the Creative Commons Attribution 4.0 License.
the Creative Commons Attribution 4.0 License.
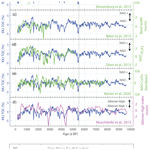
Mid-Holocene reinforcement of North Atlantic atmospheric circulation variability from a western Baltic lake sediment record
Markus Czymzik
Rik Tjallingii
Birgit Plessen
Peter Feldens
Martin Theuerkauf
Matthias Moros
Markus J. Schwab
Carla K. M. Nantke
Silvia Pinkerneil
Achim Brauer
Helge W. Arz
Knowledge about the timing, amplitude and spatial gradients of Holocene environmental variability in the circum-Baltic region is key to understanding its responses to ongoing climate change. Based on a multi-dating and proxy approach, we reconstruct changes in productivity using total organic carbon (TOC) contents in sediments of Lake Kälksjön (KKJ) from west–central Sweden spanning the last 9612 () years. An exception is the period from 1878 CE until today, in which sedimentation was dominated by anthropogenic lake level lowering and land use. In-lake productivity was higher during periods of warmer winters with shortened ice cover and prolonged growing seasons. A multi-millennial increase in productivity throughout the last ∼ 9600 years is associated with progressively warmer winters in northwestern Europe, likely triggered by the coinciding increase in Northern Hemisphere winter insolation. Decadal to centennial periods of higher productivity in KKJ tend to correspond to warmer winters during a more positive North Atlantic Oscillation (NAO) polarity, as reconstructed for the last 8000 years. In consequence, we assume our decadal to centennial productivity record from KKJ sediments for the complete ∼ 9600 years to provide a qualitative record of NAO polarity. A shift towards higher productivity variability at ∼ 5450 cal a BP is hypothesized to reflect a reinforcement of NAO-like atmospheric circulation variability, possibly driven by more vigorous changes in North Atlantic deep-water formation.
- Article
(10924 KB) - Full-text XML
- BibTeX
- EndNote
The circum-Baltic ecosystems are sensitive to both natural climate forcing and human impact (Andrén et al., 2000; BACC II, 2015; Warden et al., 2017). Existing studies from these regions point to considerable paleoenvironmental variability and spatial gradients active on interannual to millennial timescales, triggered by an interplay of internal and external forcing mechanisms (BACC II, 2015; Moros et al., 2020; Zillén et al., 2008). Particularly the western “south–central Scandinavian” region of the Baltic realm experienced a unique Holocene development. Postglacial melting of the Fennoscandian ice sheet led to isostatic rebound of up to 300 m, eustatic sea level changes and shoreline displacements (Berglund, 2004). In addition, the sheltering effect of the Scandinavian mountains north of 62∘ N results in varying expressions of the prevailing North Atlantic climate (Blenckner et al., 2004; Hammarlund et al., 2003; Snowball et al., 1999). Existing paleoenvironmental information provides little detail about the possible ranges and gradients of atmospheric circulation changes, and a better delimitation of such changes can provide a more comprehensive understanding of past natural climate variability in the western Baltic region and, ultimately, circum-Baltic climate change. Lake sediment records can provide this type of information. The composition and geochemical features of their sediment columns are modulated by past climate and anthropogenic changes in their catchment (Labuhn et al., 2018; Zolitschka et al., 2015).
Lake Kälksjön (KKJ) in west–central Sweden is well-suited for the reconstruction of changes in synoptic atmospheric circulation for most of the Holocene. Previous studies have proven the KKJ sediment column to be a continuous archive of secular changes in Earth's magnetic field and winter precipitation connected with the 8.2 ka cold event (Randsalu-Wendrup et al., 2012; Snowball et al., 2010; Stanton et al., 2010, 2011). Its position in central Sweden at 60∘ N is sensitive to changes in the North Atlantic climate and not substantially affected by the orographic shielding of the Scandinavian mountains (Hurrell, 1995; Uvo, 2003). In this study, we apply a multi-proxy and dating approach to reconstruct changes in lake productivity and deduce the driving climate and human factors throughout the last ∼ 9600 years.
KKJ is located in west–central Sweden at an altitude of 97 m a.s.l. (60∘09′ N, 13∘03′ E) (Fig. 1) (Stanton et al., 2010; Zillén et al., 2003). The modern basin was isolated from ancient Lake Vänern through isostatic rebound following the last deglaciation. KKJ has a surface area of 0.42 km2, maximum water depth of 13.6 m and catchment size of 4 km2 (Fig. 1) (Stanton et al., 2011). Four creeks discharge into the lake from the hilly and forested northeastern shores. Its only outflow in the west was artificially incised in 1878 CE to lower the lake level and enlarge the grassland for stockbreeding in the southern and western catchment (Fig. 2). In October 1993 and 1999 CE, 64.2 and 67.3 t of chalk flour were distributed in the lake to raise the water pH level, respectively (Stanton et al., 2010).
