at which it was present (Symons et al., 1888).
Table B2Continued.
All of the data necessary to repeat our analyses are found in the tables in this paper.
CG carried out the analysis presented in this study and drafted the paper. CK and SE provided guidance and expertise on the volcanological and geological contexts and critically reviewed the paper. DS provided guidance and expertise on the meteorological context (including proposing the pertinence of the convective instability mechanism) and critically reviewed the paper.
The contact author has declared that neither they nor their co-authors have any competing interests.
Publisher's note: Copernicus Publications remains neutral with regard to jurisdictional claims in published maps and institutional affiliations.
This article is part of the special issue “Interdisciplinary studies of volcanic impacts on climate and society”. It is not associated with a conference.
The authors would like to thank James Lequeux (Observatoire de Paris) for undertaking a search of the papers of François Arago at the Observatoire de Paris; Donatella Randazzo (Osservatorio Astronomico di Palermo) for assistance with a search of the archives of the Osservatorio Astronomico di Palermo; Marc Prohom Duran (Servei Meteorològic de Catalunya) for providing an extract from Quintana i Mari (1938) reproducing the observations of Francesc Bolòs (source [A6]); Julia Rodriguez Sanchez (University College London) for assistance with translation of materials from Catalan; Sabine Rodda for assistance with the translation of materials from German; Kai Deng (University College London) and Zheyu Tian (University College London) for assistance with translation of materials from Mandarin; Colin Graham (Gide Loyrette Nouel) for assistance with translation and historiographical interpretation of materials from Mandarin; Alessandro Aiuppa (Università degli Studi di Palermo), Attilio Sulli (Università degli Studi di Palermo), Sergio Calabrese (Università degli Studi di Palermo) and Walter D'Alessandro (Università degli Studi di Palermo) for valuable discussions regarding the geological context of the 1831 Ferdinandea eruption; and Michael Sigl (Universität Bern) for valuable discussions regarding sulfate deposition peaks in Greenland ice cores in 1831. The authors would also like to thank Fred Prata and two anonymous reviewers for the improvements to the paper which resulted from their comments. Christopher Garrison is also grateful for an E.A. Milne Travelling Fellowship grant from the Royal Astronomical Society (Burlington House, Piccadilly, London, W1J 0BQ, UK) to support a research visit to Sicily in 2018.
This paper was edited by Francis Ludlow and reviewed by Fred Prata and two anonymous referees.
Alexandria Gazette: “Meteorological Observations Taken at the Alexandria Museum, Aug. 1831, Friday, 2 September”, p. 1, 1831a.
Alexandria Gazette: “The sun on Saturday evening…”, Thursday, 18 August, p.1, 1831b.
Allgemeine Zeitung München: “Spanien, Madrid, 18 Aug…”, Samstag, 3 September, No. 246, p. 981, 1831a.
Allgemeine Zeitung München: “Pesth, 12 Aug…”, Montag, 22 August, No. 234, p. 936, 1831b.
Arago, F.: Communication to the 22 August 1831 meeting of the Académie des Sciences, in: Journal de Chimie Médicale, de Pharmacie et de Toxicologie, Tome VII, Chez Béchet Jeune, Paris, 1831.
Arago, F.: Notices scientifiques par M. Arago: Des Comètes en général, et en particulier, de la Comète qui doit reparaître en 1832 et dont la révolution est de 6 ans 3/4, in: Bureau des Longitudes Annuaire pour l'An 1832, Bachelier Père et Fils, Paris, 1832.
Arfeuille, F., Weisenstein, D., Mack, H., Rozanov, E., Peter, T., and Brönnimann, S.: Volcanic forcing for climate modeling: a new microphysics-based data set covering years 1600–present, Clim. Past, 10, 359–375, https://doi.org/10.5194/cp-10-359-2014, 2014.
Augsburger Ordinari Postzeitung: “Odessa, den 12 August, Im hiesigen Courrier liest man Folgendes…”, Sonntag, 4 September, No. 243, 1831.
Barry, R. G. and Hall-McKim, E. A.: Essentials of the Earth's climate system, Cambridge University Press, Cambridge, https://doi.org/10.1017/CBO9781139583909, 2014.
