the Creative Commons Attribution 4.0 License.
the Creative Commons Attribution 4.0 License.
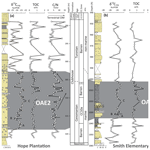
Enhanced terrestrial runoff during Oceanic Anoxic Event 2 on the North Carolina Coastal Plain, USA
Christopher M. Lowery
Jean M. Self-Trail
Craig D. Barrie
A global increase in the strength of the hydrologic cycle drove an increase in the flux of terrigenous sediments into the ocean during the Cenomanian–Turonian Oceanic Anoxic Event 2 (OAE2) and was an important mechanism driving nutrient enrichment and thus organic carbon burial. This global change is primarily known from isotopic records, but global average data do not tell us anything about changes at any particular location. Reconstructions of local terrigenous flux can help us understand the role of regional shifts in precipitation in driving these global trends. The proto-North Atlantic basin was one of the epicenters of enhanced organic carbon burial during OAE2, so constraining terrigenous flux is particularly important in this region; however, few local records exist. Here, we present two new OAE2 records from the Atlantic Coastal Plain of North Carolina, USA, recognized with calcareous nannoplankton biostratigraphy and organic carbon isotopes. We use carbon nitrogen ratios to constrain the relative contribution of marine and terrestrial organic matter; in both cores we find an elevated contribution from vascular plants beginning just before OAE2 and continuing through the event, indicating a locally strengthened hydrologic cycle. Terrigenous flux decreased during the brief change in carbon isotope values known as the Plenus carbon isotope excursion; it then increased and remained elevated through the latter part of OAE2. Total organic carbon (TOC) values reveal relatively low organic carbon burial in the inner shelf, in contrast to black shales known from the open ocean. Organic carbon content on the shelf appears to increase in the offshore direction, highlighting the need for cores from the middle and outer shelf.
The Cretaceous was characterized by intermittent periods of enhanced organic carbon burial linked to widespread black shale deposition and anoxia, termed oceanic anoxic events (OAEs; Schlanger and Jenkyns, 1976; Jenkyns, 2010). Although OAEs were originally defined by the widespread occurrence of black shales (Schlanger and Jenkyns, 1976), they were soon found to be associated with positive carbon isotope excursions driven by the excess global burial of organic carbon representing a perturbation of the global carbon cycle (Scholle and Arthur, 1980; Arthur et al., 1987; Jenkyns, 2010; Owens et al., 2017). OAEs eventually became linked with the emplacement of large igneous provinces (Tarduno et al., 1991; Whitechurch et al., 1992; Leckie et al., 2002; Snow et al., 2005; Turgeon and Creaser, 2008; Monteiro et al., 2012; McAnena et al., 2013), suggesting a causal mechanism for enhanced organic carbon burial. In the case of the Cenomanian–Turonian OAE2 (∼ 94 Ma), the emplacement of the Caribbean Large Igneous Province (e.g., Snow et al., 2005) is associated with significant warming (e.g., Friedrich et al., 2012) and resulted in a strengthening of the hydrological cycle and an increase in the flux of nutrients to the oceans (Blättler et al., 2011; Pogge von Strandmann et al., 2013).
Trends in the stable carbon isotope ratios (δ13C) of the global carbon pool reveal global changes in organic carbon burial rates (e.g., Jenkyns, 2010) but do not tell us anything about where that organic matter was buried. This is important because local organic matter enrichment can vary significantly in both timing (e.g., Tsikos et al., 2004) and magnitude (e.g., Owens et al., 2018) during an OAE. Similarly, the calcium isotope proxy used by Blättler et al. (2011) and the lithium isotope proxy used by Pogge von Strandmann et al. (2013) to determine changes in global terrigenous flux to the oceans do not tell us anything about local patterns of terrigenous runoff. Presumably, like organic carbon burial, the hydrologic cycle did not increase uniformly, but instead some regions experienced a greater change than others. Unfortunately, few local records of changes in the hydrologic cycle during OAE2 have been documented. Van Helmond et al. (2014) used pollen assemblages, freshwater-tolerant marine dinoflagellates, and biomarker data from the Bass River core (Ocean Drilling Program Site 174X) on the coastal plain of New Jersey, USA, to document local warming associated with an enhanced contribution of terrestrial organic matter during OAE2. While this result clearly indicates a stronger hydrologic cycle during OAE2, it only represents a single locality. Similar work from Wunstorf, Germany, in the Lower Saxony Basin, reveals a clear association between terrigenous flux (measured by palynology and biomarker data) and black shale development, but this association is not limited to OAE2, with additional intervals of elevated terrigenous input and black shale deposition continuing after the end of the carbon isotope excursion (van Helmond et al., 2015). In the Western Interior Seaway of North America, increases in kaolinite (a clay mineral formed in humid environments) during OAE2 may be the result of wetter conditions, but these trends may also be caused by shifting sediment source areas (Leckie et al., 1998). Overall, these existing records paint an incomplete picture.
To fully understand these trends, it is essential to develop similar datasets from additional localities. Such work will allow a more geographically complete understanding of changes in precipitation during OAE2 and thus provide a window into the mechanisms that drove hydroclimate during the hottest part of the Cretaceous greenhouse. Here, we present two new OAE2 sections from cores drilled by the United States Geological Survey (USGS) on the coastal plain of North Carolina at the Atlantic margin of North America (Fig. 1). We use organic carbon isotopes and calcareous nannoplankton biostratigraphy to identify the OAE2 interval and organic carbon nitrogen (C N) molar ratios to detect changes in terrigenous flux. These cores are only the second and third OAE2 intervals described on the Atlantic Coastal Plain after the Bass River core (Bowman and Bralower, 2005; van Helmond et al., 2014) and thus also provide important context for the response of the inner shelf to OAE2, filling in an important gap in an important region (e.g., Owens et al., 2018) during this well-studied time interval.
Cenomanian and Turonian sediments of the Atlantic Coastal Plain of the United States (Fig. 1) are part of a sequence of strata that accumulated since the rifting of the Atlantic began in the Early Jurassic. However, study of the marine units in these sediments is difficult due to the absence of outcrops of this age and environment as well as their moderate to large burial depths in the Carolinas and Georgia (Sohl and Owens, 1991). Thus, their study is restricted to the limited number of available cores and/or cuttings, and their regional interpretations are often based on geophysical data obtained from water wells and scattered oil and gas test wells.
To the south, initial subsurface work in Florida and Georgia followed the nomenclature of the Gulf Coastal Plain. Sediments in Georgia were variously attributed to the Cenomanian Woodbine Formation, the Cenomanian–Turonian Eagle Ford Formation, and the Cenomanian–Turonian Tuscaloosa Formation (Applin and Applin, 1944; Richards, 1945). Applin and Applin (1947) later introduced the name Atkinson Formation, with three unnamed members (upper, middle, and lower) for certain marine and non-marine sediments in the subsurface of southern Alabama, southern Georgia, and northern Florida. They correlated the lower member of the Atkinson with basal non-marine sands and shales of the coastal plain of Georgia, which they considered to be Cenomanian in age, and the middle member of the Atkinson to the Tuscaloosa Marine Shale, which they considered to be Cenomanian–Turonian in age (Applin and Applin, 1967).
Early work in South Carolina by Cooke (1936), Dorf (1952), and Heron (1958) considered outcrops of the Middendorf Formation to be Cenomanian in age based largely on stratigraphic position and long-ranging pollen and/or mollusks. Similarly, outcrops of the largely non-marine Cape Fear Formation in North Carolina were attributed to the Cenomanian (Stephenson, 1912; Cooke, 1936). Outcrops thought to be Turonian in age from both states were largely assigned to the Black Creek Formation.
A shift in thinking regarding stratigraphic nomenclature was spurred by examination of sediments from the Clubhouse Crossroads no. 1 core by Hazel et al. (1977), who found clear evidence of true Cenomanian–Turonian marine sediments in the later-defined Clubhouse Formation near the base of the downdip coastal plain section. Calcareous nannofossils and foraminifera of Cenomanian and Turonian age were identified in the Clubhouse Formation (Hazel et al., 1977; Hattner and Wise, 1980; Valentine, 1984) and correlated with cuttings containing calcareous nannofossils from the Fripp Island, SC, deepwater well (Valentine, 1984). In North Carolina, Zarra (1989) reinterpreted the work of Spangler (1950) using both foraminifera and sequence stratigraphic concepts, positively identifying Cenomanian and Turonian sediments from the Esso no. 1 core and from cuttings of the Mobile no. 1, Mobile no. 2, Mobile no. 3, and Marshall Collins no. 1 test wells. He used sediment and well log analysis to identify marginal marine and inner shelf facies in the lower–middle Cenomanian and middle Turonian section, with a highstand in the upper Cenomanian. These cores all contained a diverse assemblage of planktic foraminifera, including species belonging to Rotalipora, Praeglobotruncana, Dicarinella, Whiteinella, and Guembelitria (Zarra, 1989).
