the Creative Commons Attribution 4.0 License.
the Creative Commons Attribution 4.0 License.
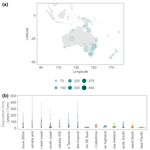
The Indo–Pacific Pollen Database – a Neotoma constituent database
Annika V. Herbert
Simon G. Haberle
Suzette G. A. Flantua
Ondrej Mottl
Jessica L. Blois
John W. Williams
Adrian George
Geoff S. Hope
The Indo–Pacific Pollen Database (IPPD) is the brainchild of the late professor Geoffrey Hope, who gathered pollen records from across the region to ensure their preservation for future generations of palaeoecologists. This noble aim is now being fulfilled by integrating the IPPD into the online Neotoma Paleoecology Database, making this compilation available for public use. Here we explore the database in depth and suggest directions for future research. The IPPD comprises 226 fossil pollen records, most postdating 20 ka but with some extending as far back as 50 ka or further. Over 80 % of the records are Australian, with a fairly even distribution between the different Australian geographical regions, with the notable exception being Western Australia, which is only represented by three records. The records are also well distributed in the modern climate space, with the largest gap being in drier regions due to preservation issues. However, many of the records contain few samples or have fewer than five chronology control points, such as radiocarbon, luminescence or Pb-210, for the younger sequences. Average deposition time for the whole database, counted as years per centimetre, is 64.8 yr cm−1, with 61 % of the records having a deposition time shorter than 50 yr cm−1. The slowest deposition time by geographical region occurs on Australia's east coast, while the fastest times are from the western Pacific. Overall, Australia has a slower deposition time than the rest of the Indo–Pacific region. The IPPD offers many exciting research opportunities to investigate past regional vegetation changes and associated drivers, including contrasting the impact of the first human arrival and European colonisation on vegetation. Examining spatiotemporal patterns of diversity and compositional turnover/rate of change, land cover reconstructions, and plant functional or trait diversity are other avenues of potential research, amongst many others. Merging the IPPD into Neotoma also facilitates inclusion of data from the Indo–Pacific region into global syntheses.
- Article
(4605 KB) - Full-text XML
-
Supplement
(301 KB) - BibTeX
- EndNote
The digital revolution and the advent of the internet transformed the landscape of fossil sample databases, enabling them to be shared with a global audience and heralding an era of scientific transparency and cooperation. The Neotoma Paleoecology Database represents a significant stride in this direction, offering a diverse range of records, including fossil pollen, charcoal, vertebrates, diatoms, ostracods, insects and geochronological data (Williams et al., 2018). This great resource has been used in hundreds of scientific publications to date, thus enabling the science of palaeoecology to take significant steps forward. In the era of open science, the importance of making scientific data publicly available cannot be overstated. Open access to data not only fosters transparency in research but also enables collaboration and innovation across disciplines and geographical boundaries (Wolkovich et al., 2012; Record et al., 2022). This approach is crucial in the field of palaeoecology, where comprehensive and accessible databases are key to understanding ecological histories and predicting future environmental changes (e.g. Lyver et al., 2015).
The journey of large databases in capturing fossil data spans several decades, beginning with pioneering efforts like Newell's palaeontological database in 1952 (Newell, 1952). The advent of spatial analyses of fossil data gave rise to significant developments, such as the European, North American and Latin American pollen databases, which were integral to the concept of a Global Pollen database, all of which are now constituent databases in Neotoma (Gajewski, 2008; Whitmore et al., 2005; Fyfe et al., 2009; Flantua et al., 2015). A notable extension of this endeavour was the Indo–Pacific Pollen Database (IPPD), originally compiled as part of the BIOME 6000 project. The aim of this project was to create biome maps for various periods (Prentice and Webb, 1998; Prentice et al., 1996; Pickett et al., 2004), namely the present day; the mid-Holocene, defined as 6000 ± 500 cal yr BP; and the Last Glacial Maximum (LGM), defined as 21 000 ± 1000 cal yr BP (Pickett et al., 2004). Subsequent enhancements involved the inclusion of full records, as opposed to time slices, with a focus on Australian records (Herbert and Harrison, 2016), the full incorporation of an Indo–Pacific compilation done by the late professor Geoffrey Hope (Hope et al., 1999) and modern samples (< 100 yr BP). The latter served as a training dataset for palaeoclimate reconstructions for comparison with palaeoclimate models (Herbert and Harrison, 2016). This version of the database has been used in multiple wide-ranging studies to date, including global overviews of rates of change processes (Mottl et al., 2021) and pollen taxonomic harmonisation processes (Birks et al., 2023). In addition, it has been used in regional studies, examining the climate dynamics of the last glacial period (Cadd et al., 2021; Herbert and Fitchett, 2021), the importance of Indigenous landscape and fire management (Mariani et al., 2022), biodiversity dynamics (Adeleye et al., 2021) and plant functional dynamics (Adeleye et al., 2023). This has added to the wealth of palaeoecological studies from this diverse region, such as Peter Kershaw's work on a southeast Australian pollen database (Kershaw et al., 1994; D'Costa and Kershaw, 1997). This is separate from the IPPD, but related, and contains many of the same sites, though with a focus on pre-European samples. Examples of other important work in this region that used their own pollen sample compilations include a floristic diversity study of South Pacific islands (Strandberg et al., 2024) and a study of human impact on the biodiversity of islands (Nogué et al., 2021).
Combined with the recent addition to Neotoma of other sites in the Southern Hemisphere through both the Latin American Pollen Database (including South American sites; Flantua et al., 2015) and the African Pollen Database (Ivory et al., 2020; Lézine et al., 2021) (both constituent databases in Neotoma), the addition of the IPPD to Neotoma suggests the enticing prospect of truly global palaeoecological studies. Here we explore this new constituent database in depth and suggest directions for future research.