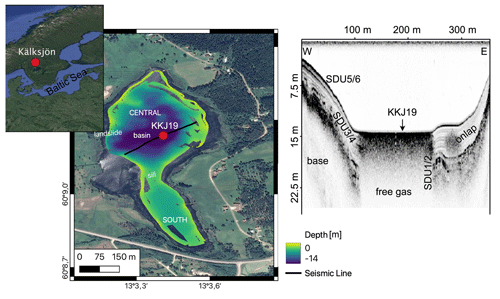
Figure 1Geographical location of Lake Kälksjön (KKJ) in the western Baltic region and a bathymetric map of the basin with the coring location of composite profile KKJ19 (© 2015 Google Maps). A seismic line crossing the coring location displays the sedimentary sequence of KKJ. The expected seismic reflections of the six sediment deposition units (SDUs) in KKJ19 are indicated.
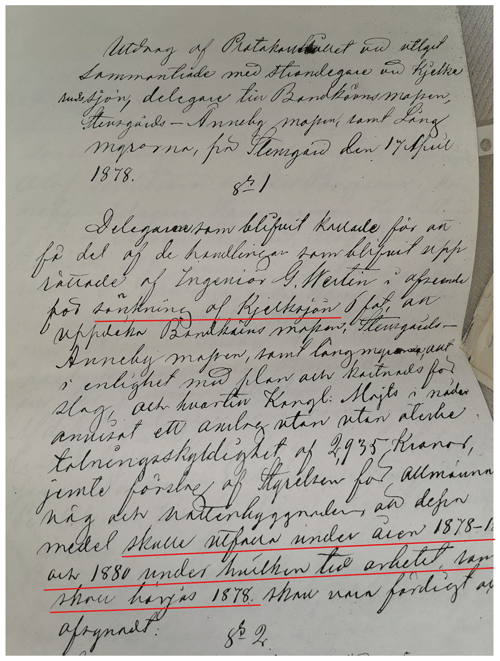
Figure 2Historic document indicating that lake level lowering of Lake Kälksjön (avsänkning of Kjelksjön) was performed within the years 1878 to 1880 CE (skulle utföra under aren 1878–1??? och 1880 …) and that the work started in 1878 CE (… under vilken tid arbetet som skulle börjas 1878). Source: local historian.
Today's climate at KKJ is controlled by its location at the transition between the temperate and subpolar zones. Mean monthly temperatures vary between 16 ∘C in July and −6 ∘C in January (Fig. 3). Lake ice cover in the KKJ area usually lasts from the end of November until the end of April (Blenckner et al., 2004). Annual mean precipitation is 720 mm with a maximum during July and August (Fig. 3).
3.1 Hydroacoustic survey
Hydroacoustic bathymetry surveys were performed using a portable Norbit iWBMSe device with a central frequency of 400 and 80 kHz bandwidth. Data were recorded with the Norbit GUI in s7k format and processed using mbsystem (Caress and Chayes, 1996), correcting for sound velocity, manually removing outliers and creating grids of 0.5 m resolution. Seismic data were recorded using a parametric Innomar 96 sediment echo sounder, with the low frequency set to 10 kHz and a vertical resolution of < 6 cm. Data were processed and plotted with Seismic Unix by applying a bandpass filter, binning to 1 m intervals and a time-varied gain (Stockwell, 1999). Two-way travel times were converted to meters by applying a sound velocity of 1500 m s−1. Positional data for the bathymetric and seismic surveys were provided by a POSMV Seastar system working with the EGNOS correction and a spatial accuracy of ∼ 45 cm.
3.2 Composite profile KKJ19
Parallel and overlapping sediment cores KKJ19-A and KKJ19-B were retrieved in August 2019 from the deepest part of KKJ using an UWITEC piston corer. The cores consist of 2 m long segments, with a diameter of 9 cm. Two surface sediment cores were retrieved from the same position with an UWITEC short core system to reduce process-based disturbances of the water-soaked sediment surface. Composite profile KKJ19 was constructed through core-to-core correlation of macroscopic lithological layers and has a total length of ∼ 8 m.
3.3 Microfacies analyses
Microfacies analyses of sediment properties were performed on a series of 80 overlapping petrographic thin sections (10×2 cm) from composite profile KKJ19. Microscopic investigations were performed using a ZEISS Axiolab polarization microscope at 25× to 200× magnification under varying light and optical conditions. Microscopic particle size measurements were performed at 200× magnification. Thin-section preparation from unconsolidated sediments includes shock-freezing with liquid nitrogen, freeze-drying and epoxy resin impregnation under vacuum (Czymzik et al., 2018).
