the Creative Commons Attribution 4.0 License.
the Creative Commons Attribution 4.0 License.
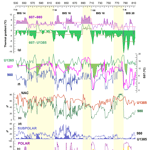
Change in the North Atlantic circulation associated with the mid-Pleistocene transition
Gloria M. Martin-Garcia
Francisco J. Sierro
José A. Flores
Fátima Abrantes
The southwestern Iberian margin is highly sensitive to changes in the distribution of North Atlantic currents and to the position of oceanic fronts. In this work, the evolution of oceanographic parameters from 812 to 530 ka (MIS20–MIS14) is studied based on the analysis of planktonic foraminifer assemblages from site IODP-U1385 (37∘34.285′ N, 10∘7.562′ W; 2585 m b.s.l.). By comparing the obtained results with published records from other North Atlantic sites between 41 and 55∘ N, basin-wide paleoceanographic conditions are reconstructed. Variations of assemblages dwelling in different water masses indicate a major change in the general North Atlantic circulation during MIS16, coinciding with the definite establishment of the 100 ky cyclicity associated with the mid-Pleistocene transition. At the surface, this change consisted in the redistribution of water masses, with the subsequent thermal variation, and occurred linked to the northwestward migration of the Arctic Front (AF), and the increase in the North Atlantic Deep Water (NADW) formation with respect to previous glacials. During glacials prior to MIS16, the NADW formation was very weak, which drastically slowed down the surface circulation; the AF was at a southerly position and the North Atlantic Current (NAC) diverted southeastwards, developing steep south–north, and east–west, thermal gradients and blocking the arrival of warm water, with associated moisture, to high latitudes. During MIS16, the increase in the meridional overturning circulation, in combination with the northwestward AF shift, allowed the arrival of the NAC to subpolar latitudes, multiplying the moisture availability for ice-sheet growth, which could have worked as a positive feedback to prolong the glacials towards 100 ky cycles.
- Article
(3812 KB) - Full-text XML
- BibTeX
- EndNote
Climate in the North Atlantic region is characterized by the continuous poleward heat flow carried out by the oceanic circulation. The Gulf Stream and the North Atlantic Current (NAC) transport warm and salty surface water, originating in the tropical region, towards the polar ocean, the northeast Atlantic, and along the western European margin, transferring heat and moisture to the atmosphere during the process (e.g. McCartney and Talley, 1984; Ruddiman and McIntyre, 1984; Schmitz and McCartney, 1993; Rahmstorf, 1994; Chapman and Maslin, 1999). Surface circulation and associated heat flow is pumped by the sinking of surface water in the subpolar region and formation of the North Atlantic Deep Water (NADW). As a matter of fact, the Atlantic meridional overturning circulation (AMOC) is responsible for ∼50 % of the total poleward heat advection (Sabine et al., 2004; Adkins, 2013).
The NAC forms the transition zone between the cold and productive waters located north of the Arctic Front (AF) (e.g. Johannessen et al., 1994), and the warm and oligotrophic waters from the subtropical gyre in the south. Each water mass has distinct physical–chemical characteristics and specific planktonic foraminiferal assemblages (e.g. Bé, 1977; Ottens, 1991; Cayre et al., 1999). Various studies have shown that surface water characteristics in the midlatitude North Atlantic depend on the strength and position of the NAC and associated oceanic fronts (Calvo et al., 2001; Naafs et al., 2010; Voelker et al., 2010). During Pleistocene glacials, the AF migrated southward into the midlatitude North Atlantic (Stein et al., 2009; Villanueva et al., 2001), cold polar waters expanded to lower latitudes, and the NAC did not reach as far north as during interglacials (e.g. Pflaumann et al., 2003).
After MIS21, a northwestward shift in the position of the AF began (Hernandez-Almeida et al., 2013), that culminated at the end of MIS16, in a similar location to that of the present day (Wright and Flower, 2002). Coinciding with the final stage of this shift, a major reorganization of the meridional overturning circulation developed, related to increased NADW formation that resulted in deeper and southward penetration of this mass of water (Poirier and Billups, 2014). Both processes could have been related to the prolongation of glacials that occurred at the end of the mid-Pleistocene transition (MPT). This was the transitional period during which the Earth's climate system underwent a major change, non-linear 100 ky cycles appeared and superimposed over the more linear, orbital ones of 41 and 23 ky.
Although there is still no agreement over the initiation of the MPT (e.g. Clark et al., 2006; Maslin and Brierley, 2015), strong 100 ky cycles have been recorded since ∼650 ka (Ruddiman et al., 1989; Imbrie et al., 1993; Mudelsee and Schulz, 1997). Related to the shift in the AF position, warm and salty surface water could reach subpolar latitudes during glacials, which would have provided the necessary humidity to prolong the growth of ice sheets, as well as enhanced meridional overturning – both processes acting as feedback mechanisms partly responsible for the change of the climate system phasing (Imbrie et al., 1993). The objective of this work is to study the evolution of glacial circulation in the North Atlantic from MIS20 to MIS14, and explore its possible relation with the MPT.
Over the last glacial cycle, the Iberian margin recorded both peak displacement events of the AF and periods of greater influence of subtropical water from the Azores Current (AzC) (e.g. Martrat et al., 2007; Eynaud et al., 2009; Salgueiro et al., 2010). There is also evidence that polar to tropical planktonic foraminifers' assemblages co-occurred in a latitudinal band around 35–40∘ N during the Last Glacial Maximum (McIntyre et al., 1972), which suggests that the limit between both water masses was situated slightly more southwards than it is today (Fiúza et al., 1998; Peliz et al., 2005). Site IODP-U1385 (37∘34′ N) lies within this oscillating boundary and has been shown an ideal location to study oceanographic changes in the North Atlantic through glacial–interglacial periods (e.g. Maiorano et al., 2015; Martin-Garcia et al., 2015; Rodríguez-Tovar et al., 2015; Rodrigues et al., 2017). Analyses of planktonic foraminifer assemblages are used to identify the different water masses, and results from IODP-U1385 are compared with published data from other North Atlantic latitudes to reach basin-wide conclusions.