Basurah, H. M.: Auroras in Arabian Peninsula, J. King Saud. Univ. Sci., 22, 195–200, https://doi.org/10.4197/Sci.22-2.15, 2010.
Bayliffe, B. G. and Rawes, J. A.: Extracts from the Journal and Ship's Log of the Hon. Company's Ship Repulse (British Library Ref: L/MAR/B/51F.), available at: http://www.heicshipslogs.co.uk/logs/h022.htm#76 (last access: 30 May 2021), 2016.
Bohren, C. F. and Huffman, D. R.: Absorption and scattering of light by small particles, WILEY-VCH Verlag GmbH and Co. KGaA, Weinheim, https://doi.org/10.1002/9783527618156, 2004.
Bolòs, F.: Historial meteorològic i agricola de l'estació, 1831, in: Quintana i Marí, A., Assaig Sobre El Clima D'Olot, Servei Meteorologic De Catalunya, Notes D'Estudi, No. 69, Casa D'Assistència President Macià, Barcelona, 62–63, 1938.
Breen, P. H.: Nat Turner's Revolt: Rebellion and Response in Southampton County, Virginia, Ph. D. dissertation, University of Georgia, Athens, Georgia, 2005.
Brönnimann, S., Franke, J., Nussbaumer, S. U., Zumbühl, H. J., Steiner, D., Trachsel, M., Hegerl, G. C., Schurer, A., Worni, M., Malik, A., Flückiger, J., and Raible, C. C.: Last phase of the Little Ice Age forced by volcanic eruptions, Nat. Geosci., 12, 650–656, https://doi.org/10.1038/s41561-019-0402-y, 2019.
Bull, G.: Blue sun and moon, Meteorol. Mag, 80, 1–4, 1951.
Burnes, A.: Travels into Bokhara; Being the account of a journey from India to Cabool, Tartary and Persia; Also, Narrative of a Voyage on the Indus, from the sea to Lahore, second edition, volume 1, John Murray, London, 329 pp., 1834.
Cacciatore, N.: Osservazioni Meteorologiche Fatte Nel Real Osservatorio Di Palermo Nel Mese Di Luglio Dell'Anno 1831, in: Giornale di Scienze Lettere e Arti per La Sicilia, Tomo XXXV. – Anno IX, Luglio, Agosto e Settembre, Tipografia del Giornale Letterario, Palermo, 1831a.
Cacciatore, N.: Osservazioni Meteorologiche Fatte Nel Real Osservatorio Di Palermo Nel Mese Di Agosto Dell'Anno 1831, in: Giornale di Scienze Lettere e Arti per La Sicilia, Tomo XXXV. – Anno IX, Luglio, Agosto e Settembre, Tipografia del Giornale Letterario, Palermo, 1831b.
Cacciatore, N.: Osservazioni Meteorologiche Fatte Nel Real Osservatorio Di Palermo Nel Mese Di Settembre Dell'Anno 1831, in: Giornale di Scienze Lettere e Arti per La Sicilia, Tomo XXXVI. – Anno IX, Ottobre, Novembre e Dicembre, Tipografia del Giornale Letterario, Palermo, 1831c.
Cacciatore, N.: Osservazioni Meteorologiche Fatte Nel Real Osservatorio Di Palermo Nel Mese Di Ottobre Dell'Anno 1831, in: Giornale di Scienze Lettere e Arti per La Sicilia, Tomo XXXVI. – Anno IX, Ottobre, Novembre e Dicembre, Tipografia del Giornale Letterario, Palermo, 1831d.
Camuffo, D. and Enzi, S.: Impact of the clouds of volcanic aerosols in Italy during the last 7 centuries, Nat. Hazards, 11, 135–161, https://doi.org/10.1007/BF00634530, 1995.
Canton Register: “About a week previously, the sun, for several days…”, Thursday, 15 September, Vol. 4, No. 18, 1831.
Carpenter, L. G.: The Remarkable Sunsets, Nature, 30, 32–32, https://doi.org/10.1038/030032d0, 1884.
Coltelli, M., Cavallaro, D., D'Anna, G., D'Alessandro, A., Grassa, F., Mangano, G., Patanè, D., and Gresta, S.: Exploring the submarine Graham Bank in the Sicily Channel, Ann. Geophys.-Italy, 59, 2, https://doi.org/10.4401/ag-6929, 2016.