3.4 Geochemistry
Total organic carbon (TOC) contents, δ13C values of organic matter (δ13Corg) and C N ratios were measured at 1 cm resolution from freeze-dried and homogenized sediment samples using an EA Isolink elemental analyzer coupled to a DELTA V advantage isotope ratio mass spectrometer (Thermo Fisher Scientific). Before the measurements, the samples were weighted into Ag capsules, in situ decalcified, first with 3 % and second with 20 % HCl, and dried for 3 h at 75 ∘C. The calibration was performed based on an elemental (urea) and certified isotope standard (IAEA-CH-7: δ13C −31.8 ‰) and checked with an internal soil reference sample (Boden3, HEKATECH). Replicate analyses of the standards resulted in a reproducibility of 0.2 wt % for TOC and 0.2 ‰ for δ13Corg.
Element intensity profiles were acquired for composite profile KKJ19 from the cleaned split-core surface using an ITRAX X-ray fluorescence core scanner (Croudace et al., 2019). Measurements were conducted with a Cr X-ray source operated at 30 kV and 60 mA that irradiated a surface of 0.2×8 mm for 3 s at each sample position. Additionally, twofold replicate measurements were acquired for each core section at two or three intervals of at least 2 cm length and with distinctively different sedimentological compositions. Based on the proportion of zero values (< 5 %) and the relative standard deviation (< 15 %) the elements Al, Si, S, K, Ca, V, Ti, Mn and Fe were selected for investigation. Finally, the initial measurements at 200 µm step size were resampled to a 2 mm resolution.
Centered log-ratio transformation of element intensities lifts the compositional constraints and permits rigorous statistical analyses (Aitchison, 1982, 1986). For this advantage, centered log ratios of element intensities were used for principal component analyses and calculating groups of similar compositions by Ward's hierarchical clustering (Martin-Puertas et al., 2017). Replicate measurements were used for estimating confidence limits and reducing the influence of noise on the statistical analyses. All statistical analyses were performed using the Xelerate software package (Weltje et al., 2015).
3.5 Pollen analysis
Pollen sample preparation includes treatment with 25 % HCl, 10 % KOH, acetolysis at 100 ∘C and 40 % cold HF (Fægri and Iversen, 1989). The final samples were washed with ethanol and transferred into silicon oil. Pollen abundances were analyzed at 400× magnification with a Zeiss Axiolab microscope. To estimate pollen concentrations and accumulation, three tablets with exotic marker grains (Lycopodium clavatum spores, batch no. 050220211) were added to each sample before preparation. Pollen analyses in KKJ sediments were carried out for the intervals from 0 to 63 cm composite depth to cover the main period of human activity and 260 to 410 cm composite depth including the transition from sediment deposition unit (SDU) 3 to 4.
3.6 Chronology
An age model for composite profile KKJ19 was calculated using the Oxcal software working with a P-sequence model and the IntCal20 calibration curve (Bronk Ramsey, 2008; Reimer et al., 2020). The calculations were carried out based on 14C ages of plant macrofossils and lithological marker layers of known age. Measurements of 137Cs and 241Am contents were performed with a Canberra Ge-detector BE3830-7500SL-RDC-6-ULBB gamma spectrometer (Moros et al., 2017).
4.1 Bathymetry
KKJ is separated into a 6.1 m deep southern and 13.6 m deep central basin, divided by a sill with ∼ 4 m water depth (Fig. 1). The deepest part of the central basin and coring location of composite profile KKJ19 is flat with slopes < 1∘. It has a maximum E–W extension of 300 m and N–S extension of 330 m (Fig. 1). A single elevation with 9.8 m water depth is located in the central basin's northern part. Its flat part reaches within 50 m to the western shore, resulting in slope angles of > 20∘. There, morphological remnants of a minor landslide extend 60 m into the lake (Fig. 1).
4.2 Sediment deposition units
Composite profile KKJ19 can be subdivided into six sediment deposition units (SDUs). Boundaries between the SDUs are mainly characterized by shifts in organic and detrital matter contents (Figs. 4 and 5). In the deep central basin including the coring location, the seismic data are masked by the presence of free gas (Fig. 1). In consequence, we connect the seismic records from the basin onset and margin, without masking gas, to the SDUs in sediment core KKJ19 (Fig. 1).
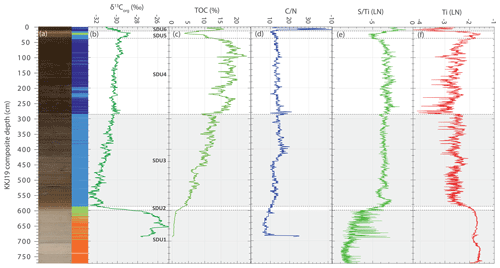
Figure 4Composite profile KKJ19 with selected proxy records. (a) Photograph of composite profile KKJ19 with XRF-based element cluster stratigraphy and corresponding (b) δ13Corg, (c) total organic carbon (TOC), (d) C N, (e) S Ti and (f) Ti records. Sediment deposition units (SDUs) 1 to 6 are indicated.