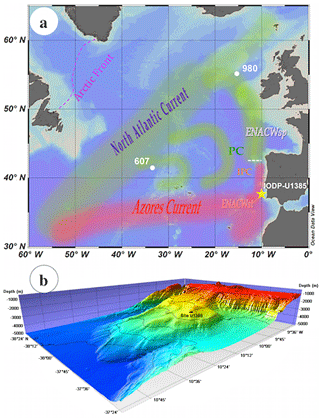
Figure 1(a) Modern surface circulation in the North Atlantic and location of IODP-U1385 and other sites discussed in this paper. ENACWsp: eastern North Atlantic Central Water of subpolar origin; ENACWst: eastern North Atlantic Central Water of subtropical origin; IPC: Iberian Poleward Current; PC: Portugal Current. The white dashed line represents the today's approximate surface limit between ENACWsp and ENACWst (Fiúza et al., 1998). (b) Regional bathymetry of the southwest Iberian margin, showing site U1385 (Expedition 339 Scientists, 2012).
2.1 IODP site U1385
The southwestern Iberian margin is a focal location for paleoclimate and oceanographic research of the Quaternary (Hodell et al., 2013). Site IODP-U1385 was drilled at the so-called Shackleton site (37∘34.284′ N, 10∘7.562′ W), at 2589 m water depth (Fig. 1). At the surface, this area lies under the influence of the North Atlantic Central Water (NACW), with a complex circulation pattern; at depth, the NADW flows between ∼2200 and 4000 m, above the Antarctic Bottom Water (AABW).
Today's surface water circulation in the North Atlantic (Fig. 1a) consists of two different branches. The NAC, after reaching the subpolar ocean, drifts southwards along Europe, transporting the eastern North Atlantic Central Water of subpolar origin (ENACWsp), formed north of 46∘ (Brambilla and Talley, 2008). In the south, the AzC, of subtropical origin (ENACWst) and formed along the Azores Front (Rios et al., 1992), drifts eastwards and bifurcates when approaching the continental margin. The ENACWst is saltier, warmer, less dense than the ENACWsp and overflows it along the Iberian Peninsula with a decreasing lower limit from south to north until ∼42.7∘ N (Fiúza et al., 1998).
Sediments at site U1385 define a single, very uniform, lithological unit. Calcareous muds and calcareous clays dominate the lithology. The relative proportions of carbonate (23 %–39 %) and terrigenous materials are seen in the sediment colour that varies from dark (i.e. more terrigenous) to light (i.e. more calcareous). The average sedimentation rate for the section is of ∼10 cm ky−1 (Stow et al., 2012).
2.2 Foraminiferal study
This study covers a section comprised between 67.2 and 94.6 crmcd (MIS14–MIS20). The age model (Hodell et al., 2015) is based on the correlation of the benthic oxygen isotope record to the global benthic LR04 isotope stack (Lisiecki and Raymo, 2005). For better comparison of our results with data from other North Atlantic sites, new age models were calculated for sites 980 and 607, based on correlations with the LR04 stack.
Sampling was performed every 20 cm, providing a 1.76 ky resolution on average. A total of 147 samples, 1 cm thick, were freeze dried, weighed, and washed over a 63 µm mesh. The > 63 µm residue was dried, weighed, and sieved again to separate and weigh the > 150 µm fraction. Planktonic foraminifers' taxa were identified (Kennett and Srinivasan, 1983) in aliquots of this last fraction containing a minimum of 300 specimens.
The microfaunal analysis focused on species and assemblages that are associated with North Atlantic surface water masses (Appendices A and B). Neogloboquadrina pachyderma sinistral (N. pachyderma sin) is an indicator of polar water (Cayre et al., 1999; Pflaumann et al., 2003; Eynaud et al., 2009). Turborotalita quinqueloba dwells in cold waters and is usually associated with the AF (Johannessen et al., 1994; Cayre et al., 1999). Globigerina bulloides, Globigerinella siphonifera (aequilateralis), Globorrotalia inflata, and Neogloboquadrina incompta (former N. pachyderma dextral) form the NAC assemblage, as defined by Ottens (1991). Finally, species included in the warm surface assemblage (Vautravers et al., 2004) are Beela digitata, Globigerina falconensis, Globigerinella siphonifera (aequilateralis), Globigerinoides ruber, Globigerinoides sacculifer, Globoturborotalita rubescens, Globoturborotalita tenella, Orbulina universa, and Pulleniatina obliquiloculata.
2.3 Estimation of thermal gradients
Thermal gradients in the North Atlantic are reconstructed by calculating the difference between the sea surface temperature (SST) from two sites. The site 607 was used as the starting point and compared with sites 980 for the latitudinal gradient (SST607 – SST980) and U1385 for the longitudinal one (SST607 – SSTU1385). In this way, a positive longitudinal gradient means that SST was warmer at site 607 than at U1385; a negative longitudinal gradient indicates warmer SST off the southwest Iberian Peninsula than at site 607.
This estimation of thermal gradients is possible because all the SST records used for this work are based on planktonic foraminifers' census counts. Nevertheless, prior to the comparison, interpolation was applied to obtain records with the same age points.