Colton, C.: Four Years in Great Britain, 1831–1835, Vol. 1, Harper and Brothers, New York, 312 pp., 1835.
Compo, G. P., Whitaker, J. S., Sardeshmukh, P. D., Matsui, N., Allan, R. J., Yin, X., Gleason, B. E., Vose, R. S., Rutledge, G., Bessemoulin, P., Brönnimann, S., Brunet, M., Crouthamel, R. I., Grant, A. N., Groisman, P. Y., Jones, P. D., Kruk, M. C., Kruger, A. C., Marshall, G. J., Maugeri, M., Mok, H. Y., Nordli, Ø., Ross, T. F., Trigo, R. M., Wang, X. L., Woodruff, S. D., and Worley, S. J.: The Twentieth Century Reanalysis Project, Q. J. Roy. Meteor. Soc., 137, 1–28, https://doi.org/10.1002/qj.776, 2011.
Costa, A. J., Suzuki, Y., and Koyaguchi, T.: Understanding the plume dynamics of explosive super-eruptions, Nat. Commun., 9, 654, https://doi.org/10.1038/s41467-018-02901-0, 2018.
Crosweller, H. S., Arora, B., Brown, S. K., Cottrell, E., Deligne, N. I., Guerrero, N. O., Hobbs, L., Kiyosugi, K., Loughlin, S. C., Lowndes, J., Nayembil, M., Siebert, L., Sparks, R. S. J., Takarada, S., and Venzke, E.: Global database on large magnitude explosive volcanic eruptions (LaMEVE), J. Appl. Volcanol., 1, 4, https://doi.org/10.1186/2191-5040-1-4, 2012.
Davy, J.: X. Further notice of the new volcano in the Mediterranean, Phil. Trans. R. Soc., 122, 251–253, https://doi.org/10.1098/rstl.1832.0011, 1832.
Dean, R. D.: Graham Island, Charles Lyell, and the Craters of Elevation Controversy, Isis, 71, 571–588, https://doi.org/10.1086/352593, 1980.
DeKay, J. E.: Sketches of Turkey in 1831 and 1832, J. and J. Harper, New York, 1833.
Devine, J. D., Sigurdsson, H., Davis, A. N., and Self, S.: Estimates of sulfur and chlorine yield to the atmosphere from volcanic eruptions and potential climatic effects, J. Geophys. Res.-Solid Earth, 89, 6309–6325, https://doi.org/10.1029/JB089iB07p06309, 1984.
Du Bois de Montpéreux, F.: Voyage autour du Caucase, chez les Tcherkesses et les Abkhases, en Colchide, en Géorgie en Arménie et en Crimée, Librairie de Gide, Paris, volume 1, 435 pp., 1839.
Duffield, W. A., Tilling, R. I., and Canul, R.: Geology of El Chichon volcano, Chiapas, Mexico, J. Volcanol. Geoth. Res., 20, 117–132, https://doi.org/10.1016/0377-0273(84)90069-6, 1984.
Dufour, L.: Communication to the 22 August 1831 meeting of the Académie des Sciences, in: Journal de Chimie Médicale, de Pharmacie et de Toxicologie, Tome VII, Chez Béchet Jeune, Paris, 1831.
Ehlers, K., Chakrabarty, R., and Moosmüller, H.: Blue moons and Martian sunsets, Appl. Optics, 53, 1808–1819, 2014.
El Correo: “Provincia de Avila…”, Viernes, 26 Agosto, No. 489, p. 4, 1831.
Estrella, C.: Fenómeno Meteorológico, in: El Correo, Miércoles, 24 August, No. 488, p. 3, 1831.
Francis, P. and Oppenheimer, C.: Volcanoes, Second edition, 521 pages, Oxford University Press, Oxford, ISBN 978-0-1992-5469-9, 2004.
Gao, C., Robock, A., and Ammann, C.: Volcanic forcing of climate over the past 1500 years: An improved ice core-based index for climate models, J. Geophys. Res.-Atmos., 113, D23111, https://doi.org/10.1029/2008JD010239, 2008.
