the Creative Commons Attribution 4.0 License.
the Creative Commons Attribution 4.0 License.
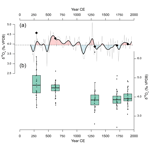
Hydrological change in southern Australia over 1750 years: a bivalve oxygen isotope record from the Coorong Lagoon
Briony Kate Chamberlayne
Jonathan James Tyler
Deborah Haynes
Yuexiao Shao
John Tibby
Bronwyn May Gillanders
Multi-centennial records of past hydroclimate change are essential for understanding the resilience of ecosystems to climatic events in addition to guiding conservation and restoration efforts. Such data are also crucial for examining the long-term controls over regional hydroclimate and the inherent variability in extreme droughts and floods. Here, we present a 1750-year record of hydroclimate variability in the Coorong South Lagoon, South Australia, part of an internationally significant wetland system at the mouth of Australia's largest river, the Murray River. Oxygen isotope ratios were measured from Arthritica helmsi bivalve shells preserved in sediments. The oxygen isotope record shows periods of persistent low and high moisture balance, from ∼500 to 1050 years and from ∼1300 to 1800 years, respectively, which is consistent with other hydroclimate reconstructions from the region. The range of oxygen isotope values in the sedimentary shells does not differ significantly from the estimated range of modern specimens from the present-day lagoon. These data suggest that the restricted and highly evaporated modern-day conditions are not markedly different to the pre-impacted state over the last 1750 years, although the absence of A. helmsi in the contemporary lagoon is likely a response to increased salinity, nutrient loading, and anoxia during the last century. These insights are potentially useful both in guiding management efforts to conserve and restore the Coorong Lagoon and for understanding long-term water resource availability in the region.
- Article
(1942 KB) - Full-text XML
-
Supplement
(396 KB) - BibTeX
- EndNote
Multi-decadal to centennial records of past hydroclimate variability are crucial for understanding long-term climate drivers, for calibrating and validating climate models, for assessing hydroclimate sensitivity to external drivers, and for estimating the probability of multi-decadal climate extremes (Ault et al., 2014; Barr et al., 2014, 2019; Cook et al., 2016; Ho et al., 2015; Kiem et al., 2016; Verdon and Franks, 2007). These data can provide a temporal perspective to setting realistic benchmarks for ecological management not provided by instrumental data (Saunders and Taffs, 2009). Such data are particularly important for the economic and environmental sustainability of the arid and semi-arid regions on the continents of the Southern Hemisphere. In addition, the inherent links between Southern Hemisphere hydroclimate and variability in the southern westerly winds (SWWs) affect oceanographic circulation and carbon cycling between the ocean and atmosphere. Recently, the vegetation and soils in semi-arid landscapes of Australia and Africa have been shown to be major carbon sinks (Haverd et al., 2013; Ma et al., 2016; Poulter et al., 2014), placing further demand upon long-term hydroclimate data in these regions.
To date, decadal-scale records of hydroclimate variability in southern Australia covering the last 2 millennia are relatively rare, mostly due to a scarcity of sufficiently long tree ring chronologies and the geographically narrow distribution of lake and speleothem archives (Dixon et al., 2017; Jansen et al., 2007; Neukom and Gergis, 2011). A recent review of hydroclimate records for the past 2000 years in Australasia identified only eight high-quality records from Australia, i.e. meeting the criteria for the PAGES2k network (Dixon et al., 2019). Alternative reconstructions of Australian hydroclimate variability have utilised distal archives, such as Antarctic ice cores and Chinese speleothems (McGowan et al., 2009; Tozer et al., 2016; Vance et al., 2013); however, major uncertainties exist concerning the stationarity of climate teleconnections when applied beyond the calibration period and geographic range (Dätwyler et al., 2018; Gallant et al., 2013). The relatively low number of high-quality records in Australia limits our understanding of the long-term influence of remote drivers of rainfall, namely the ocean–atmosphere interactions of the El Niño Southern Oscillation (ENSO), the Interdecadal Pacific Oscillation (IPO), the Indian Ocean Dipole (IOD), and the Southern Annular Mode (SAM) indices (Gallant et al., 2012; Risbey et al., 2009). A better understanding of natural, low-frequency climate variability associated with these climate drivers, along with the reconstruction of drought- or flood-dominated epochs, could assist in placing recent events into context and aid in assessing the risks associated with future climate events (e.g. O'Donnell et al., 2021). This is especially relevant for economically important estuaries with populous catchments that are highly sensitive to climatic extremes (e.g. Scanes et al., 2020).
The Coorong Lagoon in southern Australia is one such estuarine ecosystem for which there is limited knowledge of past hydroclimate variability (Kingsford et al., 2011; Reeves et al., 2015). The Coorong, along with the lakes Alexandrina and Albert (the Lower Lakes), form a large wetland at the mouth of the Murray River, which, along with the Darling River, drains the >1 000 000 km2 Murray–Darling Basin (MDB). The MDB is Australia's most important agricultural region, providing around 40 % of Australia's produce, while the region generally is of great economic, cultural, and environmental importance to Australia (MDBA, 2021). The Coorong, along with 15 other wetlands in the MDB, was recognised as a wetland of global significance by inclusion in the Ramsar Convention for Globally Significant Wetlands (Department of Environment and Water, 2021), primarily due to its biodiversity, especially for waterbird species (Paton et al., 2009). Furthermore, The Coorong and Lower Lakes are culturally important sites for the Ngarrindjeri people (Ngarrindjeri Nation and Hemming, 2019). In recent years, the condition of the Coorong Lagoon has deteriorated, with rising salinity and eutrophication and declining biodiversity all linked to declining river discharge due to agricultural and consumptive water extraction (Dittmann et al., 2019; Mosley et al., 2020, 2023; Priestley et al., 2022). Current management efforts are focused on increasing system flushing and reversing eutrophication (Mosley et al., 2020, 2023), including the consideration of proposals to open the South Lagoon of the Coorong to the Indian Ocean. However, as an inverse estuary, the South Lagoon of the Coorong is a unique environmental system, and despite its proximity to the ocean, its natural (pre-impacted) hydrological state is not well understood (Reeves et al., 2015). In this respect, palaeo-environmental studies can provide context regarding the long-term condition of the Coorong, including the range of and resilience to natural hydroclimate variability (Saunders and Taffs, 2009).
Several studies have been undertaken to understand the palaeo-environment of the Coorong Lagoon. Many of these investigated fossil invertebrate and algal assemblages (Dick et al., 2011; Fluin et al., 2007; Krull et al., 2009; Lower et al., 2013; Reeves et al., 2015) and have concluded that the current state of the wetland is unusual in its history. However, many of the dominant species found in the assemblages have broad environmental tolerances and hence give rise to ambiguity in the interpretations. Other studies have included investigations of the carbon, nitrogen, and hydrogen isotope composition of organic matter and of lipid biomarkers as proxies for variations in the source of organic matter and salinity (McKirdy et al., 2010; Tulipani et al., 2014). These methods have found that the North and South lagoons have been separate chemical systems for millennia (McKirdy et al., 2010), although both lagoons have experienced increased salinity levels more recently (McKirdy et al., 2010; Tulipani et al., 2014). Modern sediments have been well dated using lead-210 (210Pb), fallout product caesium-137 (137Cs), plutonium (), and the first arrival of exotic Pinus radiata pollen (Fluin et al., 2007; Krull et al., 2009). However, several previous studies have encountered challenges related to radiocarbon dating uncertainty, largely due to a relatively small number of dates, which makes it difficult to place long-term changes into a regional context (e.g McKirdy et al., 2010).
Here, we use the geochemistry of the bivalve Arthritica helmsi (which are abundantly preserved in the sediments of The Coorong's South Lagoon) to add to the growing body of research investigating the palaeoenvironmental history of this significant wetland. A modern study of oxygen isotope fractionation in A. helmsi demonstrated the sensitivity of bivalve geochemistry from this species to environmental change (Chamberlayne et al., 2021). In this study, we analyse oxygen isotope ratios in shells preserved in a sediment core from the South Lagoon to (i) assess the drivers of hydrological change in the context of regional climate variability and (ii) understand hydrological change in the South Lagoon through time.