Due to the recognised importance of openly accessible palaeoecological databases, fully integrating the IPPD into Neotoma (https://www.neotomadb.org/, last access: 23 October 2024) has been a long-term goal. Since 2021, a concerted effort has been made to fulfil this goal, which has involved getting hundreds of records in the right format and double-checking all the data and metadata against available publications, as well as removing duplicated entries and other data entry errors. Due to the original data no longer being available, some records had to be digitised from publications or theses. When this was done, the digitisation process and quality of results were carefully checked. Upon completing the digitisation, the stated pollen sum had to be 100 ± 10 % for the record to be accepted. Being such an underrepresented region, the integration also involved adding over 400 pollen taxa to Neotoma's taxonomic structure. This is a complicated procedure, as every single taxon needs to be validated against up-to-date and trusted sources. For most of these taxa, we used the Australian Plant Name Index (2024) (https://biodiversity.org.au/nsl/services/search/names, last access: 29 June 2024) and references therein.
Age models for all records have been reconstructed using Bacon (Blaauw and Christen, 2011) in R (R Core Team, 2022), with the versions depending on when the age model was constructed, as the database has been updated in stages. Radiocarbon dates were calibrated using either SHCal20 (Hogg et al., 2020) or SHCal13 (Hogg et al., 2013), again depending on when the age model was constructed.
For ease of presentation, we will here only present fossil records with more than two chronological control points and at least three stratigraphic levels, thus excluding many surface sample collections. The minimum number of chronological control points was chosen to be able to construct robust age–depth models. Chronological control points include dates obtained through radiocarbon dating, luminescence dating (either thermally or optically stimulated), and or Pb-210 for younger sequences. Plots were produced in R (R Core Team, 2022) to show different aspects of the IPPD (see the Supplement).
Modern observational climate data were taken from the CRU TS v4.07 gridded dataset at 0.5° grid cell resolution (Harris et al., 2020). The location of each record in the IPPD was then plotted against the full modern dataset, with the chosen climate variables being the mean annual precipitation (MAP), mean annual temperature (MAT), mean temperature of the coldest month (MTCO) and mean temperature of the warmest month (MTWA).
The IPPD holds records from a total of 530 sites, many of which contain only surface samples. A total of 226 stratigraphic records covering the Indo–Pacific region are presented here, which excludes surface samples and poorly dated records. These 226 records contain 9765 samples, with an average of 43 samples per record (SD: 51.3). There are 2461 unique taxa in the database, with an average of 56 taxa per record (SD: 31.9).
3.1 Spatial distribution
Over 83 % of the records are from Australia, with high representation from each Australian region, apart from Western Australia (Fig. 1a). Most pollen samples available through the IPPD consist of raw counts (58.4 %; Fig. 1b), and the rest are percentages, as well as a very small number of concentration counts. Just over a quarter of the records (27 %) have had to be digitised from publications or theses, due to the raw data no longer being available. Every region in the IPPD is represented mostly by sequences containing raw counts, which can therefore be used to verify the quality of the sequences from digitised sources from the same region (Figs. 1b and A2). There is also a good representation in the modern climate space, with the IPPD sites covering most of the available observed climate space, with the largest gap being in the driest regions due to preservation issues (Fig. 2). While there is a high number of sites from the southeastern coast of Australia (Appendix A; Fig. A1), the sites in the IPPD cover much of the available modern climate space despite this spatial bias (Fig. 2). This is invaluable when performing palaeoclimate reconstructions, as most methods use some form of space-for-time calibration that relies on networks of surface samples cross-referenced with current climate conditions (Herbert and Harrison, 2016; Chevalier et al., 2020).
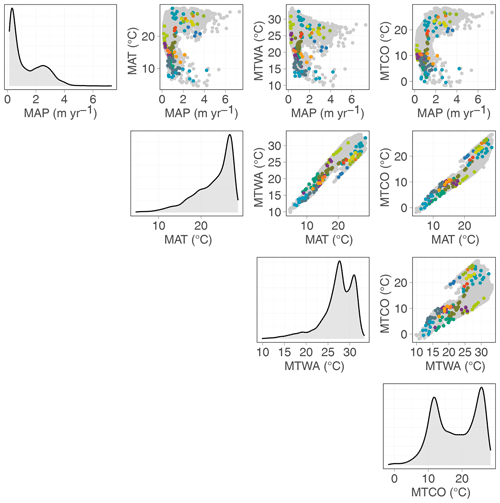
Figure 2Modern climate space covered by sites in the IPPD. Available observed climate space for the Indo–Pacific region in grey (from CRU TS v4.07; Harris et al., 2020); IPPD sites coloured according to geographical region, as presented in Fig. 1a. The line diagrams show the distribution of each climate variable, where MAP is mean annual precipitation, MAT is mean annual temperature, MTCO is mean temperature of the coldest month, and MTWA is mean temperature of the warmest month.