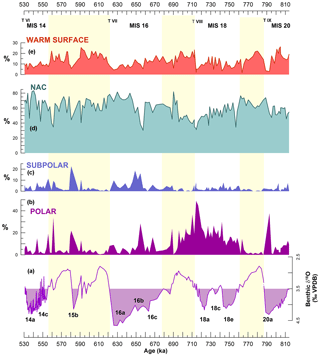
Figure 2Relative abundance of planktonic foraminiferal species and assemblages in IODP-U1385 through MIS14–MIS20, and comparison with benthic isotope data from the same site. (a) Benthic δ18O record (Hodell et al., 2015) with filling enhancing glacial conditions according to the threshold for the North Atlantic (McManus et al., 1999); glacial substages are named according to Railsback et al. (2015). Relative abundance of (b) polar species N. pachyderma sinistral; (c) subpolar species T. quinqueloba; (d) NAC assemblage (as defined by Ottens, 1991); and (e) warm surface assemblage (as defined by Vautravers et al., 2004). Yellow bands highlight interglacials. Terminations (T) are marked in Roman numerals. IODP-U1385 isotopic record is from Hodell et al. (2015).
Except in the eighth climate cycle (MIS19–MIS18), Neogloboquadrina pachyderma sinistral does not vary at glacial–interglacial scale, but peak percentages are associated either with glacial maxima (MIS20) or with deglaciations, both terminations and other deglacial events (Fig. 2b), revealing increased advection of polar water at these times. N. pachyderma sin is less abundant during interglacial conditions than during glacials, but it is important to note that its percentage during glacials changes through the time series. This species is more abundant during glacials MIS20, MIS18 (when the highest percentages occurred), and the first half of MIS16, than during late MIS16 and glacial MIS14 (Fig. 2b). After ∼650 ka, N. pachyderma sin stays below 10 %, except during deglacial events MIS15b/a and at the end of MIS15, as inferred from sharp decreases in δ18O (Fig. 2a–b). This suggests that, since mid-MIS16, the polar water has only reached the southwest Iberian margin associated with some deglacial episodes, and not during full glacial conditions or glacial maxima, in opposition to what happened before ∼650 ka.
Turborotalita quinqueloba shows lower percentage during MIS20 and MIS18, than since MIS16 (Fig. 2c). Highest values occur at ∼650 ka and during MIS15b, the glacial interval that interrupted interglacial MIS15. The variation of T. quinqueloba at site U1385 does not show an interglacial–glacial pattern, which suggests this site did not register the migration of the AF through each climate cycle.
The NAC assemblage (Ottens, 1991) is the most abundant one at this site (Fig. 2), indicating that the ENACWsp dominates the surface oceanography in the area through the time series. This assemblage does not keep a similar interglacial–glacial pattern through the whole study interval but changes its behaviour at ∼650 ka. Prior to ∼650 ka, its variation mirrors that of N. pachyderma sin, and the highest values occur during interglacials. In opposition to this, since ∼650 ka, the highest percentages have coincided with full glacial conditions (MIS16a and MIS14a), not with interglacials (Fig. 2d).
The warm surface (WS) assemblage (Vautravers et al., 2004) is typical of the subtropical water transported eastwards by the AzC. In U1385, this assemblage has shown a clear interglacial–glacial pattern only since Termination TVIII, its percentage decreasing gradually during MIS17–MIS16 until the glacial maximum (Fig. 2e). Comparing glacial stages, MIS20 records the highest average relative abundance (16.8 %) and MIS14 the lowest (8.7 %). Termination TIX records the most abrupt decrease of this assemblage (15 % drop), while at TVI it even increases (5 % rise). At the beginning of each interglacial, the percentage of this assemblage rises rapidly, suggesting that the AzC strengthens rapidly in the area after terminations.
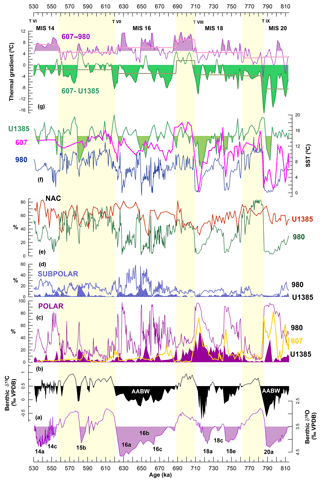
Figure 3Comparison of records from the midlatitude (IODP-U1385; ODP-607) and the subpolar (ODP-980) North Atlantic. Benthic δ18O (a) and δ13C (b) from U1385 (Hodell et al., 2015); filling in panel (b) is enhancing 13C-depleted values typical for Antarctic Bottom Water (AABW) (Adkins et al., 2005). (c) Percentage of N. pachyderma sinistral at sites U1385 (filled), 607 (glod), and 980 (purple). (d) Relative abundance of T. quinqueloba for sites U1385 (filled) and 980. (e) Relative abundance of the NAC assemblage (as defined by Ottens, 1991) at sites U1385 (red) and 980 (green). Site 980 faunal data are from Wright and Flower (2002); for this work, the NAC assemblage of site 980 has been calculated using the published census counts. (f) SST from sites 980 (dark blue; Wright and Flower, 2002), 607 (pink; Ruddiman et al., 1989), and U1385 (green; Martin-Garcia et al., 2015), with filling enhancing lower temperatures than 14.6 ∘C, the average SST for the study interval. (g) Longitudinal (green) and latitudinal (purple) thermal gradients, with the statistical mean for each MIS represented in superimposed straight lines. Age models for sites 980 and 607 have been recalculated using the LR04 stock. Yellow bands highlight interglacials. Terminations (T) are marked in Roman numerals.