This reevaluation ultimately resulted in the formal designation of the Cenomanian–Turonian Clubhouse Formation (Gohn, 1992) in the Clubhouse Crossroads core. At the type locality, the Clubhouse Formation consists of gray to gray–green, fine- to medium- grained, micaceous, muddy sands with flaser to lenticular bedding and common bioturbation. Sequence stratigraphic analysis suggests that deposition occurred in a shelf environment proximal to the shoreline and that these sediments represent latest Cenomanian–earliest Turonian sea level rise prior to the early Turonian highstand event (Aleman Gonzalez et al., 2020). The subsurface extent of this formation has now been documented across much of South Carolina and North Carolina (Weems et al., 2007, 2019; Aleman Gonzalez et al., 2020).
To the north, published documentation of marine Cenomanian–Turonian sediments from the mid-Atlantic region appears to be limited to the E.G. Taylor no. 1-G well on the eastern shore of Virginia. Valentine (1984) reports the presence of Rotalipora greenhornensis, which went extinct in the latest Cenomanian, from one sample at 463.3 m (1520 ft).
Cenomanian–Turonian sediments of the northeast Atlantic Coastal Plain consist of the subsurface Bass River Formation and its correlative up-dip equivalent, the Raritan Formation in Maryland, New Jersey, and Delaware. The Bass River Formation is herein considered to be correlative with the Clubhouse Formation of the southeastern Atlantic Coastal Plain. The Bass River Formation was first described by Petters (1976) from the TC16 well in Bass River Township, New Jersey. It is considered to be the fully marine equivalent of the Raritan Formation and is differentiated by its common shell material and deeper-water depositional environment (Miller et al., 1998). The Bass River Formation has variously been assigned a late Cenomanian to early Turonian age in a variety of cores and wells based on foraminifera (Petters, 1976, 1977; Miller et al., 1998; Sikora and Olsson, 1991), calcareous nannofossils (Valentine, 1984; Miller et al., 1998; Self-Trail and Bybell, 1995), and ostracods (Gohn, 1995). Miller et al. (2004) document the Bass River Formation as deposited predominantly in inner shelf to middle shelf paleodepths.
3.1 Study sites
The Hope Plantation core (BE-110-2004) was drilled by the USGS in April to May 2004 in Bertie County, North Carolina, on the property of Hope Plantation (36.0323∘ N; 78.0192∘ W) (Fig. 1). The hole was drilled as a stratigraphic test for Atlantic Coastal Plain aquifers and was continuously cored to a total depth of 333.6 m (1094.5 ft) below the land surface. A suite of wireline logs, including natural gamma ray and resistivity logs, were collected at the completion of drilling. Preliminary biostratigraphy placed the marine Cenomanian–Turonian boundary interval between approximately 182.8 and 228.6 m (600–750 ft). A summary of the general stratigraphy, downhole logging, and core images can be found in Weems et al. (2007).
The Smith Elementary School core (CR-675) was drilled by the USGS in February and March 2006 in Craven County, NC, on the grounds of the nominate school (35.2511∘ N; 77.2903∘ W) (Fig. 1). This hole was also drilled as a stratigraphic test for coastal plain aquifers and was continuously cored to a total depth of 323.1 m (1060.0 ft). Difficulties with the wireline tools and borehole stability limited the collection of geophysical logs, and only a partial natural gamma ray log exists for the Clubhouse Formation in this core hole. There, the marine interval that spans the Cenomanian–Turonian boundary is between 288.3 and 323.1 m of depth (945.9–1060.0 ft). Both cores are stored at the North Carolina Geological Survey Coastal Plain core storage facility in Raleigh, NC, where we sampled them in May 2019.
3.2 Calcareous nannofossils
A total of 110 samples from Hope Plantation and 84 samples from Smith Elementary School were examined for calcareous nannofossil content. Samples were taken from the central portion of broken core in order to avoid contamination from drilling fluid. Smear slides were prepared using the standard techniques of Bown and Young (1998) in samples with low total organic carbon (TOC); samples with increased TOC were prepared using the techniques of Shamrock et al. (2015) and Shamrock and Self-Trail (2016). Coverslips were affixed using Norland Optical Adhesive 61. Calcareous nannofossils were examined using a Zeiss Axioplan 2 transmitted light microscope at 1250× magnification under cross-polarized light. Light microscope images were taken using a Powershot G4 camera with a Zeiss phototube adaptor. Specimens were identified to the species level and correlated with the zonation schemes of Sissinghi (1977) and Burnett (1998), as modified by Corbett et al. (2014) for shelf settings.
3.3 Foraminifera
A total of 90 samples were prepared for examination of planktic and benthic foraminifera. Approximately 15 g of material was soaked in a mixture of peroxide and borax for at least 24 h, washed over a 63 µm sieve, dried overnight in an oven, and then examined for microfossils using a Zeiss Discovery V8 light microscope.
3.4 TOC, C N, and δ13C
Core samples were analyzed for both their elemental composition (%C and %N) and organic carbon isotope signature (δ13C VPDB). To remove inorganic carbon content all of the material to be analyzed was initially washed with 1 M hydrochloric (HCl) acid. There was no anticipated inorganic nitrogen content in the samples. All of the samples were analyzed on an Elementar vario ISOTOPE select cube elemental analyzer (EA) connected to a VisION isotope ratio mass spectrometer (IRMS). The EA system follows Dumas combustion and both generates and separates the gases used for elemental composition determination and then releases the gas to the IRMS for isotopic determination. Every fifth sample was run in duplicate, and a check standard was run in triplicate every 20th sample to ensure the accuracy of the results. The elemental results were calibrated against a known sulfanilamide standard; the precision of the results is ±0.1 % or better, and variation of duplicate samples was within the range of this uncertainty. The carbon isotope results were calibrated against four known reference standards that cover the range of isotopic signatures expected in organic material (−15 ‰ to −35 ‰), and duplicates and check standards were run at the same interval as above. All of the isotopic results are reported in per mil (‰) relative to VPDB, and the precision of the results is ±0.1 ‰ or better.
4.1 Lithology
Qualitative core descriptions are summarized below and in Figs. 2 and 3. Broad paleoenvironmental interpretations are based on lithology, paleontology, and stratigraphic relationships. Benthic foraminifera, which are powerful tools to determine paleoenvironment in marginal marine settings (e.g., Tibert and Leckie, 2004), are unfortunately absent here due to poor preservation (see Sect. 4.2 below). In both cores we recognize two informal members of the Clubhouse Formation: a marine lower member characterized by bivalves, calcareous nannoplankton, finer grained sediments, thinner beds, and sedimentary features common to inner shelf environments; and a marginal marine upper member characterized by coarser grain size, thicker beds, and woody plant debris instead of calcareous marine fossils, indicating deposition in a delta front or distributary environment.
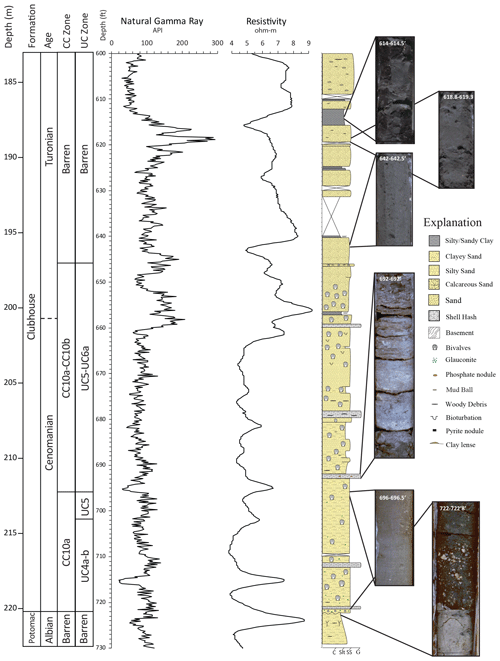
Figure 2Stratigraphic column for the Hope Plantation core with CC and UC calcareous nannoplankton biozones, natural gamma ray and resistivity logs, and representative core images. C: clay; Slt: silt; SS: sand; G: gravel.