2.1 Site description
The Coorong Lagoon and the Goolwa Channel comprise the Murray River estuary (Fig. 1). The Coorong is separated from the Indian Ocean by Holocene dunes (Murray-Wallace, 2018) through which the Murray mouth provides the drainage for the Murray–Darling river system. The Coorong Lagoon is a single entity; however, the constriction at Parnka Point effectively divides The Coorong into two separate basins. While the basins do not have specific names, from a hydrological, geochemical, and ecological perspective they are quite distinct from each other, and recent scientific and management efforts have had a specific focus on the southern basin. As a consequence, as is the case with several other studies on The Coorong, this study adopts the informal nomenclature of the North Lagoon and South Lagoon (Fig. 1). The Coorong is characterised by a north–south salinity gradient of fresh to hypersaline waters (Gillanders and Munro, 2012; Shao et al., 2021). A series of barrages constructed in the 1930s to restrict marine incursions and retain freshwater in Lake Alexandrina now regulate freshwater discharges from Lake Alexandrina into The Coorong and Murray mouth (Maheshwari et al., 1995). Currently, water in the North Lagoon is recharged by outflows from Lake Alexandrina and inflows from the Indian Ocean, while the South Lagoon is primarily recharged by direct rainfall, groundwater, and continental run-off from wetlands in the southeast of the state (Chamberlayne et al., 2021; Shao et al., 2018)
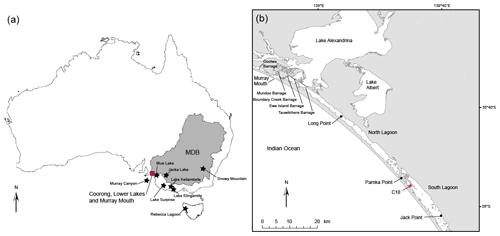
Figure 1(a) Map of Australia showing the location of the Murray–Darling basin (MDB); Coorong, Lower Lakes, and Murray mouth (red box); and proxy records included in the synthesis of southeastern Australian hydroclimate records by Dixon et al. (2019; black stars). (b) Map of the Coorong, Lower Lakes, and Murray mouth with the location for core C18 highlighted with a red star.
2.2 Sample collection and preparation
Core C18 was extracted from the South Lagoon (Fig. 1) in 2005 using a percussion corer with a 50 mm internal diameter PVC pipe (Chambers and Cameron, 2001) and stored at 4 ∘C in refrigerated facilities at The University of Adelaide. The 130 cm long core was sliced longitudinally, and one half was sampled for diatom and preliminary radiocarbon analyses (Krull et al., 2009). The remaining half of the core was then cut into 1 cm intervals and freeze dried. Arthritica helmsi shells were picked for analysis from the dried sediment and cleaned using a brush and distilled water before being oven dried at 40 ∘C for 12 h.
2.3 Chronology
The chronology for the C18 record was established via a combination of accelerator mass spectrometry (AMS 14C) radiocarbon dating and the first appearance of Pinus radiata pollen. Seventeen 14C dates were obtained from bivalve shells sampled throughout the core (Table S1 in the Supplement). The exotic Pinus radiata pollen was first introduced to Australia by Europeans, and its detection in sediments can be used as a chronostratigraphic marker for post-colonial expansion of European settlement and agriculture in the region (Tibby et al., 2003). 210Pb and 137Cs dating of adjacent cores in the Coorong Lagoon indicates that the first detection of Pinus is representative of ∼1955 CE (Krull et al., 2009). In core C18, Pinus first appears at 9 cm depth (Krull et al., 2009). Further details of the collection, preparation, and identification of Pinus can be found in Krull et al. (2009).
To assess the presence of old carbon from groundwater which has affected radiocarbon dating in other southeast Australian lakes (Barr et al., 2014; Gouramanis et al., 2010; Wilkins et al., 2012), the 14C age for the depth corresponding to Pinus arrival was compared to a 210Pb-inferred 14C age (14C210Pb), with uncertainty, following Barr et al. (2014). This was achieved by projecting a probability density curve based on the mean 210Pb age and standard deviations derived from Krull et al. (2009) against the Southern Hemisphere 14C calibration curve (Hogg et al., 2020). The mean 14C age within the error bracket was used to estimate 14C210Pb, weighted by its probability. The reservoir age was then estimated by subtracting 14C210Pb from measured 14C, and the resulting uncertainty was calculated using the following equation:
where σres and σatm are the errors associated with the 14C age of the reservoir-derived and atmosphere-derived objects, respectively (Soulet, 2015). This old-carbon effect was then subtracted from all 14C measurements from core C18 prior to calibration with the Southern Hemisphere calibration curve (Hogg et al., 2020) using the program Bacon (Blaauw and Christen, 2011) in RStudio (R Core Team, 2021).
2.4 Stable isotope analysis
In order to obtain a representative sample of the past environment, multiple individuals were combined for each analysis. Five pre-cleaned shells, collected in the 1 cm sample intervals throughout core C18, were crushed to a fine powder using an agate mortar and pestle. A sub-sample of approximately 100 µg from this carbonate powder was used for stable isotope analysis. Five valves was found to be representative of trace-element variability in a larger sample (Chamberlayne, 2015) and allowed the sample size to remain consistent throughout the core. In addition, to assess the degree of intra-sample variability, multiple individual shells of A. helmsi were also analysed from samples taken at five depths, namely 11 cm (n=30 shells), 26 cm (n=29), 57 cm (n=27), 92 cm (n=30), and 119 cm (n=27). Data from these intervals were integrated into the final record by calculating the weighted mean of the bulk sample and replicate samples from particular depths. Samples were flushed with helium and then acidified with 105 % phosphoric acid at 70 ∘C using a Nu Instruments GasPrep device before isotope analysis of the resulting CO2 with a Nu Instruments Horizon isotope ratio mass spectrometer in continuous-flow mode. Two aragonite laboratory standards, ANU-P3 () and UAC-1 (), accounted for 27 of every 100 analyses; the precision was better than 0.12 ‰ for δ18O based on replicate analyses of these standards. Oxygen isotope data are reported as δ18Os relative to the Vienna PeeDee Belemnite (VPDB) standard using the standard delta (δ) notation in parts per thousand (‰):
where R is the isotope ratio ().
2.5 Comparison of oxygen isotope ratios in core C18 and modern Arthritica helmsi
The oxygen isotope ratios measured on A. helmsi shells in core C18 were compared to both measured and predicted oxygen isotope ratios of shell carbonate in the modern North and South lagoons. Oxygen isotope ratios for live collected individuals of A. helmsi from the North Lagoon were sourced from Chamberlayne et al. (2021), who measured δ18O on shells from five locations throughout the North Lagoon from 2016 to 2018. As there are currently no living populations of A. helmsi in the South Lagoon (Dittmann et al., 2019), the oxygen isotope ratio of shell carbonate was predicted using measured temperature and oxygen isotope ratios in water reported by Chamberlayne et al. (2021). Using this approach, oxygen isotope ratios of shells (δ18Os) were predicted for hypothetical populations at Parnka Point and Jack Point using the following temperature-dependent oxygen isotope fractionation equation developed for A. helmsi by Chamberlayne et al. (2021):
To determine whether the oxygen isotope compositions varied between sites, we first tested if the data sets were normally distributed using a Shapiro–Wilk test. As the data from core C18 were not normally distributed (w=0.977, p=0.037), Wilcoxon μ tests were used to compare groups.
3.1 Chronology
The age offset due to old carbon in the South Lagoon was calculated by comparing the 14C age of bivalves from the depth corresponding with the first occurrence of Pinus pollen in core C18. In other South Lagoon cores, alpha and gamma spectrometry-based 210Pb analyses indicate that the first occurrence of Pinus in the sediments dates to 1955 ± 5 CE (−5 ± 5 yr BP; Fluin et al., 2007; Krull et al., 2009). This 210Pb-derived age was estimated to equate to 169 ± 10 14C yr BP, which was subtracted from the equivalent measured radiocarbon age (985 ± 30 14C yr BP) to produce an age offset of 816 ± 32 14C yr BP. The results of AMS radiocarbon dating are summarised in Table S1 in the Supplement. The age–depth model produced following the application of this offset to measured radiocarbon ages is shown in Fig. 2. Three samples were found to be more than 500 years older than the above or below samples and were therefore deemed to be outliers and excluded from the age–depth model (Table S1 in the Supplement). The weighted mean basal age for core C18 is 1755 cal yr BP (195 CE).
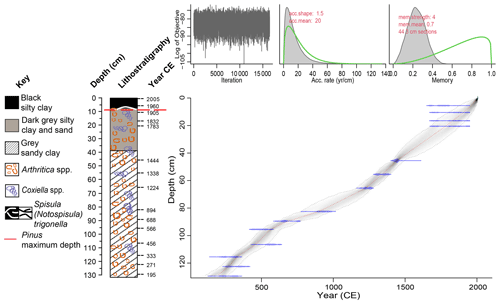
Figure 2Age–depth model and lithostratigraphy for core C18. Blue markers show the probability distributions for each dated sample. Dotted grey lines indicate modelled 95 % confidence intervals, where darker shading indicates more likely ages. The modelled weighted mean age is shown by the dotted red line. Upper panels indicate the iterations performed, accumulation rate, and memory used to construct the model.