The location of sites 607 and 980 along the main core of the NAC towards the high latitudes of the North Atlantic (Fig. 1a) allowed us to monitor past changes in the northward heat transport, using planktonic foraminifer assemblages and SST reconstructions from both sites. By contrast, planktonic foraminifer assemblages at site U1385 are more influenced by the advection of heat to the northeastern Atlantic through the easternmost branches of the NAC, and especially by the AzC, that originates in the tropics and flows towards the Iberian Peninsula following the northern margin of the subtropical gyre (Fig. 1a). In consequence, with these three strategic sites, we can monitor changes in the main circulation systems of the northeast Atlantic during the mid-Pleistocene and estimate the heat advection to the north (SST gradient between sites 607 and 980) and to the northeast Atlantic (SST gradient between sites 607 and U1385) (Fig. 3f–g).
4.1 North Atlantic circulation during glacials MIS20 and MIS18
During both glacials, progressive cooling is recorded at sites 607 and 980 (Fig. 3f). Though the cooling is more pronounced at the higher latitude, the SST gradient between both sites is not very high and decreases largely towards the end of glacial stages (Fig. 3g). In contrast, the Iberian margin remained relatively warm during most of MIS20 and a large part of MIS18 (Fig. 3f), which undoubtedly reflects a continuous flow of the AzC to this region, as also indicated by the WS assemblage record (Fig. 2e).
At the subpolar latitude of site 980, the presence of polar water has increased rapidly since glacial inceptions, as informed by very high percentages of N. pachyderma sin during MIS20, MIS18e, and MIS18a (Fig. 3c). As glacial conditions progressed, the heat flow along the main core of the NAC reduced largely and was even interrupted at glacial maxima MIS20a and MIS18a, as can be inferred from the low temperatures registered in the Azores region (site 607, Fig. 3f). This reduced advection of warm water from the tropics to subpolar latitudes triggered the southward migration of the AF, that surpassed 50∘ N during MIS20, MIS18e, and MIS18a (Wright and Flower, 2002), and favoured the advection of polar water as far south as site 607, as informed by the record of N. pachyderma sin (Fig. 3c).
While the northward flow of heat decreased progressively along both glacials, the heat flow towards the Iberian margin continued in the early part of glacial MIS18 and, especially, during MIS20, indicating a very active AzC during both glacials. This current advected warm water eastward and deflected northward along the Iberian margin, similarly to today's IPC (Fig. 1a), probably overflowing the polar water mass, as the co-occurrence of polar and subtropical fauna suggests (Fig. 2b, e). The advection of the warm AzC to site U1385 was only interrupted at Terminations TIX and TVIII, and at deglaciation MIS18e/d, when massive surges of very cold and low-salinity surface waters reached the area, which was registered by peaks of the polar species N. pachyderma sin and sharp decreases in the WS assemblage (Fig. 2b, e). This interpretation is corroborated by the negative longitudinal thermal gradient between sites 607 and U1385 (Fig. 3g), which indicates that an important fraction of the heat reaching the Iberian margin did not flow through the site 607 region.
The very low SST at the midlatitude site 607 and the low latitudinal thermal gradient during glacial maxima MIS20a, MIS18e, and MIS18a (Fig. 3f–g) suggest either a complete shutdown of the NAC core flux or a southward or southeastward diversion of this current as glacial conditions progressed. Nevertheless, the low thermal gradient between sites 607 and U1385 (Fig. 3g) implies that the southwest Iberian margin was always under the influence of the warmer AzC.
4.2 Changes in the North Atlantic circulation starting at MIS17
Both latitudinal and longitudinal thermal gradients (Fig. 3g) inform of drastic rearrangement of North Atlantic circulation starting at MIS17. SST at site 607 was much warmer than during MIS19, although both interglacials were similar, according to δ18O (Fig. 3a, f). This points to a reactivation of the NAC during MIS17 and a displacement of the current westward site (607). Such reactivation would be the result of increased NADW formation that reached higher rates than during the previous interglacial, as suggested by the ∼0.2 ‰ higher δ13C in MIS17 than in MIS19 (Fig. 3b). On the other hand, the very high latitudinal thermal gradient (Fig. 3g) suggests that this current did not reach subpolar latitudes, as it did during the following interglacial, MIS15, when this gradient was much lower.
The unusually high longitudinal thermal gradient registered during MIS17 was due to the prolonged deglaciation of MIS18 that continuously advected polar water along the Iberian margin (Martin-Garcia et al., 2015), resulting in very cold SST and high percentages of N. pachyderma sin at site U1385 (Fig. 3).
MIS16 was a very prolonged glacial with extensive ice sheets; nevertheless, polar waters did not extend to the midlatitude ocean, as suggested by the low percentages of N. pachyderma sin at sites 607 and U1385 (Fig. 3c).
The latitudinal thermal gradient for most of MIS16, and the whole MIS14, was notably higher than during MIS20–MIS18 (Fig. 3g). This great SST decrease, between sites 607 and 980, must be the result of a significant heat loss to the atmosphere and associated release of water vapour along the path of the NAC during both MIS16 and MIS14. This water vapour release provided the necessary moisture to continue ice-sheet growth, opposite to what had happened during previous glacials. Also contrary to glacials MIS20 and MIS18, when the surface water at the subpolar site 980 progressively cooled towards glacial maxima without important millennial-scale oscillations (Fig. 3f); in glacials MIS16 and MIS14, the surface ocean circulation was very variable and the AF migrated northward–southward to site 980 very frequently (Fig. 3c–d). During short time periods, the NAC reached this subpolar site, conveying heat to the northern-latitude Atlantic (Fig. 3e). However, this oscillation of the AF never affected middle latitudes, according to the fairly mild SST, and low percentage of N. pachyderma sin, recorded both in the open ocean and in the continental margin during MIS16–MIS14 (Fig. 3c, f).
At the midlatitude ocean site 607, SSTs during MIS16 and MIS14 were very different from those recorded in MIS20 and MIS18 (Fig. 3f). While in the older glacials SST decreased towards glacial maxima, this trend is not observed during MIS16 and MIS14, and warm SST was recorded also during glacial maxima.