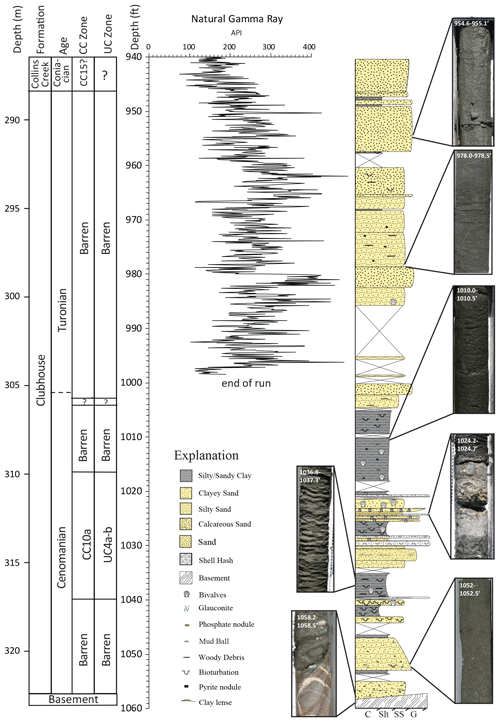
Figure 3Stratigraphic column for the Smith Elementary School core, with CC and UC calcareous nannoplankton biozones, natural gamma ray log, and representative core images.
The Clubhouse Formation in the Hope Plantation core (Fig. 2) was penetrated between 174.3 and 220.2 m below the surface. It is underlain by the floodplain paleosols of the Albian Potomac Group (Thornburg, 2008) and is overlain by undifferentiated sands and muds questionably assigned to the Cape Fear Formation (Weems et al., 2007). The Clubhouse Formation is primarily composed of clayey and silty sands punctuated by a few discrete skeletal limestones. The whole unit coarsens upward from clayey sands (from the base of the formation to about 210.0 m) to silty sands (from about 210.0 to about 201.2 m) to more pure sands (from about 201.2 m to the top of the formation, although natural gamma ray peaks suggest the inclusion of some clay in parts of this interval). This upper change corresponds to a clear change in gamma ray log response that characterizes most of the informal marginal marine upper member.
The lower marine informal member extends from the base of the Clubhouse Formation to the highest common occurrence of bivalves and calcareous nannoplankton at around 196.9 m. Glauconite occurs from the base of the informal marine unit up to about 211.2 m. Four decimeter-scale skeletal limestones composed of broken bivalves occur roughly evenly spaced through this informal member. Widely scattered woody debris is found between 210.6 and 206.0 m. Definite bioturbation is rare but evident between 203.6 and 201.2 m, just below the shift in lithology from silty sand to cleaner sand. Bivalves occur throughout the informal marine member in varying abundance. The marginal marine upper informal member of the Clubhouse Formation is characterized by massive sand interbedded with variably thick beds of massive silty clay, an increasing abundance of woody debris above 189.0 m, and the occurrence of centimeter-scale mud balls above 185.6 m. A single thin bed containing bivalves occurs at 196.9 m. Given the more terrestrial features, cleaner sands, and thin clay interbeds of the upper informal member of the Clubhouse Formation, we suggest that these sediments were deposited in a non-marine or marginal marine environment such as a distributary mouth bar or interdistributary bay system in the upper part of the Clubhouse Formation.
In the Smith Elementary core (Fig. 3), the Clubhouse Formation occurs between 288.5 and 322.7 m of depth. Its basal contact with underlying gneiss is marked by a fault, with an angular contact (∼ 45∘ to vertical in the core) and slickensides (Weems et al., 2007). This fault is overlain by an approximately 15 cm thick interval of dolomitic sand. The lithology of the Clubhouse Formation in the Smith Elementary core is overall more fine-grained than that of the Hope Plantation, with a lower fining-upwards interval, muds and limestones in the middle, and a coarsening-upward interval that extends to the unconformable upper contact with the Santonian marginal marine Collins Creek Formation.
The lower marine informal member of the Clubhouse Formation in the Smith Elementary core (322.7–∼ 305.0 m) contains a more varied lithology than that of the Hope Plantation core. The basal interval in this member is a 2.6 m thick package of massive, coarsening-upward and then fining-upward, clayey to silty, glauconite-bearing sandstone separated by a thin silty claystone above a ∼ 35 cm core gap. Coring gaps of this scale are more common in the Smith Elementary core and are associated with the contacts between sand and clay intervals. A single burrow occurs in the upper sandstone bed, and glauconite decreases up-section. The overlying interval is composed of 2.8 m of bioturbated clay and silty clay, with two ∼ 30 cm thick silty sandstones with abundant burrows and rare bivalves. The upper silty claystone contains thin clay lenses. This claystone is overlain by a 4.0 m thick interval of interbedded silty to clayey sandstone, skeletal limestones composed of broken bivalve debris, including one that has been dolomitized, and a thick (∼ 80 cm) bioturbated silty claystone containing glauconite and bivalve shells. This in turn is overlain by a 5.2 m thick silty claystone with planar bedding, phosphate nodules, pyrite, and bivalve shells. The lower 3.4 m of this claystone is laminated with no visible bioturbation. Overall this interval represents a fining-upward sequence from sand to sandy silt to silty clay; the sandy clay contains thin discrete beds of coarser material, including shell hash possibly indicating deposition above a storm wave base, before deepening to uniform silty clay representing deposition on the shelf below the storm wave base at the top of the informal marine member.
The upper marginal marine informal member of the Clubhouse Formation in the Smith Elementary core (∼ 305.0–288.5 m) is composed of meter-scale beds of silty to well-sorted sandstone that generally become coarser up-section, interbedded with centimeter-scale beds of claystone. Some beds contain woody debris and pyrite. A single bivalve occurs near the very base of the member, and a few discrete burrows are observed between 294 and 292 m. Flaser bedding occurs in a clay bed at 291.7 m. The overall coarse-grained nature of these beds as well as the alternating terrestrial and marine indicators lead us to interpret this interval as being marginal marine, perhaps representing distributary mouth bars. The overlying contact with the Collins Creek Formation is marked by a readily observable unconformity.
4.2 Biostratigraphy
Calcareous nannofossil assemblages are prevalent in the Hope Plantation core (Fig. 4), with abundances ranging from rare to common and preservation from good to poor; the top of the Clubhouse Formation is barren (196.8–185.5 m) (Self-Trail et al., 2021). The base of the Clubhouse Formation is placed in the late Cenomanian zone UC4a–b of Burnett (1998) and zone CC10a of Sissinghi (1977) based on the presence of Lithraphidites acutus, whose highest occurrence (HO) at 214.0 m marks the top of zone UC4b. The absence of Cretarhabdus loriei, whose HO marks the top of UC4a, could be due to ecological exclusion from inshore environments, and thus sediments in this interval are lumped together into a combined zone (UC4a–b). A condensed (or truncated) interval from the HO of L. acutus to the HO of Helenea chiastia at 212.9 m is placed in zone UC5 (undifferentiated) and is latest Cenomanian in age. It is unclear from nannoplankton data alone whether the HO of H. chiastia is the true extinction of this taxon (and thus this level marks the latest Cenomanian) or if the absence of this species above this level is the result of poor preservation and/or ecological exclusion from the inner shelf, as increased terrigenous flux made the waters less welcoming to marine nannoplankton. We favor the latter explanation because the sample immediately above the highest H. chiastia is barren and marks the beginning of an interval characterized by poor preservation and locally barren samples. This interval, from 212.1–197.0 m, is placed in zones UC5–UC6a and CC10a–CC10b based on the absence of both H. chiastia and Eprolithus moratus, whose lowest occurrence (LO) defines the base of zone UC6b. The Cenomanian–Turonian boundary is placed at 200.3 m based on carbon isotope data (see Sect. 4.3.1).