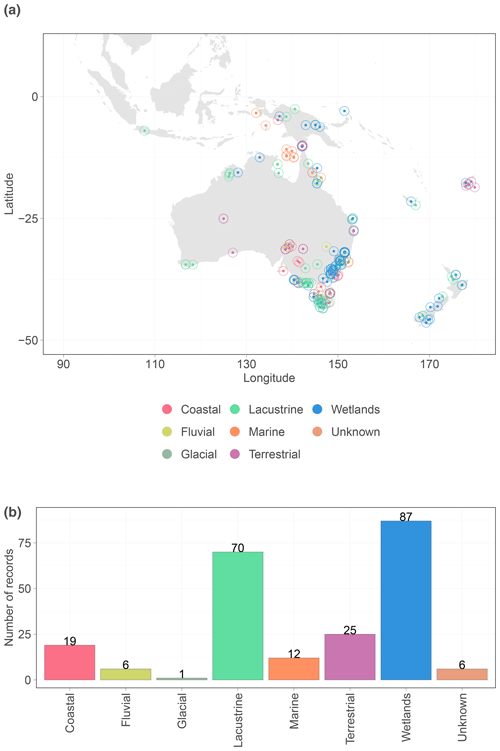
Figure 3(a) Geographical distribution of records by depositional environment; (b) number of records by depositional environment, binned into broader groupings. For detailed histograms, see Fig. A3 in the Appendix. For a full list of depositional environments, see Table S1 in the Supplement.
3.2 Depositional environment
There are a total of 33 different depositional environments represented in the database, with lakes and wetlands being the main source of records (73 % of sites; Fig. 3). A total of 31 % of the records come from lakes, natural or human-made, with a further 42 % coming from wetlands, such as swamps or fens.
3.3 Temporal distribution and chronological control
There are a total of 1637 chronological control points in the database, with an average of 7 points per record (SD: 7.8). A total of 52.6 % of the records contain fewer than five chronological points each, meaning that they may be poorly dated (Fig. 4a), depending on the number of levels associated with the site (Fig. 4b).
Some records (17 out of 226; e.g. 7.5 %) in the IPPD date back more than 50 ka, but most are younger than 20 ka (180 out of 226; e.g. 79.6 %). In addition, many sequences are not continuous and are composed of a few levels at the older end (Fig. 5). No clear geographical pattern in the distribution of short sequences or sequences with fewer than five chronological control points can be discerned from Fig. 4, meaning that the length of the sequences is not necessarily climate dependent. However, most of the older sequences are located close to the coast, where they are more likely to receive high rainfall, keeping the sediments wet and preserving the pollen grains (Fig. 5). Keeping the sediments waterlogged also decreases the risk of erosion, thereby benefiting the development of long sequences of well-preserved pollen (Delcourt and Delcourt, 1980; Lowe, 1982).
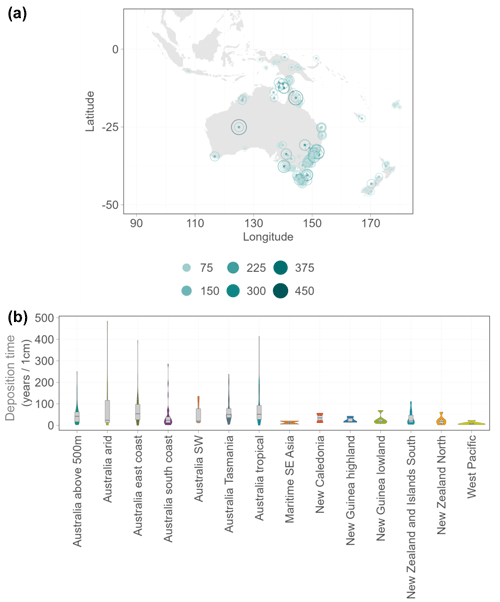
Figure 6(a) Geographical distribution of records by deposition time; (b) deposition time by geographical region, with the mean marked by a line. For a detailed histogram of the number of records by deposition time, see Fig. A6 in the Appendix.
3.4 Sedimentation rate and deposition time
For 61 % of the records, it takes less than 50 years to accumulate 1 cm of sediment. For 1.3 % of the records, it takes 300 years or more (Fig. 6 and Appendix A; Fig. A6). The average deposition time for the IPPD is 64.8 yr cm−1, but there is a very wide range, with the slowest regional sedimentation rate (largest deposition time; measured as the number of yr cm−1) on average being from the east coast of Australia. The region with the largest range of values is arid Australia, encompassing the highest and lowest rates overall. All regions in Australia except for one (south coast) have a slower average rate than any non-Australian region and also a much larger range (Fig. 6b). This could be due to lower average rainfall in Australia, which may inhibit biological activity in the lakes and wetlands that represent the majority of sites in the IPPD and slow down sediment accumulation. This is known to be an issue in arid and semi-arid areas (Ward and Larcombe, 2003), and with the rest of the regions represented in the IPPD being generally high-rainfall regions, this seems a likely explanation for the differences in rates. The average annual rainfall across all Australian IPPD sites is 1010 mm yr−1 and across all non-Australian IPPD sites 2505 mm yr−1. The reason Australian regions have larger ranges is probably due to the number of sites represented, with most sites in the database being Australian. The least represented Australian region, the southwest, also has the smallest range of any Australian region.
A large study of sedimentation rates in eastern North America found that on average mid-latitude (40–50° N) sites accumulated sediments faster (low number of yr cm−1) than low- or high-latitude sites (Webb and Webb, 1988). The authors attributed the rate in low-latitude sites to the fact that most of these sites were shallow and affected by dry seasons, making them prone to periods of erosion. This could certainly also be the case for many of the Australian sites, as it is common for lakes here to fluctuate in size or to periodically dry out completely. A more recent study focusing on the northeastern United States found that deposition times are more significantly related to depositional environment, sediment age and depth than to latitude, longitude or altitude (Goring et al., 2012). Here we have not examined the regional deposition times in that much detail, and it is therefore possible that the results differ between the regions due to the different depositional environments represented (Fig. 3).