3.2 Oxygen isotope ratios
The δ18Os values measured from each centimetre of core C18 (n=123) ranged from 2.86 ‰ at 104 cm to 5.20 ‰ at 61 cm, with a mean of 3.95 ‰ (Fig. 3a). δ18Os values were above average for the time periods ∼269–439, 529–1065, 1218–1321, and 1789–2005 CE and were below average for the time periods ∼195–275, 439–529, 1065–1218, and 1299–1789 CE (Fig. 3a). The oxygen isotope compositions of multiple individual A. helmsi shells from five intervals showed high variability (Fig. 3b). The median δ18Os ranged from 4.61 ‰ ± 0.69 ‰ at 119 cm (300 CE) to 3.84 ‰ ± 0.64 ‰ at 57 cm (1321 CE; Fig. 3b). Excluding outliers, the range of values measured was highest at 119 cm (2.66 ‰; 300 CE) and lowest at 92 cm (0.99 ‰; 631 CE).
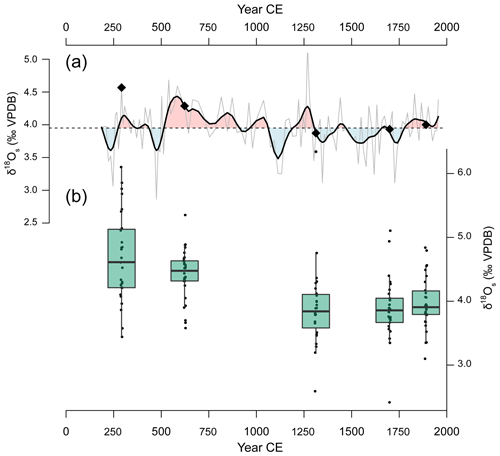
Figure 3Arthritica helmsi oxygen isotope ratios from core C18 plotted against time. In (a) the grey line represents a bulk measurement (n=5) from each centimetre of C18. The dashed line indicates the mean of all values, and the bold line is a smooth spline (spar=0.4). Blue shading indicates periods of below-mean δ18Os, while the red shading indicates periods of above-mean δ18Os. Black diamonds indicate where data from multiple individuals (n≥27) are incorporated into the bulk curve. (b) Boxplots of oxygen isotope ratios of A. helmsi shells. Data are obtained from analyses of multiple individual shells from depths corresponding to ages of 300 (n=30), 631 (n=29), 1321 (n=27), 1708 (n=30), and 1899 yr CE (n=27).
A Wilcoxon test indicated that the distribution of oxygen isotope values measured on aggregate samples of shells of A. helmsi extracted from core C18 was not significantly different from the δ18Os values predicted by the modern-water δ18O and temperature at Jack Point (p=0.15) and Parnka Point (p=0.18) in the South Lagoon (Fig. 5a). The range of δ18Os values measured from core C18 was, however, narrower than that predicted for modern carbonates. When the range of measured δ18Os values from the North Lagoon was compared to the values from core C18, the two populations were significantly different (p<0.001; Fig. 5b). To assess sampling bias, oxygen isotope values for individual A. helmsi shells were compared to the δ18Os values predicted for the modern South Lagoon and the range of measured δ18Os values from the modern North Lagoon. In agreement with the aggregate δ18Os samples, individual δ18Os values are distinctly different from the modern North Lagoon, yet they are consistent within the range of estimated δ18Os values for the South Lagoon (Fig. S1 in the Supplement).
Proxy records of hydroclimate are required to understand variability extending past Australia's short instrumental climate record. This study presents variation in the oxygen isotope ratios of shells of A. helmsi spanning the last ∼1750 years in the South Lagoon of the Coorong. This record is constrained by 17 radiocarbon dates, allowing accurate identification of the timing of hydrological change, albeit this is subject to the need to correct radiocarbon dates for a substantial old-carbon effect. Here, we interpret the record in the context of previous studies of Coorong palaeohydrology in addition to regional hydroclimate reconstructions. Furthermore, the possible drivers of hydrological change in the Coorong's South Lagoon are considered.
4.1 Interpreting δ18Os in South Lagoon sediments
The processes that control the fractionation of oxygen isotopes in the carbonate of A. helmsi were examined in a previous study of modern populations in the Coorong Lagoon (Chamberlayne et al., 2021). A temperature-dependent fractionation equation was developed in that study, which determined that an increase in δ18Os by 1 ‰ corresponds to a decrease in temperature by ∼4.43 ∘C (Chamberlayne et al., 2021; Eq. 3). Alternatively, a change in water temperature by 1 ∘C would be expressed as a ∼0.23 ‰ change in the oxygen isotope value of A. helmsi. As this study sampled multiple shells per sample to reduce seasonal and inter-annual bias in the signal captured by the oxygen isotope composition of shells, we interpret this record to be more heavily influenced by the isotopic composition of water rather than temperature. This interpretation is based, in part, on the relatively low variance in regional temperature reconstructions for the last 2000 years (Kaufman et al., 2020) whilst acknowledging that mainland Australia remains very poorly represented by global temperature reconstructions for recent millennia. The oxygen isotopic composition of modern Coorong waters showed a positive linear relationship with salinity, demonstrating an overriding dominance of evaporation over isotopic variability within the lagoon, although the slope and strength of this relationship varied spatially (Chamberlayne et al., 2021). As such, the oxygen isotope record developed in this study is primarily interpreted as reflecting the inflow : evaporation (I : E) water balance of the South Lagoon.
4.2 Palaeohydrology of the Coorong Lagoon and southeastern Australia
The oxygen isotope record from A. helmsi implies a relatively stable hydrological state over the last ∼1750 years, superimposed by periods of both enhanced and reduced I : E (Fig. 3a). In particular, between 500–1050 CE, higher δ18Os is interpreted to reflect a period of prolonged, relatively low I : E (Fig. 3a). A relatively brief period of low δ18Os occurred between ∼1050–1200 CE, prior to another period of high δ18Os between 1200–1300 CE (Fig. 3a). This overall period of relatively dry climate in this record largely coincides with records from western Victoria, approximately 300–500 km southeast of The Coorong (Barr et al., 2014; Dixon et al., 2019; Tyler et al., 2015; Wilkins et al., 2013). Two diatom-inferred conductivity (salinity) records from Lake Surprise and Lake Elingamite indicate both drier and more variable climate during this period (Fig. 4; Barr et al., 2014). Diatom-based ecological indicators, such as a greater proportion of shallow-water diatom taxa suggestive of lower lake levels at these and two other lakes (Tower Hill and Lake Purrumbete), also indicate dryer climates prior to 1300 AD (Barr et al., 2014; Tyler et al., 2015). Warmer temperatures and lower lake levels were also inferred from the increased frequency of aragonite laminae in the sediments of Lake Keilambete and Lake Gnotuk in western Victoria (Fig. 4; Wilkins et al., 2013). Off the coast of South Australia, proximal to the River Murray mouth, alkenone-derived sea surface temperatures (SSTs) in the Great Australian Bight suggest a warm-water anomaly from 650 to 950 CE despite an overall cooling trend in the late Holocene (Perner et al., 2018). Chronological uncertainties preclude the precise alignment of these records; however, the broad agreement suggests that a period of relatively dry climate occurred between ∼500 to 1200 CE across southeastern Australia (Fig. 4).
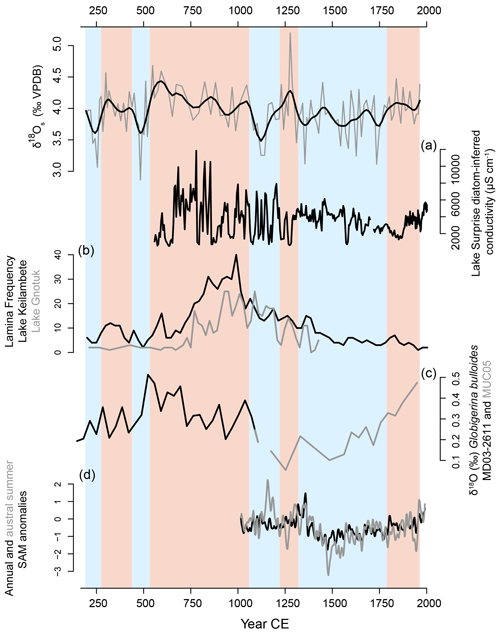
Figure 4δ18Os from core C18 A. helmsi shells compared against (a) diatom-inferred lake conductivity from Lake Surprise, southeast Australia (SEA)* (Barr et al., 2014); (b) lamina frequency in Lake Keilambete and Lake Gnotuk, SEA* (Wilkins et al., 2013); (c) Globigerina bulloides δ18O from Murray Canyon cores MD03-2611 and MUC05* (Moros et al., 2009; Barrows et al., 2020); and (d) austral summer and annual mean SAM index reconstructions (Dätwyler et al., 2017, 2018). Red and blue shading represents periods of higher- and lower-than-average A. helmsi δ18Os, respectively. Records with a * were included in the synthesis by Dixon et al. (2019).