Although warmer SST were recorded through the midlatitude North Atlantic, a negative thermal gradient still prevailed during MIS16–MIS14 between sites 607 and U1385 (Fig. 3g), indicating a continuous heat flow toward the southwest Iberian Peninsula. This suggests that this region remained under the influence of the subtropical AzC during most parts of glacials MIS16 and MIS14, as it also did during MIS20, based on the mild SST registered at that time (Fig. 3f). Contrary to previous glacials, the NAC remained vigorous at site U1385 during MIS16, except at ∼655 ka, and MIS14, and increased its strength as glacials advanced (Fig. 2d).
4.3 Implications of changes in the North Atlantic circulation associated with the MPT
Assuming a close correlation between the rate of AMOC and benthic δ13C levels (Zahn et al, 1997; Adkins et al., 2005; Hoogakker et al., 2006), we interpret that the published δ13C data from the subpolar North Atlantic (Wright and Flower, 2002; Hodell et al., 2008; Hodell and Channell, 2016) document a long-term increase in the NADW formation rate that was initiated in MIS22 and culminated in MIS14. Since MIS17, midlatitude and subtropical North Atlantic sites registered a progressive increase of NADW at depths previously occupied by the AABW (δ13C data in, e.g. Poirier and Billups, 2014; Martin-Garcia et al., 2015).
The increased production of NADW, during glacials after MIS16 with respect to previous ones, triggered the advection of relatively warm NAC towards subpolar latitude, providing additional humidity to the area and thus enhancing the growth of ice sheets, which led to the prolonged and extreme glaciation of MIS16, one of the first and most prominent glacials of the “100 ky world”. In addition, the intermittent advection of this warm water made ice sheets more vulnerable to internal instabilities, with the subsequent release of icebergs registered in the North Atlantic during MIS16 (e.g. Wright and Flower, 2002; Hodell et al., 2008). The interaction between a more intense AMOC and ice-sheet instabilities, registered by rapid migrations of the AF north and south of site 980 (Fig. 3c–d), resulted in punctual events of sharp reduction of the NADW formation, like that at ∼655 ka that coincided with one of the southernmost positions of the AF, according to the record of T. quinqueloba at site 980 (Wright and Flower, 2002), and was also registered at U1385 by peaks in this species and in N. pachyderma sin, coinciding with very low percentage of NAC assemblage (Fig. 3b–e). Both this episode and the outstanding one ∼650 ka, with the lowest δ13C value since MIS18 in middle latitudes in coincidence with very high abundance of the NAC assemblage in high latitudes (Fig. 3b, e), point to an exceptionally vigorous but shallow NA overturning cell, underlain by significant volumes of southern-sourced water, similarly to the situation at the end of TII (Böhm et al., 2014). This mode of AMOC, according to benthic δ13C records, was maintained during glacial stages MIS16, MIS15b, and MIS14, when the subpolar site 980 recorded > 0.25 ‰ higher δ13C than more southern sites (Wright and Flower, 2002; Martin-Garcia et al., 2015; Hodell et al., 2016).
This vigorous AMOC mode recorded in MIS14 was the culmination of a sequence of increasing deepening of the overturning circulation cell that was initiated in MIS22, and was registered by a tendency towards higher benthic δ13C, both at high and midlatitude sites (U1308 and U1313), from MIS22 to MIS14 (Hodell and Channell, 2016), and was especially noticeable during glacial stages. During MIS20 and MIS18, ice-sheet collapses (Wright and Flower, 2002) produced a continuous flux of meltwater pulses that kept very weak NADW formation, with the deep North Atlantic being occupied by southern-sourced waters, according to very low benthic δ13C recorded both in middle and high latitudes (Wright and Flower, 2002; Hodell and Channell, 2016; Hodell et al., 2015). During these glacials, the almost-shutdown AMOC maintained the AF at a southern position and prevented the northward flux of the necessary moisture for the growth of ice sheets, which could not work as a positive feedback and extend glacial stages over obliquity and precessional (41 and 23 ky) cycles, as they worked during MIS16, one of the first and most prominent glacials of the “100 ky world”.
By studying planktonic foraminiferal assemblages from the Iberian margin (IODP-U1385) for the interval 812–530 ka and comparing them with records from other sites between 41 and 55∘ N, we are able to trace paleoceanographic conditions across the North Atlantic from MIS20 to MIS14 and draw the following conclusions.
Variations of microfaunal assemblages associated with surface currents indicate a major change in the general North Atlantic circulation during this interval, coinciding with the definite establishment of the 100 ky climate phasing. In surface, this change consisted in the redistribution of water masses and associated SST that happened linked to the northwestward migration of the AF during MIS16, and was related to the increasing NADW formation trend that was initiated in MIS22.
Prior to MIS16, the AMOC rate was very low, especially during glacials, the AF was at a southerly position, and the NAC diverted southeastwards, developing steep south–north and east–west thermal gradients, and blockading the arrival of warm water, with associated moisture, to the high-latitude North Atlantic.
During MIS16, the NADW formation increased with respect to previous glacials, especially during glacial maxima, which resulted in the northwestward AF shift and enhanced surface circulation, allowing the arrival of the relatively warm NAC to subpolar latitudes and increasing the moisture availability to continuing the ice-sheet growth, which would have worked as a positive feedback to prolong the duration of glacials to 100 ky cycles.
The available datasets used for this article can be accessed at the NOAA/World Data Service for Paleoclimatology archive (ftp://ftp.ncdc.noaa.gov/pub/data/paleo/contributions_by_author/wright2002/wright2002.txt, Wright and Flower, 2009) and at the PANGAEA data repository (https://doi.org/10.1594/PANGAEA.701229, Ruddiman et al., 1989; https://doi.org/10.1594/PANGAEA.872082, Hodell et al., 2015).