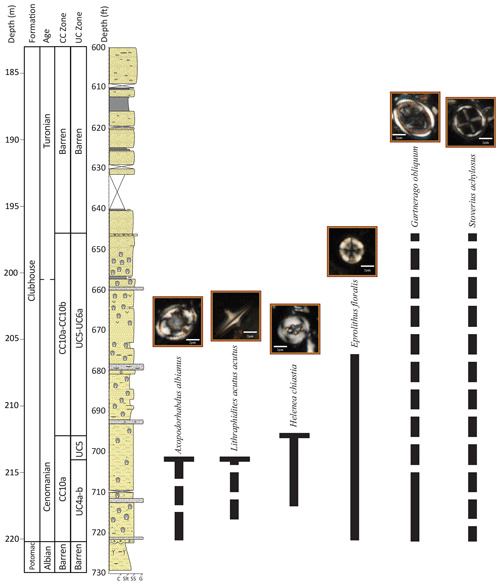
Figure 4Ranges of key calcareous nannoplankton species in the Hope Plantation core. Dashed lines indicate sporadic occurrence.
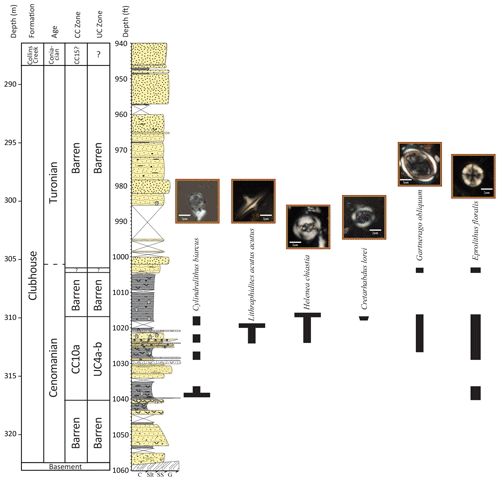
Figure 5Ranges of key calcareous nannoplankton species in the Smith Elementary School core. Dashed lines indicate sporadic occurrences.
Calcareous microfossils are only sporadically present in the Smith Elementary School sediments (Self-Trail et al., 2021) (Fig. 5). Even though the presence of glauconite, burrowing, fish debris, and scattered shell fragments indicates deposition in a marine environment, intervals barren of calcareous nannoplankton are common and extensive from 322.5–317.3 and 309.9–290.4 m (Fig. 5). The presence of Cylindralithus biarcus at 316.4 m, the HO of L. acutus at 310.9 m, and the HOs of H. chiastia and C. loriei at 310.2 m place this interval in the late Cenomanian calcareous nannofossil zone UC4a–b to zone CC10a. The rare occurrence of poorly preserved calcareous nannofossils at 305.9 m suggests continued placement in the Cenomanian or Turonian, but no diagnostic species were recovered, and thus the Cenomanian–Turonian boundary must once again be placed using carbon isotopes at 305.4 m (see Sect. 4.3.1). An unconformity at the top of the Clubhouse Formation (288.4 m) corresponds to a change from a barren interval below to a Santonian assemblage of calcareous nannofossils above.
All samples examined for planktic and benthic foraminifera were barren of whole specimens. This is unlikely to be the result of anoxia at the time of deposition, as this would not explain the lack of planktic foraminifera, which occupied a mixed layer habitat similar to the nannoplankton observed in the same interval. A few samples contained very rare fragments of both planktic and benthic foraminifera, indicating that foraminifera were present in these sections but that they were subsequently dissolved, either in situ or in the 17 years since the cores were drilled. This may be due in part to the relatively organic-rich nature of the sediments and to the presence of pyrite, both of which have been found to result in dissolution of calcareous microfossils in cored sediments of the Atlantic Coastal Plain (Self-Trail and Seefelt, 2005; Seefelt et al., 2015). However, the well-documented occurrence of planktic and benthic foraminifera in more distal coastal plain cores (e.g., Valentine, 1982, 1984; Zarra, 1989; Gohn, 1992) bodes well for future micropaleontological studies in this region.
4.3 Geochemistry
4.3.1 Carbon Isotopes
Organic carbon isotope (δ13C) data (Fig. 6) in each core show clear positive excursions associated with OAE2 in the marine interval of the Clubhouse Formation. Both isotope records display a ∼ 2 ‰ positive shift with the classic A–B–C structure of OAE2, with an initial excursion (A), a brief recovery followed by a second peak (B), and a longer plateau with a small peak (C) first described by Pratt and Threlkeld (1984) in the US Western Interior Seaway. The Hope Plantation core, which is characterized by coarser grains and a more proximal environment, has a more expanded OAE2 interval (∼ 17.4 m) compared to the somewhat more distal Smith Elementary core (∼ 10.4 m). We compare the expanded Hope Plantation carbon isotope record to other representative North American OAE2 records from the Western Interior Seaway as well as the Gulf of Mexico and Atlantic coastal plains in Fig. 7. The termination of the OAE2 carbon isotope excursion roughly corresponds to the Cenomanian–Turonian boundary (e.g., Kennedy et al., 2005) and has been used to define that level in our cores.
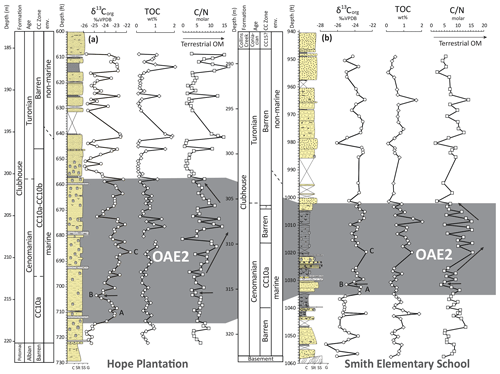
Figure 6Geochemical data from the Hope Plantation (a) and Smith Elementary School (b) cores plotted against stratigraphic columns for each. The gray shaded area represents the OAE2 interval in each core. A–B–C labels on the carbon isotope (δ13C) curve correspond to named points of the OAE carbon isotope excursion (see Fig. 7). TOC: total organic carbon; C N: carbon nitrogen ratio. Arrows indicate a brief reduction in the C N ratio coincident with the Plenus isotope excursion (“B” on the δ13C plot) and a broad increase in values during the main part of the δ13C excursion. Note the slight change in depth scale between the two cores, as the studied interval at Smith Elementary is 10 ft (3.1 m) thicker than at Hope Plantation.
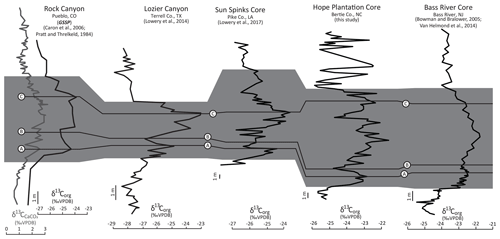
Figure 7Comparison of North American carbon isotope curves from the Cenomanian–Turonian Global Stratotype Section and Point at Rock Canyon in Pueblo, CO; Lozier Canyon in Terrell Co., TX, near the transition from the Western Interior Seaway to the Gulf of Mexico; the Sun Spinks core in Pike Co., LA, on the Gulf Coastal Plain; the Hope Plantation core from Bertie Co., NC, on the Atlantic Coastal Plain; and the Bass River core from Bass River, NJ, on the Atlantic Coastal Plain. The Rock Canyon record includes both bulk carbonate (gray line) and organic carbon (black line) isotopes; all other sites only show organic carbon isotopes. The position of the A–B–C peaks (first identified by Pratt and Threlkeld, 1984) is traced between the cores. The gray bar shows the extent of OAE2 in each core.
4.3.2 Total organic carbon
Total organic carbon data (Fig. 6) reveal relatively low enrichment in organic carbon in the Hope Plantation core: generally < 1 weight percent (wt %) TOC except for a few discrete peaks associated with woody debris. Average values are slightly higher during OAE2 (∼ 0.6 wt %) compared to background levels in the overlying interval (∼ 0.4 wt %) but just barely. Values are slightly higher overall in the Smith Elementary School core, particularly during OAE2; the upper part of the event averages about 1.0 wt % TOC.
4.3.3 Organic carbon nitrogen ratios
The ratio of total organic carbon to total nitrogen is a common proxy for the relative contributions of algae and land plants to sedimentary organic matter (e.g., Meyers, 1994, 1997, 2003). Due to differences in their composition (e.g., the abundance of cellulose in land plants) vascular plants tend to have C N ratios of 20 or greater, while algae have C N ratios of 4–10 (Meyers, 1994). Changes in the C N ratio in marine settings therefore reflect changes in the relative contribution of terrigenous organic matter to offshore areas. C N can thus be used to reconstruct changes in the hydrologic cycle, with increased C N ratios indicating a higher flux of terrestrial organic matter due to enhanced weathering (Meyers, 2003). Sediments with low TOC (< 0.3 wt %) can cause problems for C N interpretations because in such settings the proportion of inorganic nitrogen can be high enough to artificially depress the data, suggesting more marine organic matter than is really there (Meyers, 1997); our data are consistently above 0.5 wt % TOC, so this is not a concern (see Sect. 4.3.2).