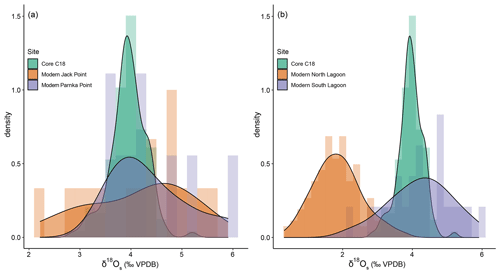
Figure 5Density plots with histograms of A. helmsi δ18Os, comparing values from core C18 with (a) modern locations in the South Lagoon and (b) the North and South lagoons. Modern δ18Os values for the North Lagoon are sourced from Chamberlayne et al. (2021). δ18Os values for the modern South Lagoon sites Jack Point and Parnka Point are calculated from temperature and δ18Ow values from Chamberlayne et al. (2021) using the oxygen isotope fractionation equation developed in that study.
Excursions in compound-specific hydrogen isotope ratios of n alkanes in South Lagoon sediments, alongside diatom-inferred reductions in salinity, suggested two major freshening events at ∼1100 and ∼1410 CE (McKirdy et al., 2010). The timing of these events, which were attributed to increased surface runoff and groundwater inflow (McKirdy et al., 2010), is broadly coincident with negative excursions in δ18Os derived in this study, although additional freshening events are inferred at around 250, 500, 1500, and 1750 CE (Fig. 3a), likely artefacts of higher-resolution sampling in the C18 record. Beyond this, the period between ∼1300–1800 CE appears to have experienced higher I : E compared to the preceding millennium, with a possible shift towards drier conditions after ∼1800 CE (Fig. 3a). The timing of this wetter phase corresponds with the Little Ice Age, a period of significant global climate change, including cooling over the Northern Hemisphere continents (Gebbie and Huybers, 2019). Several sites in southeastern Australia have also recorded climates with high effective moisture during this period. Lakes in western Victoria showed reduced variability with high inferred precipitation : evaporation prior to 1840 CE (Jones et al., 2001; Tibby et al., 2018; Tyler et al., 2015). At Lake Surprise, the period is marked by a prolonged phase of low diatom-inferred conductivity (Fig. 4; Barr et al., 2014), and higher lake levels are inferred for Lake Keilambete after 1500 CE (Wilkins et al., 2013). Furthermore, oxygen isotope ratios in the planktonic foraminifer species Globigerina bulloides from sediments in the Murray Canyons offshore South Australia indicate that the Little Ice Age was possibly a prominent cold phase (Fig. 4; Moros et al., 2009).
A recent study examining coherent variability in proxy records of southeastern Australian hydroclimate during the last 1200 years has identified two vectors interpreted to reflect hydroclimate changes (Dixon et al., 2019). The statistical analysis included eight proxy records which met stringent criteria for inclusion in the PAGES Aus2k database (Dixon et al., 2019). The analysis suggests an increase in effective moisture between 900 and 1750 CE and generally wetter conditions between 1400 and 1750 CE (Dixon et al., 2019). The agreement of the results of this regional synthesis with the interpretation of the Coorong oxygen isotope record suggests that hydroclimate variability in the South Lagoon may have been consistent with broader, regional-scale variability and climate forcing.
Comparison of this record with others from the region is enabled by the construction of the age–depth model constrained by 17 radiocarbon dates. However, three dates were excluded from the model due to being out of sequence with those above and below. One possibility is that the carbon offset applied in the model may not be constant through time due to varying inputs of young carbon. Other possibilities include the mixing of top sediments by storm activity or that older shells were washed into the lagoon from older shorelines. Further radiocarbon dating of adjacent cores would greatly improve the confidence in the timing of the change in this record in addition to addressing these uncertainties.
4.3 Drivers of regional hydroclimate in the Coorong and southeastern Australia
Rainfall in southeastern Australia is driven by multiple large-scale ocean–atmosphere interactions including ENSO, IOD, and SAM, which can affect autumn, summer, and spring rainfall on different timescales (Gallant et al., 2012; Holgate et al., 2022; Risbey et al., 2009). As such, the moisture balance in the Coorong is controlled by multiple and co-varying drivers and so is difficult to attribute to a single climate process. Of those drivers, the effect of ENSO on hydroclimate in southeastern Australia during the last 2 millennia presents a conundrum, since the transition from the Medieval Climate Anomaly to the Little Ice Age in the Northern Hemisphere (e.g. Wanamaker et al., 2012) is often inferred to have coincided with a shift from a more La-Niña-like climate towards El Niño dominance (Mann et al., 2009; Perner et al., 2018). In southeastern Australia, however, this shift is towards wetter climate during the Little Ice Age, in agreement with records from the western Pacific (Emile-Geay et al., 2013), thus contrasting with the expected relationship between El-Niño-like climates and drought (Wang and Hendon, 2007).
In a recent review of palaeoclimate-informed IOD trends, variability, and interactions, Abram et al. (2020a) proposed that changes in mean hydroclimate from prolonged periods of drier- or wetter-than-average conditions could be explained by changes in the strength of interannual IOD and ENSO variability rather than changes in the mean state of either forcing. Furthermore, studies of recent droughts in Australia have concluded that the lack of La Niña and negative IOD events, and hence reduced interannual variability, is responsible for prolonged drought conditions (King et al., 2020; Ummenhofer et al., 2009). The shift from dry to wet conditions observed in the South Lagoon of the Coorong and other southeastern Australian records around 1300 CE broadly coincides with a mid-millennium increase in the variability of IOD and ENSO in the eastern equatorial Indian Ocean (Abram et al., 2020b) and amplified ENSO variability in the tropical Pacific Ocean (Rustic et al., 2015).
The southern westerly winds (SWWs), which are largely described by the SAM index, also impact hydroclimate in southeastern Australia (Marshall, 2003; Risbey et al., 2009), particularly the generation of frontal rainfall during the winter, albeit over shorter timescales than ENSO and the IOD (King et al., 2020). Positive phases of SAM, in association with a southerly shift of the SWWs, have been related to reduced rainfall in southeastern Australia in several studies (Cai and Cowan, 2006; Hendon et al., 2007; Risbey et al., 2009). Proxy-data-based reconstructions of SAM show a negative trend from ∼1300 CE (Abram et al., 2014; Dätwyler et al., 2018), coinciding with the shift from dry to wet hydroclimate in the South Lagoon (Fig. 4). However, this observation contrasts with an inferred period of dry conditions recorded by pollen and charcoal data from lake sediments in Tasmania, which are interpreted to reflect a positive SAM anomaly between 950–1450 CE (Fletcher et al., 2018). In contrast, this period is inferred to have been a period of both wet (∼1000–1200 CE) and dry (1200–1300 CE) conditions in the South Lagoon (Fig. 4). It is possible that the timing of positive SAM events could cause this variation. A high index polarity of SAM during winter has been found to result in decreased daily rainfall over southeast and southwest Australia, whereas during summer, it is associated with decreased rainfall in western Tasmania but increased rainfall in the southeastern region of mainland Australia (Hendon et al., 2007). As a consequence, differences in the seasonal effects of SAM on the climate of mainland Australia and western Tasmania may explain the apparent differences in timing of wet and dry periods between the two regions. Reconstructions of SAM during the last millennium suggest increased austral summer anomalies at ∼1150 CE, which were not evident in annual SAM anomalies, which instead peak at ∼1300 CE (Fig. 4; Dätwyler et al., 2018). The temporal similarities in summer and annual positive SAM events and the wet and dry phases in the C18 record suggest that the season of SAM anomalies may therefore be an influential driver of multi-decadal- to centennial-scale hydroclimate variability in the South Lagoon and possibly over regional southeastern Australia.
4.4 The pre-impacted hydrology of the South Lagoon: implications for restoration targets
The ecological demise of the South Lagoon has led to calls for drastic action to reduce salinity and nutrient loading in the lagoon (Project Coorong, 2021). The solutions considered include improvements to the connectivity between the North and South lagoons and maintaining the Murray mouth to provide greater seawater exchange with the Coorong (Department for Environment and Water, 2019). Such proposed actions highlight an urgent need to understand the pre-impacted hydrological state of the Coorong, which in turn led to the development of this unique and iconic ecosystem.