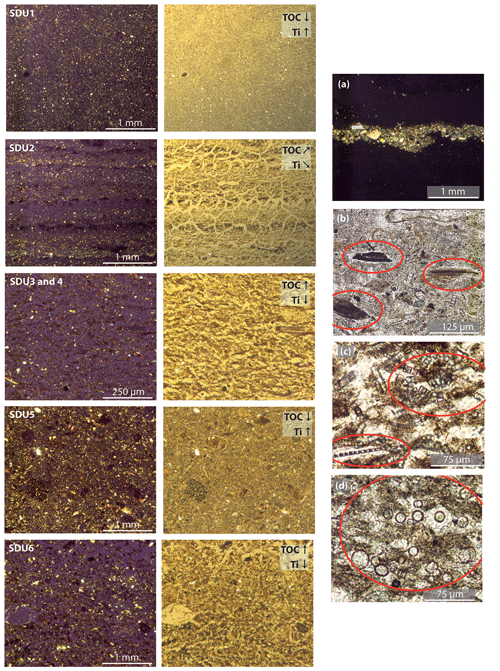
Figure 5(left) Overview micrographs of Lake Kälksjön (KKJ) sediment deposition units (SDUs) 1 to 6 under polarized and plane light. Relative abundances of TOC and Ti contents in each SDU are indicated. (right) Micrographs highlighting individual sediment components. (a) Liming layer from 1993 CE (5 cm composite depth, polarized light), (b) terrestrial organic debris in SDU 5 (18 cm composite depth, plane light), (c) diatom frustules (Aulacoseiraceae and Fragilariaceae) in SDU 3 (305 cm composite depth, plane light) and (d) crysophyte cysts in SDU 3 (298 cm composite depth, plane light).
SDU 1 (772 to 600 cm composite depth). SDU 1 is composed of homogenous clay- to silt-sized detrital grains (Fig. 5), including quartz, feldspar and mica. The predominance of the detrital material is reflected by high Ti values, low TOC contents of ∼ 1 % and δ13Corg values up to −25 ‰ (Fig. 4).
SDU 2 (600 to 583 cm composite depth). SDU 2 is characterized by progressively increasing deposition of organic material, from sporadic layers to a massive organic-rich unit and decreasing abundances of detrital grains (Fig. 5). Accordingly, SDU 2 depicts an increase in TOC contents from 2 % to 4 % and a drop in Ti values (Fig. 4). While the change from SDU 2 to the overlying SDU 3 appears sharp at 583 cm composite depth in the microscopic observations, the drop in Ti points to a slightly thicker SDU 2 reaching up to 570 cm composite depth (Fig. 4). SDUs 1 and 2 likely correspond to the upper part of a seismic unit situated on top of the acoustic base with a chaotic internal texture and few internal laminations. The top of this unit is marked by a high-amplitude seismic unconformity (Fig. 1).
SDU 3 (583 to 287 cm composite depth). KKJ sediments in SDU 3 are predominantly composed of amorphous and particulate organic material, with some incorporated benthic diatoms and crysophyte cysts (Fig. 5). Within the course of SDU 3, TOC contents increase from 4 % at 583 cm to 12 % at 287 cm composite depth, superimposed by centimeter-scale fluctuations (Fig. 4). Low δ13Corg values increase from below −32 ‰ to up to −30 ‰, broadly in phase with the TOC time series (Fig. 4). Excluding minor peaks, C N ratios vary between 14 and 16 (Fig. 4).
SDU 4 (287 to 37 cm composite depth). Sedimentological and geochemical features of SDU 4 are comparable to those of SDU 3. The main distinction from SDU 3 is enhanced centimeter-scale proxy fluctuations (Fig. 4). TOC contents show a continuing increase with maxima of ∼ 20 % in the upper part of SDU 4 (Fig. 4). Even though less distinct, δ13Corg and S Ti values resemble the trend present in the TOC record (Fig. 4). C N ratios within SDU 4 range from about 11 to 14 (Fig. 4). Non-arboreal pollen (NAP) upland and wild grass group pollen show a minor increase at the transition from SDU 3 to 4, but then return to low values (Fig. 6). A more distinct increase in these settlement indicators occurs at 55 cm composite depth. SDUs 3 and 4 are not readily differentiated in the seismic data and correspond to a unit with parallel internal laminations that is widespread in the northern basin. Its maximum observed thickness is 5 m, but the unit thickness is expected to increase in the deepest part of the basin and the core location, which could not be imaged due to the presence of free gas. The unit onlaps morphological elevations within the northern basin, where its thickness decreases to less than 1 m (Fig. 1).