C N ratios in both cores are elevated during OAE2, indicating an enhanced contribution of terrestrial organic matter driven by a strengthened hydrologic cycle (Fig. 6). In the Hope Plantation core, C N ratios increased from an average of 5.5 prior to OAE2 to 7.1 during the event, with higher values later in the event peaking around 14.4. Average values dropped back to 5.5 after OAE2, including occasional peaks reflecting the inclusion of woody plant debris visible in the core. In the Smith Elementary School core, C N ratios increased from 8.4 before OAE2 to 9.53 during OAE2, with peak values (up to 16.0) again occurring later in the event. Post-OAE2 C N values at Smith Elementary are less noisy than those at Hope Plantation and average 7.4.
5.1 Enhanced hydrologic cycle during OAE2
Our data indicate a strengthened hydrologic cycle in southeastern North America preceding the start of OAE2 and continuing through the event, in agreement with the data from van Helmond et al. (2014) some 500 km to the north. Palynological data from New Jersey agree with our bulk geochemical data in showing the highest terrigenous flux during the latter part of the OAE2 isotope excursion. The pre-event increase in terrigenous flux is an interesting parallel to records of pre-event global oxygen drawdown based on thallium isotopes (Ostrander et al., 2017), suggesting a link between weathering flux and deoxygenation, likely via enhanced delivery of nutrients to the oceans. Additionally, a drop in the C N ratio in both of our core records during the carbon isotope minimum referred to as the Plenus carbon isotope excursion (O'Connor et al., 2020) indicate relatively drier conditions at this time, a phenomenon also observed in New Jersey coincident with a decrease in temperatures (van Helmond et al., 2014). The Plenus Cold Event was originally interpreted as a global cooling event (hence the name; e.g., Gale and Christensen, 1996; Erbacher et al., 2005; Jarvis et al., 2011; Hasegawa et al., 2013; Gale, 2019). However, more detailed comparisons of temperature and carbon isotope records from a wide range of sites has demonstrated that the timing and magnitude of cooling vary significantly by location (O'Connor et al., 2020). Our results agree with those of van Helmond et al. (2014) that the Plenus interval resulted in a weaker hydrologic cycle and reduced terrigenous flux into the oceans, at least along the east coast of North America.
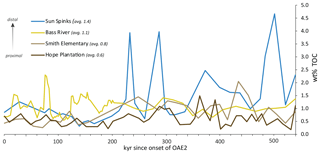
Figure 8Comparison of measured wt % TOC for the duration of OAE2 for the Sun Spinks core in Mississippi, the Bass River core in New Jersey, and the Smith Elementary School and Hope Plantation cores in North Carolina. The age model is based on the thickness of the OAE carbon isotope excursion and the orbitally tuned duration of OAE2 at the Global Stratotype Section and Point in Pueblo, CO (Sageman et al., 2006; Meyers et al., 2012) of 540 kyr, assuming a constant sedimentation rate.
5.2 OAE2 on the eastern North American shelf
The Smith Elementary School and Hope Plantation cores represent the second and third records of OAE2 on the US Atlantic Coastal Plain. As such, they provide important insight into a surprisingly understudied region. In the modern ocean, about 85 % of organic carbon burial occurs along continental margins (e.g., Burdige, 2007). A survey of all known OAE2 localities with a complete carbon isotope excursion and TOC data by Owens et al. (2018) found that there is a significant amount of “missing” organic carbon when reconstructed organic carbon burial is compared to “expected” carbon burial based on carbon isotope data. This was based on 170 sites, which, with some extrapolation, represent just 13 % of total Cenomanian–Turonian global ocean area, meaning that similar values had to be assumed for the rest of the seafloor (Owens et al., 2018). OAE2 is perhaps the best-studied event of the Cretaceous, but these results suggest a clear need for additional sites to better constrain paleoceanographic and paleoenvironmental changes during this event. By adding additional OAE2 sites on the Atlantic Coastal Plain our results help to constrain the contribution of these areas to global carbon burial.
Van Helmond et al. (2014) point out that TOC is lower in the Bass River core than other OAE2 sections in the North Atlantic region, but our results indicate that Bass River is about average for inner continental shelf deposits (Fig. 8). Average TOC during OAE2 at Bass River is 1.1 wt % (van Helmond et al., 2014); this is slightly higher than Smith Elementary (0.83 wt %) and Hope Plantation (0.63 wt %) and slightly lower than the next closest published shelf site to the southwest, the Sun Spinks core in Mississippi (1.4 wt %; Lowery et al., 2017). Sequence stratigraphic analysis of Cenomanian–Turonian sediments of the Clubhouse and Bass River formations shows that these sediments represent maximum sea level rise across the boundary on the Atlantic Coastal Plain (Miller et al., 2004; Aleman Gonzalez et al., 2020). The location of the Hope Plantation core (lowest TOC values) higher on the inner paleoshelf relative to Smith Elementary School and Bass River (higher TOC values) suggests that TOC wt % on the shelf during OAE2 was, at least in part, a function of paleodepth. To be sure, these TOC values are certainly lower than values found offshore in the open ocean or along upwelling margins in the eastern proto-North Atlantic. For example, Deep Sea Drilling Project Site 603, on the lower continental rise directly offshore of North Carolina, has an average TOC of 5.4 wt % during OAE2 (Kuypers et al., 2004), while the upwelling-prone region at Tarfaya, Morocco, has an average TOC of 8.0 wt % (Kolonic et al., 2005).
Sedimentation rate also plays an important role in organic carbon accumulation by diluting organic carbon in expanded sections so that TOC is not a reliable indicator and increasing organic carbon preservation potential in clay-rich areas (e.g., Berner, 1980). While we do not have dry bulk density measurements from these cores to calculate mass accumulation rates, we can approximate using reasonable values for organic-rich siliciclastic rocks (2.4 g cm−3, following Owens et al., 2018). We can determine the average sedimentation rate during the event using the observed thickness of the OAE2 carbon isotope excursion in each core and the orbitally tuned duration of OAE2 at the Global Stratotype Section and Point in Pueblo, CO (Sageman et al., 2006; Meyers et al., 2012), of 540 kyr. A constant sedimentation rate on the shelf during OAE2 is almost certainly an oversimplification, but it is sufficient for our purpose of comparing general trends between these cores. Using these values we find that organic carbon mass accumulation rates (OC MAR) during OAE2 average 0.05 g cm−2 kyr−1 at Hope Plantation, 0.04 g cm−2 kyr−1 at Smith Elementary School, 0.06 g cm−2 kyr−1 at Bass River, and 0.11 g cm−2 kyr−1 at Spinks. For comparison, the same method indicates OC MAR rates of 0.29 g cm−2 kyr−1 at DSDP Site 603 and 2.84 g cm−2 kyr−1 at Tarfaya (Owens et al., 2018). Owens et al. (2018) found an average OC MAR on shelf sites during OAE2 of 0.11 g cm−2 kyr−1, which means the inner shelf sites on the east coast of North America are below the global average during this event.
These data suggest a relationship with depth on the shelf and TOC deposition during OAE2. If we arrange the sites by depth (Fig. 8) we see the lowest average TOC values at Hope Plantation (0.63 wt %), the most proximal site; values are slightly higher at Smith Elementary (0.83 wt %), which appears to represent an outer estuary or inner shelf environment, and higher still at Bass River (1.1 wt %), which was inner to middle shelf (Miller et al., 2004). Estimates of organic carbon mass accumulation rates suggest all three of these inner shelf sites are very similar, ranging from 0.4–0.6 g cm−2 kyr−1. Average TOC is even higher in the Spinks core (1.4 wt %, or 0.11 g cm−2 kyr−1), which represents inner to middle shelf depths during the latter part of OAE2 (Lowery et al., 2017). This suggests the possibility of even higher values on more distal parts of the shelf and highlights the need for a true depth transect (as opposed to four cores from three states) to better understand that variability and better constrain organic carbon burial in this important environment during OAEs.