The ubiquitous presence of A. helmsi in core C18, and in South Lagoon sediments in general, suggests that the lagoon provided favourable conditions for this species prior to the 1950s (salinity <55; Wells and Threlfall, 1982). This finding is in agreement with the evidence that the aquatic plant Ruppia megacarpa, which has a salinity tolerance of 2 to 50, was the dominant species of macrophyte in the South Lagoon for the 3000 years prior to European impact (Dick et al., 2011). In addition, analyses of foraminifera (Dick et al., 2011) and diatom assemblages (McKirdy et al., 2010) suggest that water salinity in the South Lagoon was relatively stable for at least 3000 years prior to the 1950s. Estimates of the pre-European salinity of the South Lagoon from microfossil and geochemical evidence range from estuarine (∼5–30; Dick et al., 2011) to slightly above that of seawater (>35; Reeves et al., 2015) based on microfauna and microflora fossil assemblages. The range of oxygen isotope values in individual A. helmsi shown in Fig. 3 also suggests a high range of water salinity in the past. Previous work has highlighted the effect of 20th century hydrological alteration, land clearance, and water abstraction in the MDB upon salinity and ecological conditions in the Coorong (Fluin et al., 2007; Krull et al., 2009). It is during this period that A. helmsi disappear from core C18 (∼1965) and that a black, clay-rich mono-sulfidic ooze appears – the result of anoxia and highly reducing conditions (Fig. 2; Krull et al., 2009). The combination of high salinity and anoxia in the South Lagoon made conditions unsuitable for A. helmsi (Dittmann et al., 2019), and this, along with other environmental indicators, suggests a departure from the conditions that prevailed prior to this time (Kingsford et al., 2011).
Despite the loss of A. helmsi shells from the sediment core, it appears that the I : E in the Coorong has remained relatively stable for the last ∼1750 years. The oxygen isotope values measured from sedimentary shells do not significantly differ from values estimated for modern populations calculated from measured water temperature and oxygen isotope composition in the South Lagoon (Fig. 5a). While the range of the predicted modern values is broader for both Parnka Point and Jack Point than those observed in core C18, the overlap in values suggests that the South Lagoon has been an isolated, highly evaporative system for the last ∼1750 years. In addition to falling within the range of predicted South Lagoon populations, the δ18Os values from core C18 are statistically different from the δ18Os values measured from modern populations in the North Lagoon (Fig. 5b), which has been subject to a greater influence of seawater and river water in the past and in the modern system. The waters in which the shells from the North Lagoon were collected range in salinity from 0 to 46 and in δ18O from −2.81 ‰ to 3.87 ‰ (Chamberlayne et al., 2021). The higher δ18Os values from core C18 compared to those from the modern North Lagoon suggest that the South Lagoon has, for the past 1750 years, been a more isolated and evaporated system compared to the contemporary North Lagoon. Evidence of the North and South lagoons being separate geochemical systems through time has also been inferred through the analysis of carbon and nitrogen isotope ratios from sediment cores from the two lagoons (McKirdy et al., 2010). Furthermore, microfauna assemblages point to the South Lagoon being more saline than the North Lagoon for at least the last millennium (Reeves et al., 2015).
While it is evident that the South Lagoon has undergone an increase in salinity over the last century, a consequence of both climate change and direct human impact, it appears that the hydrological balance of the lagoon has been relatively constant and that additional stressors – namely eutrophication and anoxia – may have played a major role in the recent loss of biodiversity in the system. A relatively constant hydrological balance in concert with an increase in salinity also implies that the salinity of inflowing water to the South Lagoon must have changed. Such changes may have arisen due to an increase in the flux of saline groundwater, either directly into the South Lagoon along its margins (Haese et al., 2009) or into the Salt Creek tributary, which flows into the South Lagoon. While the flow of River Murray water into Lake Alexandrina and the Coorong may have been relatively unchanged prior to human impacts, the salinity of inflowing waters has increased since that time (Hart et al., 2020), which, when subjected to intense evaporation, may have enhanced the hypersalinity of the modern South Lagoon. This study did not analyse δ18O in A. helmsi shells in sediments of the North Lagoon, though this would allow a comparison of the hydrology of each lagoon through time and would therefore be an interesting avenue for future research.
This study examined hydrological change over the last 1750 years in the Coorong South Lagoon, South Australia, through the analysis of oxygen isotopes of bivalve shells preserved in lagoonal sediments. Variability in δ18Os was interpreted as an inverse function of the balance of inflow over evaporation in the South Lagoon catchment. The overall range of δ18Os values measured from shells of A. helmsi suggests that the modern-day hydrological balance of the South Lagoon is not markedly different to that inferred for the last 1750 years, indicating that the southern basin was always a highly evaporated water body. Furthermore, the sedimentary δ18Os values differ significantly from those in the modern-day North Lagoon, reinforcing previous suggestions that the two lagoons have evolved as separate systems and that the modern North Lagoon is not an analogue for the pre-impacted South Lagoon. Studies employing similar chronological and geochemical techniques on other sediment cores from the Coorong lagoons would greatly strengthen the interpretation of this record.
Two extended periods of low and high inflow : evaporation were inferred from ∼500 to 1050 and ∼1300 to 1800 CE, respectively, consistent with other hydroclimate records in the region, in turn suggesting a common driver for multi-decadal- to centennial-scale hydroclimate variability in southeastern Australia. Comparison with reconstructed indices for major ocean–atmosphere interactions suggests the importance of the timing of SAM anomalies to hydroclimate in the Coorong, where negative SAM phases corresponded to wet periods in the Coorong, in agreement with previous studies. Changes in the variability of IOD and ENSO may have contributed to changes in the mean hydrological state. Consequently, this study contributes to both our understanding of the mechanisms of natural hydrological variability in southeastern Australia and our knowledge of the pre-impacted state of the South Lagoon in particular and the ecologically significant Coorong Lagoon system generally. Such data can assist in understanding the resilience of hydrological systems to long-term climate change trajectories.
The original data used in this research study are archived in the Mendeley Data Repository (https://doi.org/10.17632/4vktdpckhv.1, Chamberlayne, 2022).
The supplement related to this article is available online at: https://doi.org/10.5194/cp-19-1383-2023-supplement.
BKC, JJT, and BMG designed this study, and BKC carried it out. The sediment core used in this study was collected by JT and DH. The funding for coring and radiocarbon dating at Waikato University was obtained by DH; the funding for radiocarbon dating at ANSTO was obtained by BKC and YS. BKC prepared the paper with contributions from all the co-authors.
The contact author has declared that none of the authors has any competing interests.
Publisher's note: Copernicus Publications remains neutral with regard to jurisdictional claims in published maps and institutional affiliations.
We acknowledge the Coorong, Lower Lakes, and Murray Mouth region and surrounding areas as Ngarrindjeri Country and thank the Ngarrindjeri Regional Authority for permission to undertake the coring. We would like to thank Mark Rollog for his assistance with the stable isotope analyses. This project was undertaken while Briony Kate Chamberlayne and Yuexiao Shao were recipients of an Australian Postgraduate Award and Australian Graduate Research Scholarship, respectively.
This research has been supported by the Australian Institute of Nuclear Science and Engineering (Honours Scholarship and Australian National Collaborative Research Infrastructure Strategy grant) and the Australian Research Council (project no. LP0667819).
This paper was edited by Nerilie Abram and reviewed by Patrick De Deckker and two anonymous referees.
Abram, N. J., Mulvaney, R., Vimeux, F., Phipps, S. J., Turner, J., and England, M. H.: Evolution of the Southern Annular Mode during the past millennium, Nat. Clim. Change, 4, 564–569, https://doi.org/10.1038/nclimate2235, 2014.
Abram, N. J., Hargreaves, J. A., Wright, N. M., Thirumalai, K., Ummenhofer, C. C., and England, M. H.: Palaeoclimate perspectives on the Indian Ocean Dipole, Quaternary Sci. Rev., 237, 106302, https://doi.org/10.1016/j.quascirev.2020.106302, 2020a.
Abram, N. J., Wright, N. M., Ellis, B., Dixon, B. C., Wurtzel, J. B., England, M. H., Ummenhofer, C. C., Philibosian, B., Cahyarini, S. Y., Yu, T. L., Shen, C. C., Cheng, H., Edwards, R. L., and Heslop, D.: Coupling of Indo-Pacific climate variability over the last millennium, Nature, 579, 385–392, https://doi.org/10.1038/s41586-020-2084-4, 2020b.
Ault, T. R., Cole, J. E., Overpeck, J. T., Pederson, G. T., and Meko, D. M.: Assessing the risk of persistent drought using climate model simulations and paleoclimate data, J. Climate, 27, 7529–7549, https://doi.org/10.1175/JCLI-D-12-00282.1, 2014.