Garrison, C. S., Kilburn, C. R. J., and Edwards, S. J.: The 1831 eruption of Babuyan Claro that never happened: has the source of one of the largest volcanic climate forcing events of the nineteenth century been misattributed?, J. Appl. Volcanol., 7, 8, https://doi.org/10.1186/s13617-018-0078-9, 2018.
Gazzetta di Firenze: “Granducato di Toscana…”, Martedi, 16 Agosto, No. 98, p. 3, 1831.
Gazzetta di Genova: “Fenomeni celesti…”, Sabato, 13 Agosto, No. 65, 1831.
Gemmellaro, C.: Relazione dei fenomeni del Nuovo Volcano sorto dal mare fra la costa di Sicilia e l'isola di Pantellaria nel mese di luglio 1831, Pastore, Catania, 1831.
Georgia Messenger: “Solar Phenomenon…”, Saturday, 27 August, Vol. 9, No. 25, p. 2, Macon, 1831.
Global Volcanism Program: Volcanoes of the World, v. 4.10.0 (14 May 2021), edited by: Venzke, E., Smithsonian Institution, Washington, 2013.
Gouhier, M. and Paris, R.: SO2 and tephra emissions during the December 22, 2018 Anak Krakatau flank-collapse eruption, Volcanica, 2, 91–103, https://doi.org/10.30909/vol.02.02.91103, 2019.
Groves, A.: Journal of a Residence at Baghdad during the years 1830 and 1831, James Nisbet, London, 1832.
Guidoboni, E.: History and Volcanology: Dialogue Overdue? The Case of a False Eruption in Medieval Italy, Eos, Trans. AGU, 91, 231, https://doi.org/10.1029/2010EO260002, 2010.
Hallowell, B.: Communication to the Washington National Intelligencer, in: Washington National Intelligencer, Monday, 24 August, 3 pp., 1831.
Hamilton, K. and Sakazaki, T.: Exploring the “prehistory” of the equatorial stratosphere with observations following major volcanic eruptions, Weather, 73, 154–159, https://doi.org/10.1002/wea.3043, 2018.
Harvey, A. W.: Communication to Sir David Brewster, 1839, in: British Association for the Advancement of Science (BAAS), Report of the Tenth Meeting (Held in Glasgow in August 1840), John Murray, London, 11 p., 1841.
Hazard's Register of Pennsylvania: 17 September, No. 194, Vol. 8, p. 192, 1831.
Hess, M.: “I was in the town of Trenton, State of Tennessee…”, 1831, in: Roberson, D. M.: Margaret Daveiss Hess – The First Woman to Plead A Court Case in the U.S., Ansearchin News – Tennessee Genealogical Magazine, 47, 2, 3–8, 2000.
Hoffman, F.: Intorno al nuovo vulcano presso la città di Sciacca: lettera del signor Federigo Hoffmann al ducca di Serradifalco, Presso La Reale Stamperia, Palermo, 13 p., https://doi.org/10.3931/e-rara-11173, 1831.
Horner, G. R. B.: An Account of the Cholera which occurred on board the United States' ship John Adams, 1831, Am. J. Med. Sci., Vol. IX, 257–263, Carey and Lea, Philadelphia, 1831.
Horvath, H., Metzig, G., Preining, O., and Pueschel, R. F.: Observation of a blue sun over new Mexico, U.S.A., on 19 April 1991, Atmos. Environ., 28, 621–630, https://doi.org/10.1016/1352-2310(94)90039-6, 1994.
Hough, F. B.: Results of a series of meteorological observations, made in obedience to instructions from the Regents of the University, at sundry academies in the state of New York, from 1826 to 1850 inclusive, p. xiv, Weed, Parsons and Company, Albany, 1855.
Inaba, M., Tarnishing of Silver: A short review, Victoria and Albert Museum Conservation Journal, No. 18, 1996.
Jacquemont, V.: Letters from India; Describing a journey in the British Dominions of India, Tibet, Lahore and Cashmere, Vol 1., edited by: Churton, E., London, 409 pp., 1834.
Journal de Genève: “Apparence Singuliere qu'a presente le soleil…”, Jeudi, 22 Septembre, p. 168, 1831.