The Indo–Pacific region has been poorly represented in global repositories in the past (Gajewski, 2008). Having this resource available through Neotoma for the first time will go some way to creating a truly global pollen database, and full integration of the IPPD into Neotoma complements other efforts focusing on getting more datasets into Neotoma from Africa (Lézine et al., 2021) and South America (Flantua et al., 2015). Neotoma operates with a constituent database structure (Williams et al., 2018), and sites within the IPPD can be viewed through Neotoma's informatics ecosystem (e.g. through the Explorer app at https://apps.neotomadb.org/explorer/, last access: 23 October 2024, or using the neotoma R package; Dominguez Vidaña and Goring, 2023). Going forward, Annika Herbert will serve as lead steward for the IPPD within Neotoma. Possible future analyses of our database include the examination of human impact on regional vegetation, contrasting first human arrival and colonisation (e.g. López-Sáez et al., 2014; Flantua et al., 2016); examining human cultures and food production based on anthropogenic indicators (e.g. Flantua and Hooghiemstra, 2023); or the assessment of rates of vegetation change during the Holocene (e.g. Mottl et al., 2021). With such a large database now publicly accessible and open-access workflows to process and standardise large compilations (Flantua et al., 2023; Vidaña and Goring, 2023), countless opportunities for further study are available, including global and hemispheric syntheses.
Considerable work has already been done using the IPPD or similar compilations. This work includes a rate of change analysis and rainfall seasonality reconstructions on the Australian part of the IPPD going back to the last glacial period (Cadd et al., 2021; Herbert and Fitchett, 2021) and Holocene plant trait analysis for the southeastern Australian part of the database (Adeleye et al., 2023). The latter is similar to a study conducted on European pollen samples by Veeken et al. (2022), as well as several other similar studies (e.g. van der Sande et al., 2019; Lacourse and Adeleye, 2022). This highlights the importance of regional coverage, as it can be used to perform comparative studies and examine differences or similarities with the rest of the world. Another example of this is the work by Mariani and colleagues (Mariani et al., 2016, 2017, 2022), using the pollen-to-vegetation conversion model REVEALS (Sugita, 2007a, b), which has been widely used in Europe and North America for the past decade or so (Gaillard et al., 2010; Sugita et al., 2010). Mariani's work represents the first use of this valuable model in Australia, and it has been instrumental in shedding light on Indigenous fire management practices and their importance (Mariani et al., 2022).
Future work using the IPPD could likewise take inspiration from methodologies employed in Europe and North America to conduct similar research in the Indo–Pacific region or use it to complete a global synthesis of a commonly used technique. Examples of the latter include large-scale quantitative climate reconstructions using various statistical techniques. Such studies are not commonly performed in the Indo–Pacific region (see Cook and van der Kaars, 2006), but the potential has been proven previously (Herbert and Harrison, 2016). Another possibility is to perform an in-depth study of deposition times, examining the influence of factors such as latitude, altitude, depositional environment, sediment age and depth, similar to studies in the United States (Webb and Webb, 1988; Goring et al., 2012). Fully accessible global palaeoecological databases make these types of studies possible, and with more sites being added every day, the possibilities for innovative research will likewise expand.
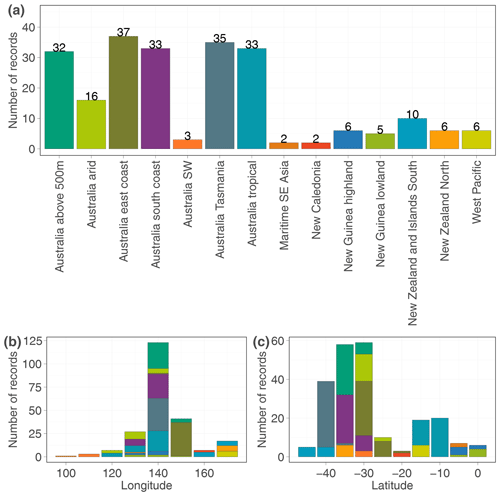
Figure A1(a) Number of records by geographical region; (b) number of records by latitude, with colours representing the regions from panel (a); and (c) number of records by longitude, with colours representing the regions from panel (a).
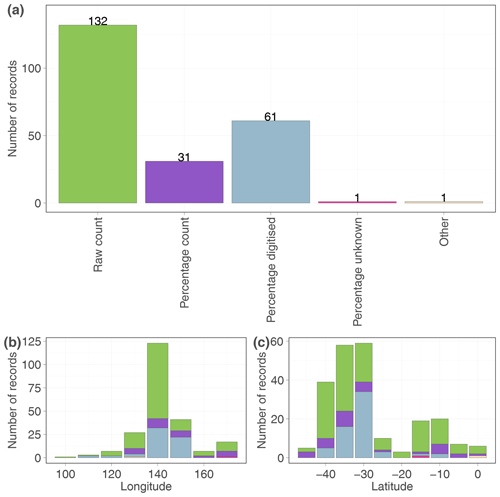
Figure A2(a) Number of records by count type; (b) number of records by latitude, with colours representing count type from panel (a); and (c) number of records by longitude, with colours representing count type from panel (a).
The code for all figures is available at our public GitHub repository at https://github.com/HOPE-UIB-BIO/IPPD_overview (last access: 26 October 2024) (https://doi.org/10.5281/zenodo.14003190, Mottl, 2024). All data were processed in R, using FOSSILPOL (https://github.com/HOPE-UIB-BIO/R-Fossilpol-package, last access: 7 November 2024, https://doi.org/10.5281/zenodo.14049214, Mottl and Flantua, 2024). Over half the sites are freely available through Neotoma (https://apps.neotomadb.org/explorer/?search=%7B%22metadata%22:%7B%22databaseId%22:4%7D%7D, last access: 23 October 2024), and the rest are in the process of being uploaded (to be completed by April 2025).
The supplement related to this article is available online at: https://doi.org/10.5194/cp-20-2473-2024-supplement.