Barr, C., Tibby, J., Gell, P., Tyler, J., Zawadzki, A., and Jacobsen, G. E.: Climate variability in south-eastern Australia over the last 1500 years inferred from the high-resolution diatom records of two crater lakes, Quaternary Sci. Rev., 95, 115–131, https://doi.org/10.1016/j.quascirev.2014.05.001, 2014.
Barr, C., Tibby, J., Leng, M. J., Tyler, J. J., Henderson, A. C. G., Overpeck, J. T., Simpson, G. L., Cole, J. E., Phipps, S. J., Marshall, J. C., McGregor, G. B., Hua, Q., and McRobie, F. H.: Holocene El Niño–Southern Oscillation variability reflected in subtropical Australian precipitation, Sci. Rep.-UK, 9, 1–9, https://doi.org/10.1038/s41598-019-38626-3, 2019.
Barrows, T. T., Perner, K., and De Deckker, P.: Sea surface temperature from sediment cores MD03-2611G and MUC-3 reconstructed by modern analogue technique, PANGAEA [data set], https://doi.org/10.1594/PANGAEA.911822, 2020.
Blaauw, M. and Christen, J. A.: Flexible Paleoclimate Age-Depth Models Using an Autoregressive Gamma Process, Bayesian Anal., 6, 457–474, https://doi.org/10.1214/11-BA618, 2011.
Cai, W. and Cowan, T.: SAM and regional rainfall in IPCC AR4 models: Can anthropogenic forcing account for southwest Western Australian winter rainfall reduction?, Geophys. Res. Lett., 33, 1–5, https://doi.org/10.1029/2006GL028037, 2006.
Chamberlayne, B.: Late Holocene seasonal and multicentennial hydroclimate variability in the Coorong Lagoon, South Australia: Evidence from stable isotopes and trace element profiles of bivalve molluscs, Honours thesis, University of Adelaide, https://hdl.handle.net/2440/117977, 2015.
Chamberlayne, B. K.: 1750 years of hydrological change in southern Australia: a bivalve oxygen isotope record from The Coorong Lagoon, Mendeley Data [data set], https://doi.org/10.17632/4vktdpckhv.1, 2022.
Chamberlayne, B. K., Tyler, J. J., and Gillanders, B. M.: Controls Over Oxygen Isotope Fractionation in the Waters and Bivalves (Arthritica helmsi) of an Estuarine Lagoon System, Geochem. Geophy. Geosy., 22, 1–18, https://doi.org/10.1029/2021gc009769, 2021.
Chambers, J. W. and Cameron, N. G.: A rod-less piston corer for lake sediments: An improved, rope-operated percussion corer, J. Paleolimnol., 25, 117–122, https://doi.org/10.1023/A:1008181406301, 2001.
Cook, B. I., Palmer, J. G., Cook, E. R., Turney, C. S. M., Allen, K., Fenwick, P., O'Donnell, A., Lough, J. M., Grierson, P. F., Ho, M., and Baker, P. J.: The paleoclimate context and future trajectory of extreme summer hydroclimate in eastern Australia, J. Geophys. Res., 121, 12820–12838, https://doi.org/10.1002/2016JD024892, 2016.
Dätwyler, C., Neukom, R., Abram, N. J., Gallant, A. J. E., Grosjean, M., Jacques-Coper, M., Karoly, D. J., and Villalba, R.: Teleconnection stationarity, variability and trends of the Southern Annular Mode (SAM) during the last millennium, NOAA [data set], https://doi.org/10.1007/s00382-017-4015-0, 2017.
Dätwyler, C., Neukom, R., Abram, N. J., Gallant, A. J. E., Grosjean, M., Jacques-Coper, M., Karoly, D. J., and Villalba, R.: Teleconnection stationarity, variability and trends of the Southern Annular Mode (SAM) during the last millennium, Clim. Dynam., 51, 2321–2339, https://doi.org/10.1007/s00382-017-4015-0, 2018.
Department of Environment and Water: Coorong and Lakes Alexandrina and Albert Wetland Ramsar Site: https://www.environment.sa.gov.au/topics/water/water-and-the-environment/wetlands/Coorong_and_Lakes_Alexandrina_and_Albert_wetland_Ramsar_site, last access 17 June 2021.
Dick, J., Haynes, D., Tibby, J., Garcia, A., and Gell, P.: A history of aquatic plants in the Coorong, a Ramsar-listed coastal wetland, South Australia, J. Paleolimnol., 46, 623–635, https://doi.org/10.1007/s10933-011-9510-4, 2011.
Dittmann, S., Gordillo, O. L., and Baring, R.: Benthic Macroinvertebrate survey 2018–2019 report Coorong, Lower Lakes and Murray Mouth Icon Site, Adelaide, SA, https://www.mdba.gov.au/sites/default/files/pubs/coorong-lower-lakes-murray-mouth-benthic-macroinvertebrate-survey-2018-2019.pdf (last access: 10 June 2021), 2019.
Dixon, B., Tyler, J., Henley, B. J., and Drysdale, R.: Regional patterns of hydroclimate variability in southeastern Australia over the past 1200 years, Earth Sp. Sci. Open Arch., https://doi.org/10.1002/essoar.10501482.1, 2019.
Dixon, B. C., Tyler, J. J., Lorrey, A. M., Goodwin, I. D., Gergis, J., and Drysdale, R. N.: Low-resolution Australasian palaeoclimate records of the last 2000 years, Clim. Past, 13, 1403–1433, https://doi.org/10.5194/cp-13-1403-2017, 2017.
Emile-Geay, J., Cobb, K. M., Mann, M. E., and Wittenberg, A. T.: Estimating central equatorial pacific SST variability over the past millennium. Part II: Reconstructions and implications, J. Climate, 26, 2329–2352, https://doi.org/10.1175/JCLI-D-11-00511.1, 2013.
Fletcher, M. S., Benson, A., Bowman, D. M. J. S., Gadd, P. S., Heijnis, H., Mariani, M., Saunders, K. M., Wolfe, B. B., and Zawadzki, A.: Centennial-scale trends in the Southern Annular Mode revealed by hemisphere-wide fire and hydroclimatic trends over the past 2400 years, Geology, 46, 363–366, https://doi.org/10.1130/G39661.1, 2018.
Fluin, J., Gell, P., Haynes, D., Tibby, J., and Hancock, G.: Palaeolimnological evidence for the independent evolution of neighbouring terminal lakes, the Murray Darling Basin, Australia, Hydrobiologia, 591, 117–134, https://doi.org/10.1007/s10750-007-0799-y, 2007.
Gallant, A. J. E., Kiem, A. S., Verdon-Kidd, D. C., Stone, R. C., and Karoly, D. J.: Understanding hydroclimate processes in the Murray-Darling Basin for natural resources management, Hydrol. Earth Syst. Sci., 16, 2049–2068, https://doi.org/10.5194/hess-16-2049-2012, 2012.
Gallant, A. J. E., Phipps, S. J., Karoly, D. J., Mullan, A. B., and Lorrey, A. M.: Nonstationary Australasian teleconnections and implications for paleoclimate reconstructions, J. Climate, 26, 8827–8849, https://doi.org/10.1175/JCLI-D-12-00338.1, 2013.
Gebbie, G. and Huybers, P.: The little ice age and 20th-century deep pacific cooling, Science, 363, 70–74, https://doi.org/10.1126/science.aar8413, 2019.
Gillanders, B. M. and Munro, A. R.: Hypersaline waters pose new challenges for reconstructing environmental histories of fish based on otolith chemistry, Limnol. Oceanogr., 57, 1136–1148, https://doi.org/10.4319/lo.2012.57.4.1136, 2012.
Gouramanis, C., Wilkins, D., and De Deckker, P.: 6000 years of environmental changes recorded in Blue Lake, South Australia, based on ostracod ecology and valve chemistry, Palaeogeogr. Palaeocl., 297, 223–237, https://doi.org/10.1016/j.palaeo.2010.08.005, 2010.
Haese, R., Murray, E., and Wallace, L.: Nutrient sources, water quality, and biogeochemical processes in the Coorong,South Australia, Geoscience Australia, ISBN 978-1-921498-78-7, 2009.
Hart, B., Walker, G., Katupitiya, A., and Doolan, J.: Salinity management in the Murray-Darling Basin, Water, 12, 1829, 2020.
Haverd, V., Raupach, M. R., Briggs, P. R., Canadell, J. G., Davis, S. J., Law, R. M., Meyer, C. P., Peters, G. P., Pickett-Heaps, C., and Sherman, B.: The Australian terrestrial carbon budget, Biogeosciences, 10, 851–869, https://doi.org/10.5194/bg-10-851-2013, 2013.