Khaykin, S., Legras, B., Bucci, S., Sellitto, P., Isaksen, L., Tencé, F., Bekki, S., Bourassa, A., Rieger, L., Zawada, D., Jumelet, J., and Godin-Beekmann, S.: The 2019/20 Australian wildfires generated a persistent smoke-charged vortex rising up to 35 km altitude, Commun. Earth Environ., 1, 22, https://doi.org/10.1038/s43247-020-00022-5, 2020.
Kiessling, J.: Untersuchungen über Dämmerungserscheinungen: zur Erklärung der nach dem Krakatau-Ausbruch beobachteten atmosphärisch-optischen Störung, L. Voss, Hamburg und Leipzig, 172 pp., 1888.
Krueger, A., Krotkov, N., and Carn, S.: El Chichon: The genesis of volcanic sulfur dioxide monitoring from space, J. Volcanol. Geoth. Res., 175, 408–414, https://doi.org/10.1016/j.jvolgeores.2008.02.026, 2008.
La Mer, V. K. and Kerker, M.: Light Scattered by Particles, Sci. Am., 188, 69–77, 1953.
Laurent, P. E.: Recollections of a Classical Tour Through Various Parts of Greece, Turkey and Italy, Vol. 2, G. and W. B. Whittaker, London, 1821.
L'Abeille: “Nouvelle-Orleans, 17 Août. L'almanach de la Havane…', Jeudi, 18 Août, p. 1, New Orleans, 1831.
London and Paris Observer: “Arts and Sciences, Meetings of Scientific Bodies…”, 328, 28 August, p. 558, Paris, 1831.
London Morning Post: “New York, Aug. 18 – Solar Phenomenon…”, Tuesday, 27 September 1831.
Luhr, J. F., Carmichael, I. S. E., and Varekamp, J. C.: The 1982 eruptions of El Chichón Volcano, Chiapas, Mexico: Mineralogy and petrology of the anhydrite bearing pumices, J. Volcanol. Geoth. Res., 23, 69–108, https://doi.org/10.1016/0377-0273(84)90057-X, 1984.
Luhr, J. F.: Primary igneous anhydrite: Progress since its recognition in the 1982 El Chichón trachyandesite, 175, 394–407, https://doi.org/10.1016/j.jvolgeores.2008.02.016, 2008.
Mather, T. A., Pyle, D. M., and Oppenheimer, C.: Tropospheric Volcanic Aerosol, in: Volcanism and the Earth's Atmosphere, 189–212, https://doi.org/10.1029/139GM12, 2004.
Mathieu: Communication to the 29 August 1831 meeting of the Académie des Sciences, in: Mémorial Encyclopédique et Progressif des Connaissances Humaines, October, No. 10, 1831.
Meinel, A. and Meinel, M.: Sunsets, twilights, and evening skies, Cambridge University Press, Cambridge, 1991.
Mie, G.: Beiträge zur Optik trüber Medien, speziell kolloidaler Metallösungen, Ann. Phys., 330, 377–445, https://doi.org/10.1002/andp.19083300302, 1908.
Minnaert, M.: The Nature of Light and Colour in the Open Air, Dover Publications, 362 pp., 1954.
Mobile Register: “Singular Phenomena…”, 17 August 1831, in: American Journal of Science and Arts, January, Vol. XXI, p. 198, 1832.
Newhall, C. G. and Self, S.: The volcanic explosivity index (VEI) an estimate of explosive magnitude for historical volcanism, J. Geophys. Res.-Oceans, 87, 1231–1238, https://doi.org/10.1029/JC087iC02p01231, 1982.
New York Evening Post: “Pittsburgh (Penn.) Aug 12. Singular appearance…”, Wednesday, 17 August, No. 9050, p.2, 1831a.
New York Evening Post: “Atmospheric Phenomenon…”, Tuesday, 16 August, 9049, p. 2, 1831b.
Niles' Weekly Register: “The Solar Phenomena…”, in: Niles' Weekly Register, 1 October, Fourth Series, No. 5, Vol. V, Baltimore, 1831.
Oppenheimer, C., Scaillet, B., and Martin, R. S.: Sulfur degassing from volcanoes: source conditions, surveillance, plume chemistry and earth system impacts, Rev. Mineral Geochem., 73, 363–421, https://doi.org/10.2138/rmg.2011.73.13, 2011.