AVH, SGH, SGAF and OM conceptualised the paper. AVH wrote the first draft and performed the data analysis. OM made the figures. All authors except GSH helped with data processing and reviewed the drafts. GSH compiled the first database and initialised the project.
The contact author has declared that none of the authors has any competing interests.
Publisher's note: Copernicus Publications remains neutral with regard to jurisdictional claims made in the text, published maps, institutional affiliations, or any other geographical representation in this paper. While Copernicus Publications makes every effort to include appropriate place names, the final responsibility lies with the authors.
The late Geoffrey Hope and Eric Grimm were integral in the early stages of this project, as well as in creating earlier versions of the database and the initial upload efforts. None of the current work would be possible without their efforts. The work of the data contributors, data stewards and IPPD/Neotoma community is gratefully acknowledged. The assistance of Matthew Jacques, Matthew Langer, Michael Rehani, Grace Roper and Jocelyn Wai-Yee Lam in preparing IPPD records for upload as undergraduate students at University of Wisconsin–Madison is gratefully acknowledged.
Annika V. Herbert has been supported by the Australian Research Council's Centre of Excellence in Biodiversity and Heritage. Ondrej Mottl and Suzette G. A. Flantua have been supported by the European Research Council (ERC) under the European Union's Horizon 2020 research and innovation programme (grant no. 741413) as part of the project called “HOPE Humans On Planet Earth – Long-term Impacts on biosphere dynamics”. Additionally, Suzette G. A. Flantua received support from the Trond Mohn Research Foundation (TMF) and the University of Bergen for the startup grant “TMS2022STG03” as part of the project called “Past, Present, and Future of Alpine Biomes Worldwide – PPF-Alpine”. Ondrej Mottl has been supported by the Czech Science Foundation PIF grant (grant no. 23-063861), by the Charles University Research Centre programme (grant no. UNCE/24/SCI/006), and by the Institutional Support for Science and Research of the Ministry of Education, Youth and Sports of the Czech Republic. Jessica L. Blois has been supported by the U.S. National Science Foundation Division of Earth Sciences (NSF EAR) (grant no. 1948579).
This paper was edited by Zhongshi Zhang and reviewed by two anonymous referees.
Adeleye, M. A., Mariani, M., Connor, S., Haberle, S. G., Herbert, A., Hopf, F., and Stevenson, J.: Long-term drivers of vegetation turnover in Southern Hemisphere temperate ecosystems, Global Ecol. Biogeogr., 30, 557–571, https://doi.org/10.1111/geb.13232, 2021.
Adeleye, M. A., Haberle, S. G., Gallagher, R., Andrew, S. C., and Herbert, A.: Changing plant functional diversity over the last 12,000 years provides perspectives for tracking future changes in vegetation communities, Nat. Ecol. Evol., 7, 224–235, https://doi.org/10.1038/s41559-022-01943-4, 2023.
Australian Plant Name Index: IBIS database, Centre for Australian National Biodiversity Research, Australian Government, Canberra, https://biodiversity.org.au/nsl/services/search/names, last access: 29 June 2024.
Birks, H. J. B., Bhatta, K. P., Felde, V. A., Flantua, S. G. A., Mottl, O., Haberle, S. G., Herbert, A., Hooghiemstra, H., Birks, H. H., Grytnes, J.-A., and Seddon, A. W. R.: Approaches to pollen taxonomic harmonisation in Quaternary palynology, Rev. Palaeobot. Palyno., 319, 104989, https://doi.org/10.1016/j.revpalbo.2023.104989, 2023.
Blaauw, M. and Christen, J. A.: Flexible paleoclimate age-depth models using an autoregressive gamma process, Bayesian Anal., 6, 457–474, https://doi.org/10.1214/11-BA618, 2011.
Cadd, H., Petherick, L., Tyler, J., Herbert, A., Cohen, T. J., Sniderman, K., Barrows, T. T., Fulop, R. H., Knight, J., Kershaw, A. P., Colhoun, E. A., and Harris, M. R. P.: A continental perspective on the timing of environmental change during the last glacial stage in Australia, Quaternary Res., 102, 5–23, https://doi.org/10.1017/qua.2021.16, 2021.
Chevalier, M., Davis, B. A. S., Heiri, O., Seppä, H., Chase, B. M., Gajewski, K., Lacourse, T., Telford, R. J., Finsinger, W., Guiot, J., Kühl, N., Maezumi, S. Y., Tipton, J. R., Carter, V. A., Brussel, T., Phelps, L. N., Dawson, A., Zanon, M., Vallé, F., Nolan, C., Mauri, A., de Vernal, A., Izumi, K., Holmström, L., Marsicek, J., Goring, S., Sommer, P. S., Chaput, M., and Kupriyanov, D.: Pollen-based climate reconstruction techniques for late Quaternary studies, Earth-Sci. Rev., 210, 103384, https://doi.org/10.1016/j.earscirev.2020.103384, 2020.
Cook, E. J. and van der Kaars, S.: Development and testing of transfer functions for generating quantitative climatic estimates from Australian pollen data, J. Quaternary Sci., 21, 723–733, https://doi.org/10.1002/jqs.1076, 2006.
D'Costa, D. and Kershaw, A. P.: An expanded recent pollen database from south-eastern Australia and its potential for refinement of palaeoclimatic estimates, Aust. J. Bot., 45, 583–605, https://doi.org/10.1071/BT96046, 1997.
Delcourt, P. A. and Delcourt, H. R.: Pollen preservation and Quaternary environmental history in the southeastern United States, Palynology, 4, 215–231, https://doi.org/10.1080/01916122.1980.9989209, 1980.
Flantua, S. G. A. and Hooghiemstra, H.: Anthropogenic pollen indicators: Global food plants and Latin American human indicators in the pollen record, Scientific Data, 10, 721, https://doi.org/10.1038/s41597-023-02613-1, 2023.