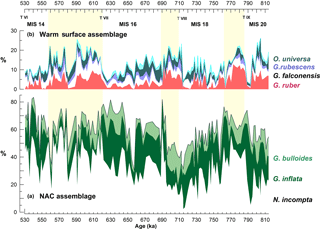
Figure B1Faunal composition of both the NAC and the warm surface assemblages at site U1385 through the study interval. (a) N. incompta (white), G. inflata (dark green), and G. bulloides (light green). (b) G. ruber (red), G. falconensis (white), G. rubescens (lilac), and O. universa (dark green); and in cyan, other species with less than 1.5 % each: G. siphonifera, G. tenella, B. digitata, G. sacculifer, and P. obliquiloculata.
The authors declare that they have no conflict of
interest.
Edited by: Marit-Solveig Seidenkrantz
Reviewed by: two anonymous referees
Adkins, J.: The role of deep ocean circulation in setting glacial climates, Paleoceanography, 28, 539–561, 2013.
Adkins, J. F., Ingersoll, A. P., and Pasquero, C: Rapid climate change and conditional instability of the glacial deep ocean from the thermobaric effect and geothermal heating, Quaternary Sci. Rev., 24, 581–594, 2005.
Bé, A. W. H: Recent planktonic foraminifera, Oceanic Micropaleontology, 1, edited by: Ramsay, A. T. S., Elsevier, New York, 1–100, 1977.
Böhm, E., Lippold, J., Gutjahr, M., Frank, M., Blaser, P., Antz, B., Fohlmeister, J., Frank, N., Andersen, M. B., and Deininger, M: Strong and deep Atlantic meridional overturning circulation during the last glacial cycle, Nature, 517, 73–76, https://doi.org/10.1038/nature14059, 2014.
Brambilla, E. and Talley, L. D: Subpolar Mode Water in the northeastern Atlantic: 1. Averaged properties and mean circulation, J. Geophys. Res., 113, C04025, https://doi.org/10.1029/2006JC004062, 2008.
Calvo, E., Villanueva, J., Grimalt, J. O., Boelaert, A., and Labeyrie, L: New insights into the glacial latitudinal temperature gradients in the North Atlantic. Results from U sea surface temperatures and terrigenous inputs, Earth Planet. Sc. Lett., 188, 509–519, 2001.
Cayre, O., Lancelot, Y., and Vincent, E: Paleoceaanographic reconstructions from planktonic foraminifera off the Iberian Margin: Temperature, salinity and Heinrich events, Paleoceanography, 14, 384–396, 1999.
Chapman, M. R. and Maslin, M. A: Low-latitude forcing of meridional temperature and salinity gradients in the subpolar North Atlantic and the growth of glacial ice sheets, Geology, 27, 875–878, 1999.
Clark, P. U., Archer, D., Pollard, D., Blum, J. D., Rial, J. A., Brovkin, V., Mix, A. C., Pisias, N. G., and Roy, M: The middle Pleistocene transition: characteristics, mechanisms, and implications for long-term changes in atmospheric pCO2, Quaternary Sci. Rev., 25, 3150–3184, 2006.
Expedition 339 Scientists: Mediterranean outflow: environmental significance of the Mediterranean Outflow Water and its global implications, IODP Prel. Rept., 339, https://doi.org/10.2204/iodp.pr.339.2012, 2012.
Eynaud, F., de Abreu, L., Voelker, A., Schonfeld, J., Salguerio, E., Turon, J. L., Penaud, A., Toucanne, S., Naughton, F., and Sanchez-Goñi, M. F: Position of the Polar Front along the western Iberian margin during key cold episodes of the last 45 ka, Geochem. Geophy. Geosy., 10, Q07U05, https://doi.org/10.1029/2009GC002398, 2009.
Fiúza, A. F. G., Hamann, M., Ambar, I., Díaz del Río, G., González, N., and Cabanas, J. M: Water masses and their circulation off western Iberia during May 1993, Deep-Sea Res., 45, 1127–1160, 1998.
Hernandez-Almeida, I., Bjoklund, K. R., Sierro, F. J., Filippelli, G. M., Cacho, I., and Flores, J. A: A high resolution opal and radiolarian record from the subpolar North Atlantic during the Mid-Pleistocene Transition (1069–779 ka): Paleoceanographic implications, Palaeogeogr. Palaeocl., 391, 49–70, https://doi.org/10.1016/j.palaeo.2011.05.049, 2013.
Hodell, D., Crowhurst, S., Skinner, L., Tzedakis, P. C., Margari, V., Channell, J. E. T., Kamenov, G., Maclachlan, S., and Rothwell, G: Response of Iberian Margin sediments to orbital and suborbital forcing over the past 420 ka, Paleoceanography, 28, 185–199, 2013.
Hodell, D., Lourens, L., Crowhurst, S., Konijnendijk, T., Tjallingii, R., and the Shackleton Site Project Members: A reference time scale for Site U1385 (Shackleton Site) on the Iberian Margin, Global Planet. Change, 133, 49–64, 2015.
Hodell, D. A. and Channell, J. E. T.: Mode transitions in Northern Hemisphere glaciation: co-evolution of millennial and orbital variability in Quaternary climate, Clim. Past, 12, 1805–1828, https://doi.org/10.5194/cp-12-1805-2016, 2016.
Hodell, D. A., Channell, J. E. T., Curtis, J. H., Romero, O. E., and Röhl, U: Onset of “Hudson Strait” Heinrich events in the eastern North Atlantic at the end of the middle Pleistocene transition (∼640 ka)?, Paleoceanography, 23, PA4218, https://doi.org/10.1029/2008PA001591, 2008.