Penndorf, R.: On the phenomenon of the colored sun, especially the “blue” sun of September 1950, Geophysical Research Papers No. 20, Air Force Cambridge Research Center, Cambridge, Massachusetts, 1953.
Philosophical Magazine: Meteorological Observations for August 1831, July – December, Vol. X, 318–320, 1831.
Porch, W. M.: Blue moons and large fires, App. Opt., 28, 1778–1784, 1989.
Porter, D.: Constantinople and its Environs in a Series of Letters, Harper and Brothers, New York, 1835.
Poussou: Lettre de M. Poussou, supérieur de la mission des Lazaristes à Damas, à M. Etienne, procureur-général de la congregation des Lazaristes, 1831, in: Annales de la Propagation de la Foi, Tome Cinquante-Troisieme, 541 pp. et seq, L'Éditeur des Annales, Lyon, 1881.
Prata, A. T., Folch, A., Prata, A. J., Biondi, R., Brenot, H., Cimarelli, C., Corradini, S., Lapierre, J., and Costa, A.: Anak Krakatau triggers volcanic freezer in the upper troposphere, Sci. Rep., 10, 3584, https://doi.org/10.1038/s41598-020-60465-w, 2020.
Pyle, D. M. and Barclay, J.: Historical records of volcanic eruptions deserve more attention, Nature Reviews Earth and Environment, 1, 183–184, 2020.
Rampino, M. R. and Self, S.: Sulphur-rich volcanic eruptions and stratospheric aerosols, Nature, 310, 677–679, https://doi.org/10.1038/310677a0, 1984.
Randel, W. J.: Middle Atmosphere/Zonal Mean Climatology, in: Encyclopedia of atmospheric sciences, edited by: Holton, J. R., Curry, J. A., and Pyle, J. A., Academic Press, 1358–1365, https://doi.org/10.1016/B0-12-227090-8/00227-X, 2003.
Reid, W.: Communication to Sir David Brewster, 1839, in: British Association for the Advancement of Science (BAAS), Report of the Tenth Meeting (Held in Glasgow in August 1840), John Murray, London, 10 p., 1841.
Retalis, A. and Cartalis, C.: Definition of the tropopause height in the South-Eastern Mediterranean region, Toxicol. Environ. Chem., 58, 259–267, https://doi.org/10.1080/02772249709358415, 1997.
Riccò, A.: L'île Ferdinandea, le soleil bleu et les crépuscules rouges de 1831, in: Compt. Rend. Hebd. Ac. Sc., Vol. CII, 1060–1063, Paris, 1886.
Robertson, A., Overpeck, J., Rind, D., Mosley-Thompson, E., Zielinski, G., Lean, J., Koch, D., Penner, J., Tegen, I., and Healy, R.: Hypothesized climate forcing time series for the last 500 years, J. Geophys Res.-Atmos., 106, 14783–14803, https://doi.org/10.1029/2000JD900469, 2001.
Robock, A.: Volcanic eruptions and climate, Rev. Geophys., 38, 191–219, https://doi.org/10.1029/1998RG000054, 2000.
Roulin, F. D.: Communication to the 29 August 1831 meeting of the Académie des Sciences, in: Mémorial Encyclopédique et Progressif des Connaissances Humaines, October, No. 10, 1831.
Rozet, C. A.: Relation de la Guerre d'Afrique pendant les années 1830 et 1831, Volume 2, Chez Firman, Didot, Frères, Libraires, Paris, 1832.
Rozet, C. A.: Voyage dans la Régence d'Alger, 1, 155–157, Arthus Bertrand, Paris, 1833.
Russo Ferrugia, S.: Storia dell'Isola Ferdinandea, Trapani, 60 pp., 1831.
Savannah Republican: “Remarkable state of the Atmosphere…”, Thursday, 18 August, Vol. XXVIII, 168, p. 2, 1831.
Schaefer, B. E.: Astronomy and the limits of vision, Vistas Astron., 36, 311–361, https://doi.org/10.1016/0083-6656(93)90113-X, 1993.