Flantua, S. G. A., Hooghiemstra, H., Grimm, E. C., Behling, H., Bush, M. B., González-Arango, C., Gosling, W. D., Ledru, M.-P., Lozano-García, Maldonado, A., Prieto, A. R., Rull, V., and Van Boxel, J. H.: Updated site compilation of the Latin American Pollen Database, Rev. Palaeobot. Palyno., 223, 104–115, https://doi.org/10.1016/j.revpalbo.2015.09.008, 2015.
Flantua, S. G. A., Hooghiemstra, H., Vuille, M., Behling, H., Carson, J. F., Gosling, W. D., Hoyos, I., Ledru, M. P., Montoya, E., Mayle, F., Maldonado, A., Rull, V., Tonello, M. S., Whitney, B. S., and González-Arango, C.: Climate variability and human impact in South America during the last 2000 years: synthesis and perspectives from pollen records, Clim. Past, 12, 483–523, https://doi.org/10.5194/cp-12-483-2016, 2016.
Flantua, S. G. A., Mottl, O., Felde, V. A., Bhatta, K. P., Birks, H. H., Grytnes, J.-A., Seddon, A. W. R., and Birks, H. J. B.: A guide to the processing and standardization of global palaeoecological data for large-scale syntheses using fossil pollen, Global Ecol. Biogeogr., 32, 1377–1394, https://doi.org/10.1111/geb.13693, 2023.
Fyfe, R. M., de Beaulieu, J.-L., Binney, H., Bradshaw, R. H. W., Brewer, S., Le Flao, A., Finsinger, W., Gaillard, M.-J., Giesecke, T., Gil-Romera, G., Grimm, E. C., Huntley, B., Kunes, P., Kühl, N., Leydet, M., Lotter, A. F., Tarasov, P. E., and Tonkov, S.: The European Pollen Database: past efforts and current activities, Veg. Hist. Archaeobot., 18, 417–424, https://doi.org/10.1007/s00334-009-0215-9, 2009.
Gaillard, M.-J., Sugita, S., Mazier, F., Trondman, A.-K., Broström, A., Hickler, T., Kaplan, J. O., Kjellström, E., Kokfelt, U., Kuneš, P., Lemmen, C., Miller, P., Olofsson, J., Poska, A., Rundgren, M., Smith, B., Strandberg, G., Fyfe, R., Nielsen, A. B., Alenius, T., Balakauskas, L., Barnekow, L., Birks, H. J. B., Bjune, A., Björkman, L., Giesecke, T., Hjelle, K., Kalnina, L., Kangur, M., van der Knaap, W. O., Koff, T., Lagerås, P., Latałowa, M., Leydet, M., Lechterbeck, J., Lindbladh, M., Odgaard, B., Peglar, S., Segerström, U., von Stedingk, H., and Seppä, H.: Holocene land-cover reconstructions for studies on land cover-climate feedbacks, Clim. Past, 6, 483–499, https://doi.org/10.5194/cp-6-483-2010, 2010.
Gajewski, K.: The Global Pollen Database in biogeographical and palaeoclimatic studies, Prog. Phys. Geog., 32, 379–402, https://doi.org/10.1177/0309133308096029, 2008.
Goring, S., Williams, J. W., Blois, J. L., Jackson, S. T., Paciorek, C. J., Booth, R. K., Marlon, J. R., Blaauw, M., and Christen, J. A.: Deposition times in the northeastern United States during the Holocene: establishing valid priors for Bayesian age models, Quaternary Sci. Rev., 48, 54–60, https://doi.org/10.1016/j.quascirev.2012.05.019, 2012.
Harris, I., Osborn, T. J., Jones, P., and Lister, D. H.: Version 4 of the CRU TS monthly high-resolution gridded multivariate climate dataset, Sci. Data, 7, 1–18, https://doi.org/10.1038/s41597-020-0453-3, 2020.
Herbert, A. V. and Fitchett, J. M.: Quantifying late Quaternary Australian rainfall seasonality changes using the Poaceae: Asteraceae pollen ratio, Quaternary Res., 102, 24–38, https://doi.org/10.1017/qua.2020.18, 2021.
Herbert, A. V. and Harrison, S. P.: Evaluation of a modern-analogue methodology for reconstructing Australian palaeoclimate from pollen. Rev. Palaeobot. Palyno., 226, 65–77, https://doi.org/10.1016/j.revpalbo.2015.12.006, 2016.
Hogg, A. G., Hua, Q., Blackwell, P. G., Niu, M., Buck, C. E., Guilderson, T. P., Heaton, T. J., Palmer, J. G., Reimer, P. J., Reimer, R. W., Turney, C. S. M., and Zimmerman, S. R. H.: SHCal13 Southern Hemisphere calibration, 0–50,000 years cal BP, Radiocarbon, 55, 1889–1903, https://doi.org/10.2458/azu_js_rc.55.16783, 2013.
Hogg, A. G., Heaton, T. J., Hua, Q., Palmer, J. G., Turney, C. S. M., Southon, J., Bayliss, A., Blackwell, P. G., Boswijk, G., Bronk Ramsey, C., Pearson, C., Petchey, F., Reimer, P., Reimer, R., and Wacker, L.: SHCal20 Southern Hemisphere calibration, 0–55,000 years cal BP, Radiocarbon, 62, 759–778, https://doi.org/10.1017/RDC.2020.59, 2020.
Hope, G. S., O'Dea, D., and Southern, W.: Holocene vegetation histories in the Western Pacific – alternative records of human impact, in: Le Pacifique de 5000 à 2000 avant le present: suppléments à l'histoire d'une colonisation, edited by: Lilley, I. and Galipaud, J.-C., IRD, Paris, France, 387–404, ISBN 270991431X, 1999.