Hodell, D. A., Lourens, L. J., Crowhurst, S. J., Konijnendijk, T. Y. M., Tjallingii, R., Jiménez-Espejo, F. J., Skinner, L. C., and Tzedakis, P. C.: Stable oxygen isotopic record of foraminifera from IODP Site 339-U1385, https://doi.org/10.1594/PANGAEA.872082, 2015.
Hoogakker, B. A., Rohling, E. J., Palmer, M. R., Tyrrell, T., and Rothwell, R. G: Underlying causes for long-term global ocean δ13C fluctuations over the last 1.20 Myr, Earth Planet. Sc. Lett., 248, 15–29, 2006.
Johannessen, T., Jansen, E., Flatoy, A., and Ravelo, A. C: The relationship between surface water masses, oceanographic fronts and paleoclimatic proxies in surface sediments of the Greenland, Iceland, Norwegian seas, NATO ASI Series, 117, edited by: Zahn, R. K., Pedersen, T. F., Kanimski, M. A., and Labeyrie, L., Springer-Verlag, New York, 61–85, 1994.
Imbrie, J., Berger, A., Boyle, E. A., Clemens, S. C., Duffy, A., Howard, W. R., Kukla, G., Kutzbach, J., Martinson, D. G., McIntyre, A., Mix, A. C., Molfino, B., Morley, J. J., Peterson, L. C., Pisias, N. G., Prell, W. L., Raymo, M. E., Shackleton, N. J., and Toggweiler, J. R.: On the structure and origin of major glaciation cycles 2: The 100 000 year cycle, Paleoceanography, 8, 699–735, 1993.
Kennett, J. P. and Srinivasan, M. S: Neogene Planktonic Foraminifera. A Phylogenetic Atlas, Hutchinson Ross Publishing Company, New York, 1983.
Lisiecki, L. E. and Raymo, M. E: A Pliocene-Pleistocene stack of 57 globally distributed benthic δ18O records, Paleoceanography, 20, PA1003, https://doi.org/10.1029/2004PA001071, 2005.
Maiorano, P., Marino, M., Balestra, B., Flores, J. A., Hodell, D. A., and Rodrigues, T: Coccolithophore variability from the Shackleton Site (IODP Site U1385) through MIS 16-10, Global Planet. Change, 133, 35–48, 2015.
Maslin, M. A. and Brierley, C. M: The role of orbital forcing in the early middle Pleistocene transition, Quatern. Int., 389, 47–55, 2015.
Martin-Garcia, G. M., Alonso-Garcia, M., Sierro, F. J., Hodell, D. A., and Flores, J. A: Severe cooling episodes at the onset of deglaciations on the Southwestern Iberian margin from MIS 21 to 13 (IODP site U1385), Global Planet. Change, 135, 159–169, 2015.
Martrat, B., Grimalt, J. O., Shackleton, N. J., de Abreu, L., Hutterli, M. A., and Stocker, T. F: Four Climate Cycles of Recurring Deep and Surface Water Destabilizations on the Iberian Margin, Science, 317, 502–507, 2007.
McCartney, M. S. and Talley, L. D: Warm-to-cold conversion in the northern North Atlantic Ocean, J. Phys. Oceanogr. 14, 922–935, 1984.
McIntyre, A., Ruddiman, W. F., and Jantzen, R: Southward penetrations of the North Atlantic Polar Front: faunal and floral evidence of large-scale surface water mass movements over the last 225 000 years, Deep-Sea Res., 19, 61–77, 1972.
McManus, J. F., Oppo, D. W., and Cullen, J. L: A 0.5-million.year record of millennial. scale climate variability in the North Atlantic, Science, 283, 971–975, 1999.
Mudelsee, M. and Schulz, M: The Mid-Pleistocene climate transition: onset of 100 ka cycle lags ice volume build-up by 280 ka, Earth Planet. Sc. Lett., 151, 117–123, 1997.
Naafs, B. D. A., Stein, R., Hefter, J., Khélifi, N., De Schepper, S., and Haug, G. H: Late Pliocene changes in the North Atlantic Current, Earth Planet. Sc. Lett., 298, 434–442, 2010.
Ottens, J. J: Planktic foraminifera as North Atlantic watermass indicators, Oceanol. Acta, 14, 123–140, 1991.
Peliz, A., Dubert, J., Santos, A. M. P., Oliveira, P. B., and Le Cann, B: Winter upper ocean circulation in the Western Iberian Basin – Fronts, eddies and poleward flows: an overview, Deep-Sea Res. Pt I, 52, 621–646, 2005.
Pflaumann, U., Dupratm, J., Pujol, C., and Labeyrie, L. D: SIMMAX: a modern analog technique to deduce Atlantic sea surface temperatures from planktonic Foraminifera in deep-sea sediments, Paleoceanography, 11, 15–35, 1996.
Pflaumann, U., Sarnthein, M., Chapman, M., de Abreu, L., Funnell, B., Huels, M., Kiefer, T., and Maslin, M: Glacial North Atlantic: sea-surface conditions reconstructed by GLAMAP 2000, Paleoceanography, 18, 1065, https://doi.org/10.1029/2002PA000774, 2003.
Poirier, R. K. and Billups, K: The intensification of northern component deepwater formation during the mid-Pleistocene climate transition, Paleoceanography, 29, 1046–1061, 2014.
Rahmstorf, S: Rapid climate transitions in a coupled ocean-atmosphere model, Nature, 372, 82–85, 1994.
Railsback, L. B., Gibbard, P. L., Head, M. J., Voarintsoa, N. R. G., and Toucanne, S: An optimized scheme of lettered marine isotope substages for the last 1.0 million years, and the climatostratigraphic nature of isotope stages and substages, Quaternary Sci. Rev., 111, 94–106, 2015.