Schomburgk, R. H.: The History of Barbados, Longman, Brown, Green and Longmans, London, 722 pp., 1848.
Schwabe, H. F.: Beobachtungen an Sonne, Planeten (Erdatmosphäre) und Kometen während des Jahres 1831, in: Archiv für die gesammte Naturlehre, edited by: Kastner, K. W. G., Johann Adam Stein, Nürnberg, 22, 393–395, 1831.
Self, S., Rampino, M. R., and Carr, M. J.: A reappraisal of the 1835 eruption of Cosigüina and its atmospheric impact, B. Volcanol., 52, 57–65, https://doi.org/10.1007/BF00641387, 1989.
Self, S., Zhao, J. X., Holasek, R. E., Torres, R. C., and King, A. J.: The atmospheric impact of the 1991 Mount Pinatubo eruption, in: Fire and Mud: Eruptions and Lahars of Mount Pinatubo, Philippine Institute of Volcanology and Seismology and the University of Washington Press, edited by: Newhall, C. G. and Punongbayan, R. S., Philippines, 1126 pp., 1993.
Sigl, M., McConnell, J. R., Layman, L., Maselli, O., McGwire, K., Pasteris, D., Dahl-Jensen, D., Steffensen, J. P., Vinther, B., Edwards, R., Mulvaney, R., and Kipfstuhl, S.: A new bipolar ice core record of volcanism from WAIS Divide and NEEM and implications for climate forcing of the last 2000 years, J. Geophys Res.-Atmos., 118, 1151–1169, https://doi.org/10.1029/2012JD018603, 2013.
Slivinski, L. C., Compo, G. P., Whitaker, J. S., Sardeshmukh, P. D., Giese, B. S., McColl, C., Allan, R., Yin, X., Vose, R., Titchner, H., Kennedy, J., Spencer, L. J., Ashcroft, L., Brönnimann, S., Brunet, M., Camuffo, D., Cornes, R., Cram, T. A., Crouthamel, R., Domínguez-Castro, F., Freeman, J. E., Gergis, J., Hawkins, E., Jones, P. D., Jourdain, S., Kaplan, A., Kubota, H., Blancq, F. L., Lee, T.-C., Lorrey, A., Luterbacher, J., Maugeri, M., Mock, C. J., Moore, G. W. K., Przybylak, R., Pudmenzky, C., Reason, C., Slonosky, V. C., Smith, C. A., Tinz, B., Trewin, B., Valente, M. A., Wang, X. L., Wilkinson, C., Wood, K., and Wyszyński, P.: Towards a more reliable historical reanalysis: Improvements for version 3 of the Twentieth Century Reanalysis system, Q. J. Roy. Meteor. Soc., 145, 2876–2908, https://doi.org/10.1002/qj.3598, 2019.
Smythe, G. W.: Views and Description of the late Volcanic Island off the coast of Sicily, Joseph Booker, London, 1831.
Spatola, D., Micallef, A., Sulli, A., Basilone, L., Ferreri, R., Basilone, G., Bonanno, A., Pulizzi, M., and Mangano, S.: The Graham Bank (Sicily Channel, central Mediterranean Sea): Seafloor signatures of volcanic and tectonic controls, Geomorphology, 318, 375–389, https://doi.org/10.1016/j.geomorph.2018.07.006, 2018.
Spilliaert, N., Allard, P., Meìtrich, N., and Sobolev, A. V.: Melt inclusion record of the conditions of ascent, degassing, and extrusion of volatile-rich alkali basalt during the powerful 2002 flank eruption of Mount Etna (Italy), J. Geophys. Res., 111, B04203, https://doi.org/10.1029/2005JB003934, 2006.
Stothers, R. B.: Mystery cloud of AD 536, Nature, 307, 344–345, https://doi.org/10.1038/307344a0, 1984a.
Stothers, R. B.: The Great Tambora Eruption in 1815 and Its Aftermath, Science, 224, 1191, https://doi.org/10.1126/science.224.4654.1191, 1984b.
Stothers, R. B.: The great dry fog of 1783, Climatic Change, 32, 79–89, https://doi.org/10.1007/BF00141279, 1996.