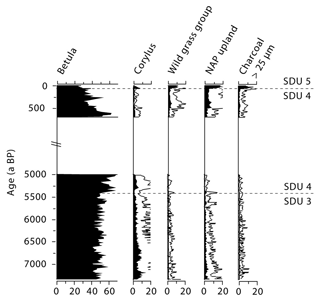
Figure 6Pollen records from Lake Kälksjön (KKJ) sediments covering the transitions between SDUs 3 and 4, as well as SDUs 4 and 5.
SDU 5 (37 to 12 cm composite depth). Detrital matter contents in SDU 5 increase from 37 cm, reach a maximum around 20 cm and decrease back to background values until 12 cm composite depth (Fig. 4). Littoral diatoms and organic macro-remains are incorporated in the detrital matrix (Fig. 5). Maximum detrital grain size is 50 µm. Variations of detrital matter abundance as indicated by the Ti record vary anti-phased with the TOC contents that decrease from 18 % to 4 %, before rising back to 16 % (Fig. 4). At the onset of SDU 5, wild grass group and NAP upland pollen percentages, as well as the abundance of micro-charcoal particles > 25 µm, show a distinct increase (Fig. 6).
SDU 6 (12 to 0 cm composite depth). KKJ sediments in SDU 6 in general resemble the organic-rich deposits in SDU 4, with high TOC contents of about 15 % and low Ti values (Fig. 4). One exception is the peak in C N ratios up to 42, corresponding to the liming layers (Fig. 4). Wild grass group and NAP upland pollen percentages, as well as the abundance of micro-charcoal particles, remain high (Fig. 6). SDUs 5 and 6 correspond to the uppermost homogeneous section of the seismic sequence. It is separated from the underlying sediments by a high-amplitude seismic reflection (Fig. 1). The unit is best observed at the basin's margins but can be traced into the central basin (Fig. 1).
Our stratigraphic subdivision is in general agreement with that of the KKJ sediment core investigated by Stanton et al. (2010). Minor differences in sedimentation rates are likely attributable to slightly different coring locations (∼ 100 m distance) and dissimilar coring techniques (Stanton et al., 2010). By contrast to the results of Stanton et al. (2010), our investigations did not reveal annually laminated (varved) sediments for most of composite profile KKJ19. Possible reasons might be slight lateral changes in varve preservation within the central basin of KKJ and/or sediment micro-disturbances caused during thin-section preparation. In addition, their varve counts from modified photographs might have revealed sedimentological features that are not visible in our microscopic observations.
4.3 Statistical analyses of XRF profiles
Element correlations are presented in Table 1. The clustering results are visualized in a principal component (PC) biplot showing that PC1 explains 86.3 % and PC2 7.7 % of the variance (Fig. 7). Variations of PC1 are dominated by the negative correlation between the lithogenic elements (Si, Ti and K), as well as S and Fe (Fig. 7). The positive correlation of Si with the detrital elements Ti and K indicates that diatom productivity plays a minor role in changing silica contents in KKJ sediments (Fig. 7, Table 1). Although organic matter cannot be measured by XRF core scanning, the positive correlation between S and TOC (r=0.83, p < 0.01) reveals that S is a proxy for organic matter accumulation in KKJ sediments (Fig. 8). Therefore, the S Ti ratio can be used to trace relative variations of organic and detrital material in KKJ19 sediments (Fig. 4). Moreover, our element clustering reveals a strong divide between the detrital and organic-rich sediments. The negative correlation between Ca and Mn dominates the variations in PC2, which matches the deviation found in the organic-rich sediments of clusters 3 and 4 (Fig. 7).
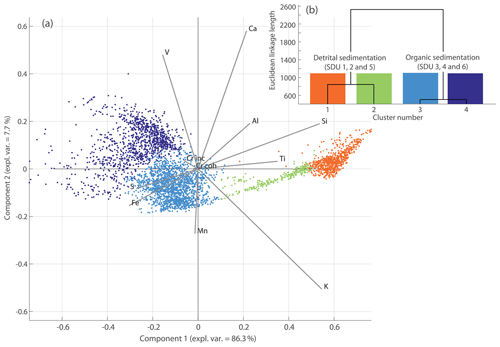
Figure 7Statistical analyses of the XRF profiles from Lake Kälksjön (KKJ) sediments. (a) Covariance biplot visualizing the correlations of the main elements with regard to the first two principal components. (b) Hierarchical clustering solution reflecting the difference between the detrital sediments of SDUs 1, 2 and 5, as well as organic sediments of SDUs 3, 4 and 6.