Rios, A. F., Perez, F. F., and Fraga, F: Water Masses in the Upper and Middle North-Atlantic Ocean East of the Azores, Deep-Sea Res. Pt. I, 39, 645–658, 1992.
Rodrigues, T., Alonso-García, M., Hodell, D. A., Rufino, M., Naughton, F., Grimalt, J. O., Voelker, A. H. L., and Abrantes, F: A 1-Ma record of sea surface temperature and extreme cooling events in the North Atlantic: A perspective from the Iberian Margin, Quaternary Sci. Rev., 172, 118–130, 2017.
Rodríguez-Tovar, F. J., Dorador, J., Martin-Garcia, G. M., Sierro, F. J., Flores, J. A., and Hodell, D. A.: Response of macrobenthic and foraminifer communities to changes in deep-sea environmental conditions from Marine Isotope Stage (MIS) 12 to 11 at the “Shackleton Site”, Global Planet. Change, 133, 176–187, 2015.
Ruddiman, W. F. and McIntyre, A: Ice-age thermal response and climatic role of the surface Atlantic Ocean, 40∘N to 63∘N, Geol. Soc. Am. B., 95, 381–396, 1984.
Ruddiman, W. F., Raymo, M. E., Martinson, D. G., Clement, B. M., and Backman, J.: Stable isotope record, calcium carbonate conentrations, and sea surface temperture reconstructions of sediment cores from the North Atlantic, https://doi.org/10.1594/PANGAEA.701229, 1989.
Ruddiman, W. F., Raymo, M. E., Martinson, D. G., Clement, B. M., and Backman, J: Sea surface temperature reconstruction of DSDP Site 94-607 in the North Atlantic, https://doi.org/10.1594/PANGAEA.52373, 1989.
Sabine, C. L., Feely, R. A., Gruber, N., Key, R. M., Lee, K., Bullister, J. L., Wanninkhof, R., Wong, C. S., Wallace, D. W. R., Tilbrook, B., Millero, F. J., Peng, T. H., Kozyr, A., Ono, T., and Rios, A. F.: The oceanic sink for anthropogenic CO2, Science, 305, 367–371, 2004.
Salgueiro, E., Voelker, A., Abrantes, F., Meggers, Pflaumann, U., Loncaric, N., González-Álvarez, R., Oliveira, P., Bartels-Jónsdóttir, H. B., Moreno, J., and Wefer, G.: Planktonic foraminifera from modern sediments reflect upwelling patterns off Iberia: Insights from a regional transfer function, Mar. Micropaleontol., 66, 135–164, 2008.
Salgueiro, E., Voelker, A. H. L., de Abreu, L., Abrantes, F., Meggers, H., and Wefer, G: Temperature and productivity changes off the western Iberian margin during the last 150 ky, Quaternary Sci. Rev., 29, 680–695, 2010.
Schiebel, R. and Hemleben, C: Planktic foraminifers in the modern ocean, 358 pp., Springer-Verlag Ed., Berlin Heidelberg, 2017.
Schmitz, W. J. and McCartney, M. S: On the North Atlantic circulation, Rev. Geophys., 31, 29–49, 1993.
Stein, R., Hefter, J., Grützner, J., Voelker, A., and Naafs, B. D. A: Variability of surface water characterstics and Heinrich-like events in the Pleistocene midlatitude North Atlantic Ocean: Biomarker and XRD records from IODP Site U1313 (MIS 16-9), Paleoceanography 24, PA2203, https://doi.org/10.1029/2008PA001639, 2009.
Stow, D. A. V., Hernández-Molina, F. J., Alvarez-Zarikian, C. A., and Expedition 339 Scientists: Mediterranean outflow: environmental significance of the Mediterranean Outflow Water and its global implications, IODP Preliminary Report, 339, https://doi.org/10.2204/iodp.pr.339, 2012.
Vautravers, M. J., Shackleton, N. J., Lopez-Martinez, C., and Grimalt, J. O: Gulf Steam variability during marine isotope stage 3, Paleoeanography, 19, PA2011, https://doi.org/10.1029/2003PA000966, 2004.
Villanueva, J., Calvo, E., Pelejero, C., Grimalt, J. O., Boelaert, A., and Labeyrie, L: A latitudinal productivity band in the Central North Atlantic over the last 270 kyr: an alkenone perspective, Paleoceanography, 16, 617–626, 2001.
Voelker, A. H. L., Rodrigues, T., Billups, K., Oppo, D., McManus, J., Stein, R., Hefter, J., and Grimalt, J. O.: Variations in mid-latitude North Atlantic surface water properties during the mid-Brunhes (MIS 9-14) and their implications for the thermohaline circulation, Clim. Past, 6, 531–552, https://doi.org/10.5194/cp-6-531-2010, 2010.
Wright, A. K. and Flower, B. P: Surface and deep ocean circulation in the subpolar North Atlantic during the mid-Pleistocene revolution, Paleoceanography, 17, 1068, https://doi.org/10.1029/2002PA000782, 2002.
Wright, A. K. and Flower, B. P.: Subpolar North Atlantic mid-Pleistocene Faunal Census and SST Data, available at: ftp://ftp.ncdc.noaa.gov/pub/data/paleo/contributions_by_author/wright2002/wright2002.txt (last access: 1 February 2018), 2009.
Zahn, R., Schönfeld, J., Kudrass, H. R., Park, M. H, Erlenkeuser, H., and Grootes, P: Thermohaline instability in the North Atlantic during meltwater events: Stable isotope and ice-rafted detritus records from core SO75-26KL, Portuguese margin, Paleoceanography, 12, 696–710, 1997.