Ivory, S. J., Lézine, A.-M., Grimm, E., and Williams, J.: Relaunshing the African Pollen Database: Abrupt change in climate and ecosystems, Past Global Changes Magazine, Past Plant Diversity and Conservation, 28, 26–28, https://doi.org/10.22498/pages.28.1.26, 2020.
Kershaw, A. P., Bulman, D., and Busby, J. R.: An examination of modern and pre-European settlement pollen samples from southeastern Australia – assessment of their application to quantitative reconstruction of past vegetation and climate, Rev. Palaeobot. Palyno., 82, 83–96, https://doi.org/10.1016/0034-6667(94)90021-3, 1994.
Lacourse, T. and Adeleye, M. A.: Climate and species traits drive changes in Holocene forest composition along an elevation gradient in Pacific Canada, Front. Ecol. Evol., 10, 838545, https://doi.org/10.3389/fevo.2022.838545, 2022.
Lézine, A.-M., Ivory, S. J., Gosling, W. D., and Scott, L.: The African Pollen Database (APD) and tracing environmental change: State of the Art, in: Quaternary Vegetation Dynamics, edited by: Runge, J., Gosling, W., Lézine, A.-M., and Scott, L., CRC Press, London, UK, https://doi.org/10.1201/9781003162766, 5–12, 2021.
López-Sáez, J. A., Abel-Schaad, D., Pérez-Díaz, S., Blanco-González, A., Alba-Sánchez, F., Dorado, M., Ruiz-Zapata, B., Gil-García, M. J., Gómez-González, C., and Franco-Múgica, F.: Vegetation history, climate and human impact in the Spanish Central System over the last 9000 years, Quatern. Int., 353, 98–122, https://doi.org/10.1016/j.quaint.2013.06.034, 2014.
Lowe, J. J.: Three Flandrian pollen profiles from the Teith Valley, Perthshire, Scotland. II. Analysis of deteriorated pollen, New Phytol., 90, 371–385, https://doi.org/10.1111/j.1469-8137.1982.tb03268.x, 1982.
Lyver, P. O., Wilmshurst, J. M., Wood, J. R., Jones, C. J., Fromont, M., Bellingham, P. J., Stone, C., Sheehan, M., and Moller, H.: Looking back for the future: local knowledge and palaeoecology inform biocultural restoration of coastal ecosystems in New Zealand, Hum. Ecol., 43, 681–695, https://doi.org/10.1007/s10745-015-9784-7, 2015.
Mariani, M., Connor, S. E., Theuerkauf, M., Kuneš, P., and Fletcher, M.-S.: Testing quantitative pollen dispersal models in animal-pollinated vegetation mosaics: An example from temperate Tasmania, Australia, Quaternary Sci. Rev., 154, 214–225, https://doi.org/10.1016/j.quascirev.2016.10.020, 2016.
Mariani, M., Connor, S. E., Fletcher, M.-S., Theuerkauf, M., Kuneš, P., Jacobsen, G., Saunders, K. M., and Zawadzki, A.: How old is the Tasmanian cultural landscape? A test of landscape openness using quantitative land-cover reconstructions, J. Biogeogr., 44, 2410–2420, https://doi.org/10.1111/jbi.13040, 2017.
Mariani, M., Connor, S. E., Theuerkauf, M., Herbert, A., Kunes, P., Bowman, D., Fletcher, M.-S., Head, L., Kershaw, A. P., Haberle, S. G., Stevenson, J., Adeleye, M., Cadd, H., Hopf, F., and Briles, C.: Disruption of cultural burning promotes shrub encroachment and unprecedented wildfires, Front. Ecol. Environ., 20, 292–300, https://doi.org/10.1002/fee.2395, 2022.
Mottl, O.: Code for: “The Indo-Pacific Pollen Database – a Neotoma constituent database”, Zenodo [data set], https://doi.org/10.5281/zenodo.14003190, 2024.
Mottl, O. and Flantua, S. G. A.: FOSSILPOL: The workflow to process global palaeoecological pollen data (v1.0.1-20241107), Zenodo [code], https://doi.org/10.5281/zenodo.14049214, 2024.
Mottl, O., Flantua, S. G. A., Bhatta, K. P., Felde, V. A., Giesecke, T., Goring, S., Grimm, E. C., Haberle, S., Hooghiemstra, H., Ivory, S., Kunes, P., Wolters, S., Seddon, A. W. R., and Williams, J. W.: Global acceleration in rates of vegetation change over the past 18,000 years, Science, 372, 860–864, https://doi.org/10.1126/science.abg1685, 2021.
neotomadb.org: Neotoma Paleoecology Database, https://www.neotomadb.org/, last access: 23 October 2024.
Newell, N. D.: Periodicity in invertebrate evolution, J. Paleontol., 26, 371–385, 1952.
Nogué, S., Santos, A. M. C., Birks, H. J. B., Björck, S., Castilla-Beltrán, A., Connor, S., de Boer, E. J., de Nascimento, L., Felde, V. A., Fernández-Palacios, J. M., Froyd, C. A., Haberle, S. G., Hooghiemstra, H., Ljung, K., Norder, S. J., Peñuelas, J., Prebble, M., Stevenson, J., Whittaker, R. J., Willis, K. J., Wilmshurst, J. M., and Steinbauer, M. J.: The human dimension of biodiversity changes on islands, Science, 372, 488–491, https://doi.org/10.1126/science.abd6706, 2021.