The four-cluster solution matches the main sedimentological changes described by the six SDUs well (Fig. 4). These four clusters can be related to the detrital sediments of SDU 1 (cluster 1) and the transition unit SDU 2 (cluster 2), as well as the organic-rich sediments of SDU 3 (cluster 3) and SDU 4 (cluster 4) (Fig. 4). The sediments in SDU 5 have a similar composition as in SDU 2 (both cluster 2), whereas the composition of the sediments in SDU 6 is similar to that in SDU 4 (both cluster 4). Organic sediments in cluster 3 are characterized by lower δ13Corg values and TOC contents than those in cluster 4, as well as reduced variability (Fig. 8). Detrital sediments in cluster 1 contain less TOC than those in cluster 2, but about the same amount of Ti (Fig. 8). Sediments of SDU 3 (cluster 3) and SDU4 (cluster 4) are characterized by low amounts of Ti and higher amounts of S and TOC, while the amount of TOC is generally lower in sediments of cluster 3 than in those of cluster 4 (Fig. 8).
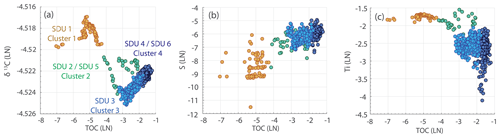
Figure 8Comparison of bulk geochemistry and XRF data from composite profile KKJ19. (a) δ13C of organic material (δ13Corg) and total organic carbon (TOC). (b) S and TOC. (c) Ti and TOC. Colors of the data points correspond to those of the cluster analysis. XRF profiles were resampled to the resolution of the bulk geochemistry records.
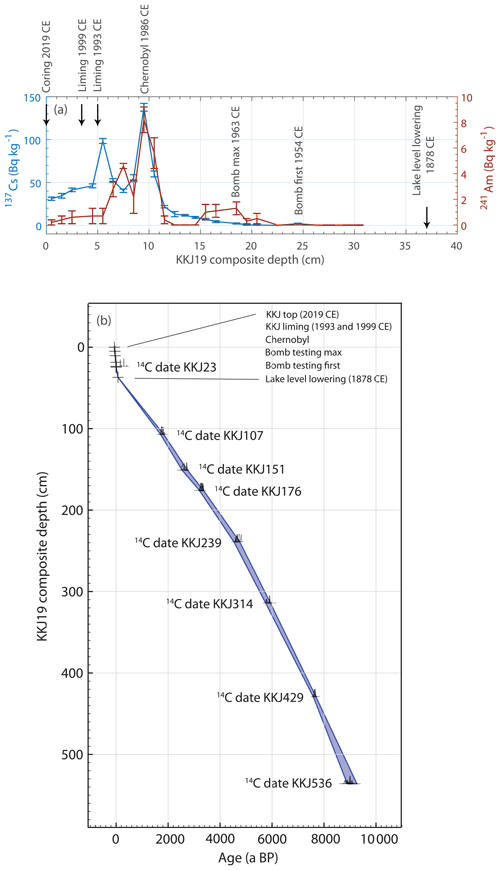
Figure 9Age–depth model for composite sediment profile KKJ19. (a) 137Cs and 241Am dating of the topmost sediments and positions of lithological marker layers of known age. (b) Calibrated 14C dates from eight plant macrofossils. The age–depth model was constructed using the OxCal software working with the IntCal20 calibration curve (Bronk Ramsey, 2008; Reimer et al., 2020).
4.4 Chronology
A chronology for composite profile KKJ19 was constructed back to 9612 () cal a BP (base of SDU 3 at 583 cm composite depth) using 8 14C dates from terrestrial plant macrofossils (Table 2), concentrations of the artificial radionuclides 137Cs and 241Am in the upper 31 cm, and four marker horizons of known age (Fig. 9). Since no plant macrofossils were picked below 536 cm composite depth ( cal a BP), no age information is available for the detrital SDUs 1 and 2.
Table 2Radiocarbon (14C) ages of terrestrial plant macrofossils from composite profile KKJ19 with sediment depths.
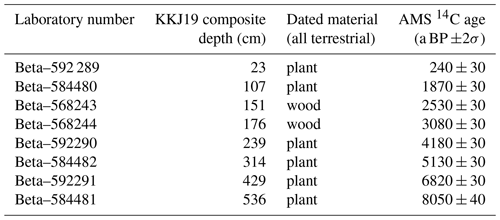
Massive amounts of 137Cs and 241Am were released to the global atmosphere by surface nuclear weapon tests starting in 1954 CE and peaking in 1963 CE (Pennington et al., 1973). A second major release of 137Cs mainly affecting the European continent occurred during the Chernobyl nuclear power plant accident in 1986 CE (Povinec et al., 2003). The associated fallout events are documented in KKJ sediments at 24.5, 18.5 and 10.5 cm composite depth (Fig. 9). The 137Cs- and 241Am-based age–depth model for the upper part of composite profile KKJ19 is independently validated by four marker horizons of known age: (I) the top of composite profile KKJ19 reflecting the coring year 2019 CE, (II + III) two lime layers at 3.5 and 5 cm composite depth indicating lake chalking in 1999 and 1993 CE, and (IV) an increase in detrital matter input at 37 cm composite depth reflecting anthropogenic lake level lowering in 1878 CE (Figs. 2 and 9) (Stanton et al., 2010). In contrast to the results from the previous KKJ sediment core (Stanton et al., 2011), our microfacies analyses revealed no continuously varved sediments that could be used for chronological purposes for most of composite profile KKJ19.