Calcareous nannoplankton biostratigraphy shows that positive carbon isotope excursions in two cores on the Atlantic Coastal Plain in North Carolina are associated with the Cenomanian–Turonian OAE2. C N ratios in both cores indicate an increase in the proportion of land plants delivered to these offshore sites, indicating a strengthened hydrologic cycle and causing increased terrigenous flux beginning slightly before OAE2 and continuing through the whole event. This agrees with palynology-based observations from the Bass River core located ∼ 500 km to the north (van Helmond et al., 2014). We therefore conclude that these changes reflect increased precipitation and weathering across eastern North America during OAE2, feeding nutrients onto the shelf and into the proto-North Atlantic and likely contributing to the widespread black shale deposition in the deep basin. These cores are the second and third records of OAE2, to our knowledge, on the coastal plain of eastern North America, and, combined with the first (Bowman and Bralower, 2005; van Helmond et al., 2014), they show relatively low average TOC values (∼ 0.6–1.1 wt %) on the inner shelf during this event while suggesting a trend of increasing values with depth, highlighting the need for more cores in this region from middle and outer shelf depths.
Total organic carbon, total nitrogen, organic carbon isotope, geophysical, and calcareous nannofossil occurrence data are available for download as a USGS Data Release at https://doi.org/10.5066/P9V0U1NF (Self-Trail et al., 2021).
CML and JMST conceived of the study and sampled the cores. JMST sat the wells in 2004 and 2005 and helped describe the cores. CDB conducted bulk organic carbon nitrogen and organic carbon isotope measurements. JMST conducted calcareous nannoplankton biostratigraphy. CML supervised foraminifer analysis. CML prepared the paper with contributions from JMST and CDB.
The authors declare that they have no conflict of interest.
Any use of trade, firm, or product names is for descriptive purposes only and does not imply endorsement by the US Government.
We would like to gratefully acknowledge the editor, Appy Sluijs, and two anonymous reviewers whose constructive feedback significantly improved this work. We are grateful to the drillers and personnel of the USGS for taking these cores, to the staff of the North Carolina Geological Survey for maintaining the cores and making them accessible for sampling, to Lara Yagodzinski for her help sampling the cores, to Kate Gilbreath for her help preparing samples for micropaleontological analysis, and to Ellen Seefelt for her assistance preparing samples for nannofossil analysis and for shipping material. Core box photographs are courtesy of the USGS and NCGS. We acknowledge the people, up to 200 at a time, who were held as slaves at Hope Plantation between 1748 and 1865.
This paper was edited by Appy Sluijs and reviewed by two anonymous referees.
Aleman Gonzalez, W. B., Self-Trail, J. M., Harris, W. B., Moore, J. P., and Farrell, K. M.: Depositional sequence stratigraphy of Turonian to Santonian sediments, Cape Fear arch, North Carolina Coastal Plain, USA, Stratigraphy, 17, 293–314, 2020.
Applin, P. L. and Applin, E. R.: Regional subsurface stratigraphy and structure of Florida and southern Georgia, Am. Assoc. Petr. Geol. B., 28, 1673–1753, 1944.
Applin, P. L. and Applin, E. R.: Regional subsurface stratigraphy, structure, and correlation of middle and early Upper Cretaceous rocks in Alabama, Georgia, and north Florida, U.S. Geological Survey Oil and Gas Investigations Chart, OC-26, 3 sheets, United States Government Printing Office, Washington, DC, USA, 1947.
Applin, P. L. and Applin, E. R.: The Gulf Series in the subsurface in northern Florida and southern Georgia, U.S. Geological Survey Professional Paper 524-G, United States Government Printing Office, Washington, DC, USA, 40 pp., 1967.
Arthur, M. A., Schlanger, S. T., and Jenkyns, H. C.: The Cenomanian–Turonian Oceanic Anoxic Event, II. Palaeoceanographic controls on organic-matter production and preservation, Geological Society, London, Special Publications, 26, 401–420, 1987.
Berner, R. A.: Early Diagenesis: A Theoretical Approach, Princeton University Press, Princeton, NJ, USA, 241 pp., 1980.
Blättler, C. L., Jenkyns, H. C., Reynard, L. M., and Henderson, G. M.: Significant increases in global weathering during Oceanic Anoxic Events 1a and 2 indicated by calcium isotopes, Earth Planet. Sc. Lett., 309, 77–88, 2011.
Bowman, A. R. and Bralower, T. J.: Paleoceanographic significance of high-resolution carbon isotope records across the Cenomanian–Turonian boundary in the Western Interior and New Jersey coastal plain, USA, Mar. Geol., 217, 305–321, 2005.
Bown, P. R. and Young, J. R.: Techniques, in: Calcareous Nannofossil Biostratigraphy, edited by: Bown, P. R., Kluwer Academic, London, UK, 16–28, 1998.
Burdige, D. J.: Preservation of organic matter in marine sediments: controls, mechanisms, and an imbalance in sediment organic carbon budgets?, Chem. Rev., 107, 467–485, 2007.
Burnett, J. A.: Upper Cretaceous, in: Calcareous Nannofossil Biostratigraphy, edited by: Bown, P. R., Kluwer Academic Publishing, Dordrecht, the Netherlands, 132–199, 1998.
Caron, M., Dall'Agnolo, S., Accarie, H., Barrera, E., Kauffman, E. G., Amédro, F., and Robaszynski, F.: High-resolution stratigraphy of the Cenomanian–Turonian boundary interval at Pueblo (USA) and wadi Bahloul (Tunisia): stable isotope and bio-events correlation, Geobios, 39, 171–200, 2006.
Cooke, C. W.: Geology of the Coastal Plain of South Carolina, U.S. Geological Survey Bulletin 867, United States Government Printing Office, Washington, DC, USA, 196 pp., 1936.
Corbett, M. J., Watkins, D. K., and Pospichal, J. J.: A quantitative analysis of calcareous nannofossil bioevents of the Late Cretaceous (Late Cenomanian-Coniacian) Western Interior Seaway and their reliability in established zonation schemes, Mar. Micropaleontol., 109, 30–45, 2014.
Dorf, E.: Critical analysis of Cretaceous stratigraphy and paleobotany of Atlantic Coastal Plain, AAPG Bull., 36, 2161–2184, 1952.
Erbacher, J., Friedrich, O., Wilson, P. A., Birch, H., and Mutterlose, J.L Stable organic carbon isotope stratigraphy across Oceanic Anoxic Event 2 of Demerara Rise, western tropical Atlantic, Geochem. Geophy. Geosy., 6, Q06010, https://doi.org/10.1029/2004GC000850, 2005.
Friedrich, O., Norris, R. D., and Erbacher, J.: Evolution of middle to Late Cretaceous oceans – a 55 my record of Earth's temperature and carbon cycle, Geology, 40, 107–110, 2012.
Gale, A.: Correlation, age and significance of Turonian Chalk hardgrounds in southern England and northern France: The roles of tectonics, eustasy, erosion and condensation, Cretaceous Res., 103, 104164, https://doi.org/10.1016/J.CRETRES.2019.06.010, 2019.
Gale, A. S. and Christensen, W. K.: Occurrence of the belemnite Actinocamax plenus in the Cenomanian of SE France and its significance, B. Geol. Soc. Denmark, 43, 68–77, 1996.
Gohn, G. S.: Revised nomenclature, definitions, and correlations for the Cretaceous formations in USGS-Clubhouse Crossroads #1, Dorchester County, South Carolina, U.S. Geological Survey Professional Paper 1518, , United States Government Printing Office, Washington, DC, USA, 39 pp. 1992.
Gohn, G. S.: Ostracode biostratigraphy of the Upper Cretaceous marine sediments in the New Jersey Coastal Plain, in: Contributions to the paleontology of New Jersey. Geological Association of New Jersey Annual Field Conference, 12th Annual Meeting, 27–28 October 1995, Wayne, NJ, USA, edited by: Baker, J. E. B., 12, 87–101, 1995.
Hasegawa, T., Crampton, J. S., Schiøler, P., Field, B., Fukushi, K., and Kakizaki, Y. Carbon isotope stratigraphy and depositional oxia through Cenomanian/Turonian boundary sequences (Upper Cretaceous) in New Zealand, Cretaceous Res., 40, 61–80, 2013.
Hattner, J. G. and Wise Jr., S. W.: Upper Cretaceous calcareous nannofossil biostratigraphy of South Carolina, South Carolina Geology, 24, 41–117, 1980.