Hendon, H. H., Thompson, D. W. J., and Wheeler, M. C.: Australian rainfall and surface temperature variations associated with the Southern Hemisphere annular mode, J. Climate, 20, 2452–2467, https://doi.org/10.1175/JCLI4134.1, 2007.
Ho, M., Kiem, A. S., and Verdon-Kidd, D. C.: A paleoclimate rainfall reconstruction in the Murray-Darling Basin (MDB), Australia: 2. Assessing hydroclimatic risk using paleoclimate records of wet and dry epochs, Water Resour. Res., 51, 8380–8396, https://doi.org/10.1111/j.1752-1688.1969.tb04897.x, 2015.
Hogg, A. G., Heaton, T. J., Hua, Q., Palmer, J. G., Turney, C. S., Southon, J., Bayliss, A., Blackwell, P. G., Boswijk, G., Bronk Ramsey, C., Pearson, C., Petchey, F., Reimer, P., Reimer, R., and Wacker, L.: SHCal20 Southern Hemisphere Calibration, 0–55 000 years cal BP, Radiocarbon, 62, 1–20, https://doi.org/10.1017/rdc.2020.59, 2020.
Holgate, C., Evans, J. P., Taschetto, A. S., Gupta, A. S., and Santoso, A.: The Impact of Interacting Climate Modes on East Australian Precipitation Moisture Sources, J. Climate, 35, 3147–3159, https://doi.org/10.1175/JCLI-D-21-0750.1, 2022.
Jansen, E., Overpeck, J., Briffa, K. R., Duplessy, J.-C., Joos, F., Masson-Delmotte, V., Olago, D., Otto-Bliesner, B., Peltier, W. R., Rahmstorf, S., Ramesh, R., Raynaud, D., Rind, D., Solomina, O., Villalba, R., and Zhang, D.: Paleoclimate, in: Climate Change 2007: The Physical Science Basis. Contribution of Working Group I to the Fourth Assessment Report of the Intergovernmental Panel on Climate Change, edited by: Solomon, S., Qin, D., Manning, M., Chen, Z., Marquis, M., Averyt, K., Tignor, M., and Miller, H., Cambridge University Press, Cambridge, United Kingdom and New York, NY, USA, 433–497, ISBN 978 0521 88009-1, 2007.
Jones, R. N., McMahon, T. A., and Bowler, J. M.: Modelling historical lake levels and recent climate change at three closed lakes, Western Victoria, Australia (c.1840–1990), J. Hydrol., 246, 159–180, https://doi.org/10.1016/S0022-1694(01)00369-9, 2001.
Kaufman, D., McKay, N., Routson, C., Erb, M., Dätwyler, C., Sommer, P. S., Heiri, O., and Davis, B.: Holocene global mean surface temperature, a multi-method reconstruction approach, Sci. Data, 7, 1–13, https://doi.org/10.1038/s41597-020-0530-7, 2020.
Kiem, A. S., Johnson, F., Westra, S., van Dijk, A., Evans, J. P., O'Donnell, A., Rouillard, A., Barr, C., Tyler, J., Thyer, M., Jakob, D., Woldemeskel, F., Sivakumar, B., and Mehrotra, R.: Natural hazards in Australia: droughts, Climatic Change, 139, 37–54, https://doi.org/10.1007/s10584-016-1798-7, 2016.
King, A. D., Pitman, A. J., Henley, B. J., Ukkola, A. M., and Brown, J. R.: The role of climate variability in Australian drought, Nat. Clim. Change, 10, 173–183, https://doi.org/10.1038/s41558-020-0712-5, 2020.
Kingsford, R. T., Walker, K. F., Lester, R. E., Young, W. J., Fairweather, P. G., Sammut, J., and Geddes, M. C.: A ramsar wetland in crisis the coorong, lower lakes and murray mouth, Australia, Mar. Freshwater Res., 62, 255–265, https://doi.org/10.1071/MF09315, 2011.
Krull, E., Haynes, D., Lamontagne, S., Gell, P., McKirdy, D., Hancock, G., McGowan, J., and Smernik, R.: Changes in the chemistry of sedimentary organic matter within the Coorong over space and time, Biogeochemistry, 92, 9–25, https://doi.org/10.1007/s10533-008-9236-1, 2009.
Lower, C. S., Cann, J. H., and Haynes, D.: Microfossil evidence for salinity events in the Holocene Coorong Lagoon, South Australia, Aust. J. Earth Sci., 60, 573–587, https://doi.org/10.1080/08120099.2013.823112, 2013.
Ma, X., Huete, A., Cleverly, J., Eamus, D., Chevallier, F., Joiner, J., Poulter, B., Zhang, Y., Guanter, L., Meyer, W., Xie, Z., and Ponce-Campos, G.: Drought rapidly diminishes the large net CO2 uptake in 2011 over semi-arid Australia, Sci. Rep.-UK, 6, 1–9, https://doi.org/10.1038/srep37747, 2016.
Maheshwari, B. L., Walker, K. F., and McMahon, T. A.: Effects of regulation on the flow regime of the river Murray, Australia, Regul. River., 10, 15–38, https://doi.org/10.1002/rrr.3450100103, 1995.
Mann, M. E., Zhang, Z., Rutherford, S., Bradley, R. S., Hughes, M. K., Shindell, D., Ammann, C., Faluvegi, G., and Fenbiao, N.: Global Signatures and Dynamical Origins of the Little Ice Age and Medieval Climate Anomaly, Science, 326, 1256–1260, https://doi.org/10.1126/science.1177303, 2018.
Marshall, G. J.: Trends in the Southern Annular Mode from observations and reanalyses, J. Climate, 16, 4134–4143, https://doi.org/10.1175/1520-0442(2003)016<4134:TITSAM>2.0.CO;2, 2003.
McGowan, H. A., Marx, S. K., Denholm, J., Soderholm, J., and Kamber, B. S.: Reconstructing annual inflows to the headwater catchments of the Murray River, Australia, using the Pacific Decadal Oscillation, Geophys. Res. Lett., 36, 1–5, https://doi.org/10.1029/2008GL037049, 2009.
McKirdy, D. M., Thorpe, C. S., Haynes, D. E., Grice, K., Krull, E., Halverson, G. P., and Webster, L. J.: The biogeochemical evolution of the Coorong during the mid- to late Holocene: An elemental, isotopic and biomarker perspective, Org. Geochem., 41, 96–110, 2010.
Moros, M., De Deckker, P., Jansen, E., Perner, K., and Telford, R. J.: Holocene climate variability in the Southern Ocean recorded in a deep-sea sediment core off South Australia, Quaternary Sci. Rev., 28, 1932–1940, https://doi.org/10.1016/j.quascirev.2009.04.007, 2009.
Mosley, L., Priestley, S., Brookes, J., Dittmann, S., Farkaš, J., Farrell, M., Ferguson, A., Gibbs, M., Hipsey, M., Leslie, J. , Huang, O., Lam-Gordillo, O., Simpson, S., Teasdale, P., Tyler, J., Waycott, M., and Welsh, D.: Coorong water quality synthesis with a focus on the drivers of eutrophication, http://www.goyderinstitute.org (last access: 21 May 2021), 2020.
Mosley, L., Priestley, S., Brookes, J., Dittmann, S., Farkaš, J., Farrell, M., Ferguson, A., Gibbs, M., Hipsey, M., Huang, O., Lam-Gordillo, O., Simpson, S., Tyler, J., Waycott, M., and Welsh, D.: Extreme eutrophication and salinisation in the Coorong estuarine-lagoon ecosystem of Australia's largest river basin (Murray-Darling), Mar. Pollut. Bull., 188, 114648, https://doi.org/10.1016/j.marpolbul.2023.114648, 2023.
Murray-Darling Basin (MDBA): The Murray-Darling Basin and why it's important, https://www.mdba.gov.au/importance-murray-darling-basin, last access: 17 May 2021.
Murray-Wallace, C. V.: Quaternary History of the Coorong Coastal Plain, Southern Australia, Springer, ISBN 9783319893419, 2018.
Neukom, R. and Gergis, J.: Southern Hemisphere high-resolution palaeoclimate records of the last 2000 years, The Holocene, 22, 501–524, https://doi.org/10.1177/0959683611427335, 2011.
Ngarrindjeri Nation and Hemming, S.: Ngarrindjeri Nation Yarrluwar-Ruwe Plan: Caring for Ngarrindjeri Country and Culture: Kungun Ngarrindjeri Yunnan (Listen to Ngarrindjeri People Talking), in: Natural History of the Coorong, Lower Lakes and Murray Mouth Region (Yarluwar-Ruwe), edited by: Mosley, L., Ye, Q., Shepherd, S., Hemming, S., and Fitzpatrick, R., University of Adelaide Press, Adelaide, Australia, 3–21, ISBN 9781925261806, 2019.