5.1 Holocene evolution of Lake Kälksjön
The succession of six SDUs in composite profile KKJ19 can be interpreted in terms of different lake stages associated with changing environmental conditions and human impact in KKJ and its catchment.
SDU 1 (pre-isolation phase). Homogenous clay and fine-silt detrital material, as well as high Ti and low TOC contents, suggest sediment deposition in an offshore location before the isolation of KKJ from ancient Lake Vänern (Figs. 4 and 5) (Björck, 1995). This is supported by the corresponding detrital element cluster 1, accompanied by the highest Ti and lowest TOC (∼ 1 %) contents of the record (Figs. 7 and 8). The material was most likely discharged into the basin during the postglacial retreat of the Fennoscandian ice sheet (Risberg et al., 1996; Stanton et al., 2010).
SDU 2 (transition phase). A shift from a predominantly detrital to an organic deposition within SDU 2 is accompanied by the detrital element cluster 2, as well as decreasing Ti and increasing TOC contents, interpreted to reflect the transition towards a more local sediment source from the establishing catchment of KKJ (Figs. 4 and 7). The likely trigger of these changes is the gradual isolation of the small lacustrine KKJ basin from ancient Lake Vänern through isostatic rebound and drainage of Lake Vänern via the Göta Älv into the Kattegat (Björck, 1995).
SDU 3: lacustrine sedimentation from 9612 () cal a BP to 5434 () cal a BP, mean sedimentation rate 14 a cm−1. The full isolation of KKJ at 9612 () cal a BP at the base of SDU 3 is reflected by the onset of continuous organic-rich sedimentation with benthic diatoms and crysophyte cysts, as well as high TOC and low Ti contents (Figs. 4 and 5).
Our microscopically determined point of isolation is seemingly in contrast with that of the former KKJ sediment core at 9193±186 cal a BP, defined by the onset of varve formation 30 cm above the onset of organic deposition at the base of SDU 3 (Stanton et al., 2010), which is not detectable in our microscopic observations. However, considering the continuing drop in Ti at the base of SDU 3 until 9452 () cal a BP to reflect this stratigraphic point would reconcile the “isolation ages” from both KKJ sediment cores within errors (Fig. 4) (see Sect. 5.2 for a detailed discussion of SDUs 3 and 4).
SDU 4: lacustrine sedimentation from 5434 () cal a BP to 1878 CE, mean sedimentation rate 21 a cm−1. Even though of similar composition, the TOC, S Ti and Ti records in SDU 4 reveal enhanced variability compared to SDU 3 (Fig. 4). In addition, the change from SDU 3 to 4 is accompanied by a shift from the objectively determined element cluster 3 to 4 (Fig. 4) (see Sect. 5.2 for a detailed discussion of SDUs 3 and 4).
SDU 5: lake level lowering from 1878 to 1981 CE (), mean sedimentation rate 4 a cm−1. A rapid increase in detrital sedimentation with high Ti and low TOC contents in SDU 5 occurred concurrent with the onset of anthropogenic lake level lowering in 1878 CE (Figs. 2 and 4). This rapid sedimentological change is paralleled by the return to the detrital element cluster 2, interpreted to reflect detrital sedimentation from a local sediment source (Fig. 4). The detrital material likely originates from the non-consolidated former shallow-water zone of KKJ. Few distinct detrital layers point to an event-based transport and deposition during snowmelt floods or heavy precipitation (Czymzik et al., 2010; Tiljander et al., 2003). The approximately 100-year-long duration of SDU 5 presumably indicates the time span of soil formation and vegetation growth under the influence of considerable land use. A sharp increase in settlement indicators (NAP upland and wild grass group pollen) and micro-charcoal particles at the onset of SDU 5 suggests that land use activity in the vicinity of the lake intensified concurrent with lake level lowering (Fig. 6).
SDU 6: post-lake level lowering from 1981 () to 2019 CE, mean sedimentation rate 3 a cm−1. Sediment composition and geochemistry in SDU 6 are similar to that in SDU 4, suggesting a return to a relatively undisturbed lacustrine sedimentation (Fig. 4). Palynologic settlement indicators in KKJ sediments reveal a continuing human presence (Fig. 6).