Hazel, J. E., Bybell, L. M., Christopher, R. A., Frederiksen, N. O., May, F. E., McLean, D. M., Poore, R. Z., Smith, C. C., Sohl, N. F., Valentine, P. C., and Witmer, R. J.: Biostratigraphy of the deep corehole (Clubhouse Crossroads corehole 1) near Charleston, South Carolina, in: Studies related to the Charleston, South Carolina earthquake of 1886 – A preliminary report, edited by: Rankin, D. W., U.S. Geological Survey Professional Paper 1028, , United States Government Printing Office, Washington, DC, USA, 71–89, 1977.
Heron Jr., S. D.: History of terminology and correlations of the basal Cretaceous formations of the Carolinas, South Carolina Division of Geology Bulletin, 2, 77–88, 1958.
Jarvis, I., Lignum, J. S., Gröcke, D. R., Jenkyns, H. C., and Pearce, M. A.: Black shale deposition, atmospheric CO2 drawdown, and cooling during the Cenomanian-Turonian Oceanic Anoxic Event, Paleoceanography, 26, PA3201, https://doi.org/10.1029/2010PA002081, 2011.
Jenkyns, H. C.: Geochemistry of oceanic anoxic events, Geochem. Geophy. Geosy., 11, Q03004, https://doi.org/10.1029/2009GC002788, 2010.
Kennedy, W. J., Walaszczyk, I., and Cobban, W. A.: The global boundary stratotype section and point for the base of the Turonian stage of the Cretaceous: Pueblo, Colorado, USA, Episodes, 28, 93–104, 2005.
Kolonic, S., Wagner, T., Forster, A., Sinninghe Damsté, J. S., Walsworth-Bell, B., Erba, E., Turgeon, S., Brumsack, H. J., Chellai, E. H., Tsikos, H., and Kuhnt, W.: Black shale deposition on the northwest African Shelf during the Cenomanian/Turonian oceanic anoxic event: Climate coupling and global organic carbon burial, Paleoceanography, 20, PA1006, https://doi.org/10.1029/2003PA000950, 2005.
Kuypers, M. M., Lourens, L. J., Rijpstra, W. I. C., Pancost, R. D., Nijenhuis, I. A., and Damsté, J. S. S.: Orbital forcing of organic carbon burial in the proto-North Atlantic during oceanic anoxic event 2, Earth Planet. Sci. Lett., 228, 465–482, 2004.
Leckie, R. M., Yuretich, R. F., West, O. L., Finkelstein, D., and Schmidt, M.: Paleoceanography of the southwestern Western Interior Sea during the time of the Cenomanian–Turonian boundary (Late Cretaceous), in: Stratigraphy and Paleoenvironments of the Cretaceous Western Interior Seaway, USA, edited by: Dean, W. D. and Arthur, M. A., SEPM Concepts in Sedimentology and Stratigraphy no. 6, Tulsa, OK, USA, 101–126, 1998.
Leckie, R. M., Bralower, T. J., and Cashman, R.: Oceanic anoxic events and plankton evolution: Biotic response to tectonic forcing during the mid-Cretaceous, Paleoceanography, 17, 13-1–13-29, https://doi.org/10.1029/2001PA000623, 2002.
Lowery, C. M., Corbett, M. J., Leckie, R. M., Watkins, D., Romero, A. M., and Pramudito, A.: Foraminiferal and nannofossil paleoecology and paleoceanography of the Cenomanian–Turonian Eagle Ford Shale of southern Texas, Palaeogeogr. Palaeocl., 413, 49–65, 2014.
Lowery, C. M., Cunningham, R., Barrie, C. D., Bralower, T., and Snedden, J. W.: The northern Gulf of Mexico during OAE2 and the relationship between water depth and black shale development, Paleoceanography, 32, 1316–1335, 2017.
McAnena, A., Flögel, S., Hofmann, P. Herrle, J. O., Griesand, A., Pross, J., Talbot, H. M., Rethemeyer, J., Wallmann, K., and Wagner, T.: Atlantic cooling associated with a marine biotic crisis during the mid-Cretaceous period, Nat. Geosci., 6, 558–561, 2013.
Meyers, P. A.: Preservation of elemental and isotopic source identification of sedimentary organic matter, Chem. Geol., 114, 289–302, 1994.
Meyers, P. A.: Organic geochemical proxies of paleoceanographic, paleolimnologic, and paleoclimatic processes, Org. Geochem., 27, 213–250, 1997.
Meyers, P. A.: Applications of organic geochemistry to paleolimnological reconstructions: a summary of examples from the Laurentian Great Lakes, Org. Geochem., 34, 261–289, 2003.
Meyers, S. R., Siewert, S. E., Singer, B. S., Sageman, B. B., Condon, D. J., Obradovich, J. D., Jicha, B.R., and Sawyer, D. A.: Intercalibration of radioisotopic and astrochronologic time scales for the Cenomanian–Turonian boundary interval, Western Interior Basin, USA, Geology, 40, 7–10, 2012.
Miller, K. G. Sugarman, P. J., Browning, J. V., Olsson, R. K., Pekar, S. F., Reilly, T. J., Cramer, B. S., Aubry, M.-P., Lawrence, R. P., Curran, J., Stewart, M., Metzger, J. M., Uptegrove, J., Bukry, D., Burckle, L. H., Wright, J. D., Feigenson, M. D., Brenner, G. J., and Dalton, R. F.: Bass River site, in: Proc. ODP, Init. Repts., edited by: Miller, K. G., Sugarman, P. J., Browning, J. V., Aubry, M.-P., Brenner, G. J., Dalton, R. F., Feigenson, M. D., Lawrence, R. P., Metzger, J. M., Olsson, R. K., Pekar, S. F., Reilly, T. J., Stewart, M., Uptegrove, J., and Wright, J. D., 174AX: College Station, TX (Ocean Drilling Program), 5–43, https://doi.org/10.2973/odp.proc.ir.174ax.1998, 1998.
Miller, K. G., Sugarman, P. J., Browning, J. V., Kominz, M. A., Olsson, R. K., Feigenson, M. D., and Hernandez, J. C.: Upper Cretaceous sequences and sea-level history, New Jersey Coastal Plain, GSA Bulletin, 116, 368–393, 2004.
Monteiro, F. M., Pancost, R. D., Ridgwell, A., and Donnadieu, Y.: Nutrients as the dominant control on the spread of anoxia and euxinia across the Cenomanian-Turonian oceanic anoxic event (OAE2): Model-data comparison, Paleoceanography, 27, PA4209, https://doi.org/10.1029/2012PA002351, 2012.
O'Connor, L. K., Jenkyns, H. C., Robinson, S. A., Remmelzwaal, S. R., Batenburg, S. J., Parkinson, I. J., and Gale, A. S.: A Re-evaluation of the Plenus Cold Event, and the Links Between CO2, Temperature, and Seawater Chemistry During OAE 2, Paleoceanogr. Paleocl., 35, e2019PA003631, https://doi.org/10.1029/2019PA003631, 2020.
Ostrander, C. M., Owens, J. D., and Nielsen, S. G.: Constraining the rate of oceanic deoxygenation leading up to a Cretaceous Oceanic Anoxic Event (OAE-2: ∼ 94 Ma), Sci. Adv., 3, e1701020, https://doi.org/10.1126/sciadv.1701020, 2017.
Owens, J. D., Lyons, T. W., Hardisty, D. S., Lowery, C. M., Lu, Z., Lee, B., and Jenkyns, H. C.: Patterns of local and global redox variability during the Cenomanian–Turonian Boundary Event (Oceanic Anoxic Event 2) recorded in carbonates and shales from central Italy, Sedimentology, 64, 168–185, 2017.
Owens, J. D., Lyons, T. W., and Lowery, C. M.: Quantifying the missing sink for global organic carbon burial during a Cretaceous oceanic anoxic event, Earth Planet. Sc. Lett., 499, 83–94, 2018.
Petters, S. W.: Upper Cretaceous subsurface stratigraphy of Atlantic Coastal Plain of New Jersey, AAPG Bull. 60, 87–107, 1976.
Petters, S. W.: Upper Cretaceous planktonic foraminifera from the subsurface of the Atlantic Coastal Plain of New Jersey, J. Foramin. Res., 7, 165–187, 1977.
Pogge Von Strandmann, P. A., Jenkyns, H. C., and Woodfine, R. G.: Lithium isotope evidence for enhanced weathering during Oceanic Anoxic Event 2, Nat. Geosci., 6, 668–672, 2013.