O'Donnell, A. J., Mccaw, W. L., Cook, E. R., and Grierson, P. F.: Megadroughts and pluvials in southwest Australia: 1350–2017 CE, Clim. Dynam., 57, 1817–1831, https://doi.org/10.1007/s00382-021-05782-0, 2021.
Paton, D. C., Rogers, D. J., Hill, B. M., Bailey, C. P., and Ziembicki, M.: Temporal changes to spatially stratified waterbird communities of the Coorong, South Australia: Implications for the management of heterogenous wetlands, Anim. Conserv., 12, 408–417, https://doi.org/10.1111/j.1469-1795.2009.00264.x, 2009.
Perner, K., Moros, M., De Deckker, P., Blanz, T., Wacker, L., Telford, R., Siegel, H., Schneider, R., and Jansen, E.: Heat export from the tropics drives mid to late Holocene palaeoceanographic changes offshore southern Australia, Quaternary Sci. Rev., 180, 96–110, https://doi.org/10.1016/j.quascirev.2017.11.033, 2018.
Poulter, B., Frank, D., Ciais, P., Myneni, R. B., Andela, N., Bi, J., Broquet, G., Canadell, J. G., Chevallier, F., Liu, Y. Y., Running, S. W., Sitch, S., and Van Der Werf, G. R.: Contribution of semi-arid ecosystems to interannual variability of the global carbon cycle, Nature, 509, 600–603, https://doi.org/10.1038/nature13376, 2014.
Priestley, S. C., Tyler, J., Liebelt, S. R., Mosley, L. M., Wong, W. W., Shao, Y., Woolston, Z., Farrell, M., Welsh, D. T., Brookes, J. D., and Collins, A. S.: N and C isotope variations along an extreme eutrophication and salinity gradient in the Coorong Lagoon, South Australia, Front. Earth Sci., 9, 1345, https://doi.org/10.3389/feart.2021.727971, 2022.
R Core Team: R: A language and environment for statistical computing, R Foundation for Statistical Computing, Vienna, Austria, https://www.R-project.org/, last access 17 May 2021.
Reeves, J. M., Haynes, D., García, A., and Gell, P. A.: Hydrological Change in the Coorong Estuary, Australia, Past and Present: Evidence from Fossil Invertebrate and Algal Assemblages, Estuar. Coast., 38, 2101–2116, https://doi.org/10.1007/s12237-014-9920-4, 2015.
Risbey, J. S., Pook, M. J., McIntosh, P. C., Wheeler, M. C., and Hendon, H. H.: On the remote drivers of rainfall variability in Australia, Mon. Weather Rev., 137, 3233–3253, https://doi.org/10.1175/2009MWR2861.1, 2009.
Rustic, G. T., Koutavas, A., Marchitto, T. M., and Linsley, B. K.: Dynamical excitation of the tropical Pacific Ocean and ENSO variability by Little Ice Age cooling, Science, 350, 1537–1541, https://doi.org/10.1126/science.aac9937, 2015.
Saunders, K. M. and Taffs, K. H.: Palaeoecology: A tool to improve the management of Australian estuaries, J. Environ. Manage., 90, 2730–2736, https://doi.org/10.1016/j.jenvman.2009.03.001, 2009.
Scanes, E., Scanes, P. R., and Ross, P. M.: Climate change rapidly warms and acidifies Australian estuaries, Nat. Commun., 11, 1–11, https://doi.org/10.1038/s41467-020-15550-z, 2020.
Shao, Y., Farkaš, J., Holmden, C., Mosley, L., Kell-Duivestein, I., Izzo, C., Reis-Santos, P., Tyler, J., Törber, P., Frýda, J., Taylor, H., Haynes, D., Tibby, J., and Gillanders, B. M.: Calcium and strontium isotope systematics in the lagoon-estuarine environments of South Australia: Implications for water source mixing, carbonate fluxes and fish migration, Geochim. Cosmochim. Ac., 239, 90–108, https://doi.org/10.1016/j.gca.2018.07.036, 2018.
Shao, Y., Farkaš, J., Mosley, L., Tyler, J., Wong, H., Chamberlayne, B. K., Raven, M., Samanta, M., Holmden, C., Gillanders, B. M., Kolevica, A., and Eisenhauer, A.: Impact of salinity and carbonate saturation on stable Sr isotopes () in a lagoon-estuarine system, Geochim. Cosmochim. Ac., 293, 461–476, https://doi.org/10.1016/j.gca.2020.11.014, 2021.
Soulet, G.,: Methods and codes for reservoir-atmosphere 14C age offset calculations, Quat. Geochronol., 29, 97–103, https://doi.org/10.1016/j.quageo.2015.05.023, 2015.
Tibby, J., Reid, M. A., Fluin, J., Hart, B. T., and Kershaw, A. P.: Assessing long-term pH change in an Australian river catchment using monitoring and palaeolimnological data, Environ. Sci. Technol., 37, 3250–3255, https://doi.org/10.1021/es0263644, 2003.
Tibby, J., Tyler, J. J., and Barr, C.: Post little ice age drying of eastern Australia conflates understanding of early settlement impacts, Quaternary Sci. Rev., 202, 45–52, https://doi.org/10.1016/j.quascirev.2018.10.033, 2018.
Tozer, C. R., Vance, T. R., Roberts, J. L., Kiem, A. S., Curran, M. A. J., and Moy, A. D.: An ice core derived 1013 year catchment-scale annual rainfall reconstruction in subtropical eastern Australia, Hydrol. Earth Syst. Sci., 20, 1703–1717, https://doi.org/10.5194/hess-20-1703-2016, 2016.
Tulipani, S., Grice, K., Krull, E., Greenwood, P., and Revill, A. T.: Salinity variations in the norther Coorong Lagoon, South Australia: Significant changes in the ecosystem following human alteration to the natural water regime, Org. Geochem., 75, 74–86, 2014.
Tyler, J. J., Mills, K., Barr, C., Sniderman, J. M. K., Gell, P. A., and Karoly, D. J.: Identifying coherent patterns of environmental change between multiple, multivariate records: an application to four 1000 year diatom records from Victoria, Australia, Quaternary Sci. Rev., 119, 94–105, https://doi.org/10.1016/j.quascirev.2015.04.010, 2015.
Ummenhofer, C. C., England, M. H., Mclntosh, P. C., Meyers, G. A., Pook, M. J., Risbey, J. S., Gupta, A. S., and Taschetto, A. S.: What causes southeast Australia's worst droughts?, Geophys. Res. Lett., 36, 1–5, https://doi.org/10.1029/2008GL036801, 2009.
Vance, T. R., Van Ommen, T. D., Curran, M. A. J., Plummer, C. T., and Moy, A. D.: A millennial proxy record of ENSO and eastern Australian rainfall from the law dome ice core, east Antarctica, J. Climate, 26, 710–725, https://doi.org/10.1175/JCLI-D-12-00003.1, 2013.
Verdon, D. and Franks, S. W.: Long-term drought risk assessment in the Lachlan River Valley – a paleoclimate perspective, Australas. J. Water Resour., 11, 145–152, https://doi.org/10.1080/13241583.2007.11465319, 2007.
Wanamaker, A. D., Butler, P. G., Scourse, J. D., Heinemeier, J., Eiríksson, J., Knudsen, K. L., and Richardson, C. A.: Surface changes in the North Atlantic meridional overturning circulation during the last millennium, Nat. Commun., 3, 899, https://doi.org/10.1038/ncomms1901, 2012.
Wang, G. and Hendon, H. H.: Sensitivity of Australian rainfall to inter-El Niño variations, J. Climate, 20, 4211–4226, https://doi.org/10.1175/JCLI4228.1, 2007.
Wells, F. E. and Threlfall, T. J.: Salinity and temperture tolerance of Hydrococcus brazieri (T. Woods, 1876) and Arthritica semen (Menke, 1843) from the Peel-Harvey estuarine system, Western Australia, J. Malacol. Soc. Aust., 5, 151–156, 1982.
Wilkins, D., De Deckker, P., Fifield, L. K., Gouramanis, C., and Olley, J.: Comparative optical and radiocarbon dating of laminated Holocene sediments in two maar lakes: Lake Keilambete and Lake Gnotuk, south-western Victoria, Australia, Quat. Geochronol., 9, 3–15, https://doi.org/10.1016/j.quageo.2012.01.008, 2012.
Wilkins, D., Gouramanis, C., De Deckker, P., Fifield, L. K., and Olley, J.: Holocene lake-level fluctuations in Lakes Keilambete and Gnotuk, southwestern Victoria, Australia, The Holocene, 23, 784–795, https://doi.org/10.1177/0959683612471983, 2013.