Pickett, E. J., Harrison, S. P., Hope, G., Harle, K., Dodson, J. R., Kershaw, A. P., Prentice, I. C., Backhouse, J., Colhoun, E. A., D'Costa, D., Flenley, J., Grindrod, J., Haberle, S., Hassell, C., Kenyon, C., Macphail, M., Martin, H., Martin, A. H., McKenzie, M., Newsome, J. C., Penny, D., Powell, J., Raine, J. I., Southern, W., Stevenson, J., Sutra, J.-P., Thomas, I., van der Kaars, S., and Ward, J.: Pollen-based reconstructions of biome distributions for Australia, Southeast Asia and the Pacific (SEAPAC region) at 0, 6000 and 18,000 14C yr BP, J. Biogeogr., 31, 1381–1444, https://doi.org/10.1111/j.1365-2699.2004.01001.x, 2004.
Prentice, I. C. and Webb, T.: BIOME 6000: reconstructing global mid-Holocene vegetation patterns from palaeoecological records, J. Biogeogr., 25, 997–1005, https://doi.org/10.1046/j.1365-2699.1998.00235.x, 1998.
Prentice, I. C., Guiot, J., Huntley, B., Jolly, D., and Cheddadi, R.: Reconstructing biomes from palaeoecological data: a general method and its application to European pollen data at 0 and 6 ka, Clim. Dynam., 12, 185–194, https://doi.org/10.1007/BF00211617, 1996.
R Core Team: R: A language and environment for statistical computing, R foundation for statistical computing, Vienna, Austria, https://www.r-project.org/ (last access: 21 October 2024), 2022.
Record, S., Jarzyna, M. A., Hardiman, B., and Richardson, A. D.: Open data facilitate resilience in science during the COVID-19 pandemic, Front. Ecol. Environ., 20, 76, https://doi.org/10.1002/fee.2468, 2022.
Strandberg, N. A., Steinbauer, M. J., Walentowitz, A., Gosling, W. D., Fall, P. L., Prebble, M., Stevenson, J., Wilmshurst, J. M., Sear, D. A., Langdon, P. G., Edwards, M. E., and Nogué, S.: Floristic homogenization of South Pacific islands commenced with human arrival, Nat. Ecol. Evol., 8, 511–518, https://doi.org/10.1038/s41559-023-02306-3, 2024.
Sugita, S.: Theory of quantitative reconstruction of vegetation I: pollen from large sites REVEALS regional vegetation composition, Holocene, 17, 229–241, https://doi.org/10.1177/0959683607075837, 2007a.
Sugita, S.: Theory of quantitative reconstruction of vegetation II: all you need is LOVE, Holocene, 17, 243–257, https://doi.org/10.1177/0959683607075838, 2007b.
Sugita, S., Parshall, T., Calcote, R., and Walker, K.: Testing the Landscape Reconstruction Algorithm for spatially explicit reconstruction of vegetation in northern Michigan and Wisconsin, Quaternary Res., 74, 289–300, https://doi.org/10.1016/j.yqres.2010.07.008, 2010.
Van der Sande, M. T., Gosling, W., Correa-Metrio, A., Prado-Junior, J., Poorter, L., Oliveira, R. S., Mazzei, L., and Bush, M. B.: A 7000-year history of changing plant trait composition in an Amazonian landscape; the role of humans and climate, Ecol. Lett., 22, 925–935. https://doi.org/10.1111/ele.13251, 2019.
Veeken, A., Santos, M. J., McGowan, S., Davies, A. L., and Schrodt, F.: Pollen-based reconstruction reveals the impact of the onset of agriculture on plant functional trait composition, Ecol. Lett., 25, 1937–1951, https://doi.org/10.1111/ele.14063, 2022.
Vidaña, S. D. and Goring, S. J.: neotoma2: An R package to access data from the Neotoma Paleoecology Database, Journal of Open Source Software, 8, 5561, https://doi.org/10.21105/joss.05561, 2023.
Ward, I. and Larcombe, P.: A process-orientated approach to archaeological site formation: application to semi-arid Northern Australia, J. Archaeol. Sci., 30, 1223–1236, https://doi.org/10.1016/S0305-4403(02)00253-4, 2003.
Webb, R. S. and Webb, T.: Rates of sediment accumulation in pollen cores from small lakes and mires of eastern North America, Quaternary Res., 30, 284–297, https://doi.org/10.1016/0033-5894(88)90004-X, 1988.
Whitmore, J., Gajewski, K., Sawada, M., Williams, J. W., Shuman, B., Bartlein, P. J., Minckley, T., Viau, A. E., Webb III, T., Shafer, S., Anderson, P., and Brubaker, L.: Modern pollen data from North America and Greenland for multi-scale paleoenvironmental applications, Quaternary Sci. Rev., 24, 1828–1848, https://doi.org/10.1016/j.quascirev.2005.03.005, 2005.
Williams, J. W., Grimm, E. C., Blois, J. L., Charles, D. F., Davis, E. B., Goring, S. J., Graham, R. W., Smith, A. J., Anderson, M., Arroyo-Cabrales, J., Ashworth, A. C., Betancourt, J. L., Bills, B. W., Booth, R. K., Buckland, P. I., Curry, B. B., Giesecke, T., Jackson, S. T., Latorre, C., Nichols, J., Purdum, T., Roth, R. E., Stryker, M., and Takahara, H.: The Neotoma Paleoecology Database, a multiproxy, international, community-curated data resource, Quaternary Res., 89, 156–177, https://doi.org/10.1017/qua.2017.105, 2018.
Wolkovich, E. M., Regetz, J., and O'Connor, M. I.: Advances in global change research require open science by individual researchers, Glob. Change Biol., 18, 2102–2110, https://doi.org/10.1111/j.1365-2486.2012.02693.x, 2012.