Pratt, L. M. and Threlkeld, C. N.: Stratigraphic significance of 13C/12C ratios in mid-Cretaceous rocks of the Western Interior, USA, in: The Mesozoic of Middle North America, edited by: Stott, D. F. and Glass, D. J., Canadian Society of Petroleum Geologists Memoir, 9, 305–312, 1984.
Richards, H. G.: Subsurface stratigraphy of Atlantic Coastal Plain between New Jersey and Georgia, Am. Assoc. Petr. Geol. B., 29, 885–955, 1945.
Sageman, B. B., Meyers, S. R., and Arthur, M. A.: Orbital time scale and new C-isotope record for Cenomanian–Turonian boundary stratotype, Geology, 34, 125–128, 2006.
Schlanger, S. O. and Jenkyns, H. C.: Cretaceous oceanic anoxic events: causes and consequences, Geol. Mijnbouw, 55, 179–184, 1976.
Scholle, P. A. and Arthur, M. A.: Carbon isotope fluctuations in Cretaceous pelagic limestones: potential stratigraphic and petroleum exploration tool, AAPG Bull., 64, 67–87, 1980.
Seefelt, E. L., Self-Trail, J. M., and Schultz, A. P.: Comparison of three preservation techniques for slowing dissolution of calcareous nannofossils in organic-rich sediments, Micropaleontology, 61, 149–164, 2015.
Self-Trail, J. M. and Bybell, L. M.: Cretaceous and Paleogene calcareous nannofossil biostratigraphy of New Jersey, in: Contributions to the paleontology of New Jersey, Geological Association of New Jersey Annual Field Conference, 12th Annual Meeting, 27–28 October 1995, Wayne, NJ, USA, edited by: Baker, J. E. B., 12, 102–139, 1995.
Self-Trail, J. M. and Seefelt, E. L.: Rapid dissolution of calcareous nannofossils: a case study from freshly cored sediments of the south-eastern Atlantic Coastal Plain, Journal of Nannoplankton Research, 27, 149–157, 2005.
Self-Trail, J. M., Barrie, C. D., and Lowery, C. M.: Isotope, organic carbon, and biostratigraphic data for the Hope Plantation (BE-110) and Smith Elementary School (CR-675) cores, North Carolina, USGS Data Release [data set], https://doi.org/10.5066/P9V0U1NF, 2021.
Shamrock, J. L. and Self-Trail, J. M.: Quantification of a pretreatment procedure for organic-rich calcareous nannofossil samples, Journal of Nannoplankton Research, 36, 65–75, 2016.
Shamrock, J. L., Munoz, E. J., and Carter, J. H.: An improved sample preparation technique for calcareous nannofossils in organic-rich mudstones, Journal of Nannoplankton Research, 35, 101–110, 2015.
Sikora, P. J. and Olsson, R. K.: A paleoslope model of late Albian to early Turonian foraminifera of the western Atlantic margin and North Atlantic basin, Mar. Micropaleontol., 18, 25–72, 1991.
Sissinghi, W.: Biostratigraphy of Cretaceous calcareous nanoplankton, Geol. Mijnbouw, 56, 37–65, 1977.
Slattery, J. S., Cobban, W. A., McKinney, K. C., Harries, P. J., and Sandness, A. L.: Early Cretaceous to Paleocene paleogeography of the Western Interior Seaway: the interaction of eustasy and tectonism, Wyoming Geological Association Guidebook, 2015, 22–60, 2015.
Snedden, J. W., Virdell, J., Whiteaker, T. L., and Ganey-Curry, P.: A basin-scale perspective on Cenomanian–Turonian (Cretaceous) depositional systems, greater Gulf of Mexico (USA), Interpretation, 4, SC1–SC22, 2015.
Snow, L. J., Duncan, R. A., and Bralower, T. J.: Trace element abundances in the Rock Canyon Anticline, Pueblo, Colorado, marine sedimentary section and their relationship to Caribbean plateau construction and oxygen anoxic event 2, Paleoceanography, 20, PA3005, https://doi.org/10.1029/2004PA001093, 2005.
Sohl, N. F. and Owens, J. P.: Cretaceous stratigraphy of the Carolina coastal plain, The geology of the Carolinas, Carolina Geological Survey, Winston-Salem, NC, USA, 191–220, 1991.
Spangler, W. B.: Subsurface geology of Atlantic coastal plain of North Carolina, AAPG Bull., 34, 100–132, 1950.
Stephenson, L. W.: The Cretaceous formations, in: The Coastal Plain of North Carolina, edited by: Clark, W. B., Miller, B. L., Stephenson, L. W., Johnson, B. L., and Parker, H. N., North Carolina Geologic and Economic Survey, 3, 73–171, 1912.
Tarduno, J. A., Sliter, W. V., Kroenke, L., Leckie, M., Mayer, H., Mahoney, J. J., Musgrave, R., Sotery, M., and Winterer, E. L.: Rapid formation of Ontong Java Plateau by Aptian mantle plume volcanism, Science, 254, 399–403, 1991.
Thornburg, J.: “Temporal variations in the paleopedology and paleoclimatology of the Early Cretaceous Potomac Group, Hope Plantation core, Bertie County, North Carolina”, unpublished Masters Thesis, Temple University, Philadelphia, PA, USA, 2008.
Tibert, N. E. and Leckie, R. M.: High-resolution estuarine sea level cycles from the Late Cretaceous: Amplitude constraints using agglutinated foraminifera, J. Foramin. Res., 34, 130–143, 2004.
Tsikos, H., Jenkyns, H. C., Walsworth-Bell, B., Petrizzo, M. R., Forster, A., Kolonic, S., Erba, E., Premoli Silva, I., Baas, M., Wagner, T., and Damsté, J. S.: Carbon-isotope stratigraphy recorded by the Cenomanian–Turonian Oceanic Anoxic Event: correlation and implications based on three key localities, J. Geol. Soc., 161, 711–719, 2004.
Turgeon, S. C. and Creaser, R. A.: Cretaceous oceanic anoxic event 2 triggered by a massive magmatic episode, Nature, 454, 323–326, 2008.
Valentine, P. C.: Upper Cretaceous subsurface stratigraphy and structure of coastal Georgia and South Carolina, U.S. Geological Survey Professional Paper 1222, United States Government Printing Office, Washington, DC, USA, 33 pp., 1982.
Valentine, P. C.: Turonian (Eaglefordian) stratigraphy of the Atlantic Coastal Plain and Texas, U.S. Geological Survey Professional Paper 1315, United States Government Printing Office, Washington, DC, USA, 21 pp., 1984.
van Helmond, N. A., Sluijs, A., Reichart, G. J., Damsté, J. S. S., Slomp, C. P., and Brinkhuis, H.: A perturbed hydrological cycle during Oceanic Anoxic Event 2, Geology, 42, 123–126, 2014.
van Helmond, N. A. G. M., Sluijs, A., Sinninghe Damsté, J. S., Reichart, G.-J., Voigt, S., Erbacher, J., Pross, J., and Brinkhuis, H.: Freshwater discharge controlled deposition of Cenomanian–Turonian black shales on the NW European epicontinental shelf (Wunstorf, northern Germany), Clim. Past, 11, 495–508, https://doi.org/10.5194/cp-11-495-2015, 2015.
Weems, R. E., Seefelt, E. L., Wrege, B. M., Self-Trail, J. M., Prowell, D. C., Durand, C., Cobbs, E. F., and McKinney, K. C.: Preliminary physical stratigraphy and geophysical data of the USGS Hope Plantation Core (BE-110), Bertie County, North Carolina, US Geological Survey Open-File Report, 1251, United States Geological Survey, Reston, VA, USA, 1–163, 2007.
Weems, R. E., Self-Trail, J. M., and Edwards, L. E.: Cross Section of the North Carolina Coastal Plain from Enfield through Cape Hatteras, US Geological Survey Open-File Report, 2019-1145, United States Geological Survey, Reston, VA, USA, 2019.
Whitechurch, H., Montigny, R., Sevigny, J., Storey, M., and Salters, V.: K-Ar and 40Ar-39Ar ages of central Kerguelen Plateau basalts, Proc. Ocean Drill. Program Sci. Results, 120, 71–77, 1992.
Zarra, L.: Sequence stratigraphy and foraminiferal biostratigraphy for selected wells in the Albemarle Embayment, North Carolina, North Carolina Geological Survey Open File Report 89-5, United States Government Printing Office, Washington, DC, USA, 52 pp., 1989.