Symons, G. (Ed.), Judd, J. W., Strachey, R., Wharton, W. J. L., Evans, F. J., Russell, F. A. R., Archibald, D., and Whipple, G. M.: The Eruption of Krakatoa: And Subsequent Phenomena, Trübner and Company, London, 1888.
Taszarek, M., Brooks, H. E., Czernecki, B., Szuster, P., and Fortuniak, K.: Climatological aspects of convective parameters over Europe: A comparison of ERA-Interim and sounding data, J. Climate, 31, 4281–4308, https://doi.org/10.1175/JCLI-D-17-0596.1, 2018.
Taylor, T. G.: Result of astronomical observations made at the Honorable the East India Company's Observatory at Madras for the year 1831, Orphan Asylum Press, Madras, 1, 148 pp., 1832.
Tizzani, V. and Croce, G. M. (Eds.): Effemeridi Romane, Volume Primo: 1828–1860, ISBN 978-8-8492-2615-7, Gangemi Editore, Rome, 2015.
Toohey, M. and Sigl, M.: Volcanic stratospheric sulfur injections and aerosol optical depth from 500 BCE to 1900 CE, Earth Syst. Sci. Data, 9, 809–831, https://doi.org/10.5194/essd-9-809-2017, 2017.
Toohey, M., Krüger, K., Schmidt, H., Timmreck, C., Sigl, M., Stoffel, M., and Wilson, R.: Disproportionately strong climate forcing from extratropical explosive volcanic eruptions, Nat. Geosci., 12, 100–107, https://doi.org/10.1038/s41561-018-0286-2, 2019.
Van de Hulst, H. C.: Light scattering by small particles, Dover Publications, New York, 470 pp., ISBN 978-0-4866-4228-4, 1981.
Washington, H. S.: Submarine eruptions of 1831 and 1891 near Pantelleria, Am. J. Sci., s4–27, 131–150, https://doi.org/10.2475/ajs.s4-27.158.131, 1909.
Washington National Intelligencer: “Norfolk, Aug. 15 – We have all seen the sun of a dusky red or copper color…”, 19 August, p. 3, 1831.
Western Carolinian: “The sun had a very singular appearance…”, Monday, 22 August, Vol. XII, No. 585, p. 3, 1831.
White, J. C., Neave, D. A., Rotolo, S. G., and Parker, D. F.: Geochemical constraints on basalt petrogenesis in the Strait of Sicily Rift Zone (Italy): Insights into the importance of short lengthscale mantle heterogeneity, Chem. Geol., 545, 119650, https://doi.org/10.1016/j.chemgeo.2020.119650, 2020.
Whymper, E.: Coloured skies after an eruption of Cotopaxi, Science, ns–3, 99–100, https://doi.org/10.1126/science.ns-3.51.99, 1884.
Wilson, R.: The blue sun of 1950 September, Mon. Not. R. Astron. Soc., 111, 478–489, 1951.
Wullenweber, N., Lange, A., Rozanov, A., and von Savigny, C.: On the phenomenon of the blue sun, Clim. Past, 17, 969–983, https://doi.org/10.5194/cp-17-969-2021, 2021.
Yue, G. K., Poole, L. R., Wang, P.-H., and Chiou, E. W.: Stratospheric aerosol acidity, density, and refractive index deduced from SAGE II and NMC temperature data, J. Geophys. Res.-Atmos., 99, 3727–3738, https://doi.org/10.1029/93JD02989, 1994.
Zhang, D.: A compendium of Chinese meteorological records of the last 3000 years, Jiangsu Education House, Nanjing, 2004.
Ziegenbalg, S. B., Brunner, B., Rouchy, J. M., Birgel, D., Pierre, C., Böttcher, M. E., Caruso, A., Immenhauser, A., and Peckmann, J.: Formation of secondary carbonates and native sulphur in sulphate-rich Messinian strata, Sicily, Sediment. Geol., 227, 37–50, https://doi.org/10.1016/j.sedgeo.2010.03.007, 2010.
Zielinski, G. A.: Stratospheric loading and optical depth estimates of explosive volcanism over the last 2100 years derived from the Greenland Ice Sheet Project 2 ice core, J. Geophys. Res.-Atmos., 100, 20937–20955, https://doi.org/10.1029/95JD01751, 1995.