5.2 Mechanism of organic matter accumulation in SDUs 3 and 4
Within the organic-rich SDUs 3 and 4, TOC contents in KKJ sediments reveal marked short- and long-term variability (Fig. 4). Changing TOC contents in lake sediments are controlled by an interplay of productivity in the water column, supply from the catchment and post-depositional degradation (Meyers and Teranes, 2001). Comparing our TOC record during SDUs 3 and 4 with the δ13Corg and pollen data from composite profile KKJ19 allows us to disentangle the main control on organic matter accumulation in KKJ sediments.
δ13Corg has been established as a proxy for productivity in the photic zone of lacustrine systems (Meyers, 1994; Stuiver, 1975). The dissolved organic carbon pool of a lake becomes enriched in 13C due to the preferential uptake of lighter 12C by phytoplankton (Teranes and Bernasconi, 2005). In consequence, phases of enhanced lake productivity are characterized by higher δ13Corg values. The parallel increase in TOC and δ13Corg in SDUs 3 and 4 hence suggests that increasing organic matter accumulation is predominantly attributable to higher productivity in the water column (Fig. 4). A further major influence on the δ13Corg signature through varying inputs of allochthonous organic matter (Meyers, 1994) is unlikely because of the microscopically determined rather homogenous composition of the organic sediment fraction during that period (Fig. 5). This is confirmed by C N ratios between 11 and 16 in SDUs 3 and 4, indicating a rather stable and predominantly aquatic source of the organic material (Meyers, 1994) (Fig. 4).
To summarize, based on the covariance with the δ13Corg record, we interpret changes in the TOC record from KKJ to mainly reflect varying in-lake productivity. We consider major human influences on productivity changes in KKJ during SDUs 3 and 4 as unlikely since we only detect a minor increase in human settlement indicators (NAP upland and wild grass group pollen) at the transition of SDU 3 to 4, followed by a return to low values (Fig. 6). Also, more distinct rises in these settlement indicators in KKJ sediments at 500 cal a BP and sediments of the nearby Lake Kårebolssjön (∼ 30 km northeast of KKJ) at 2100 a BP are not paralleled by similar changes in our TOC and δ13Corg records (Figs. 4 and 6) (Eddudóttir et al., 2021).
5.2.1 Millennial productivity trend and orbital forcing
The millennial upward trend in TOC contents (and simultaneously increasing δ13Corg values) interpreted to reflect progressively increasing productivity in KKJ reveals a sharper increase until the mid-Holocene, followed by a more moderate rise until recent times (Fig. 10). This trend is paralleled by progressively warmer winter temperatures in northwestern Europe reconstructed from pollen records, interpreted to be mainly caused by a continuous increase in orbital Northern Hemisphere winter insolation (Davis et al., 2003; Laskar et al., 2004; Wanner et al., 2008) (Fig. 10). Enhanced productivity in KKJ associated with warmer winters is best explained by shortened ice cover (on average 5 months today) allowing a prolonged growing season in spring and summer and increased metabolic rates (Karlsson et al., 2005; Willemse and Törnqvist, 1999). Winter temperature and coupled ice cover duration were reported as a main control of productivity from monitoring and model studies of multiple Swedish lakes covering a wider latitudinal and altitudinal range (Blenckner et al., 2004; Karlsson et al., 2005) (see Sect. 5.2.2 for details).
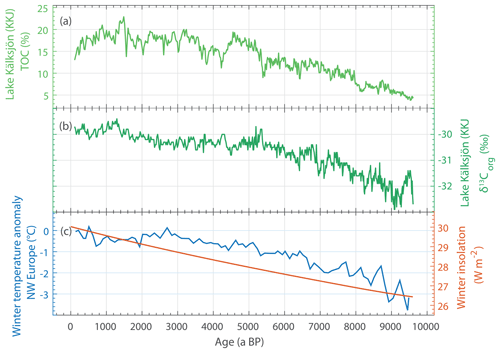
Figure 10Multi-millennial trends in paleoclimate and orbital forcing during the last ∼ 9600 years. (a) Total organic carbon (TOC) contents from Lake Kälksjön (KKJ) sediments. (b) δ13Corg from KKJ sediments. (c) Winter (January) insolation at 60∘ N (Laskar et al., 2004) and winter temperature anomaly in northwestern Europe (Davis et al., 2003).
5.2.2 Decadal to centennial productivity variability and NAO
Today, meteorology at KKJ is correlated with the predominant mode of the NAO (Fig. 11). The NAO is the major source of atmospheric circulation variability over the North Atlantic and Europe, primarily during winter (Hurrell, 1995). During its positive phase Scandinavian winter climate is characterized by above average temperatures, precipitation and windiness (Fig. 11) (Hurrell, 1995).
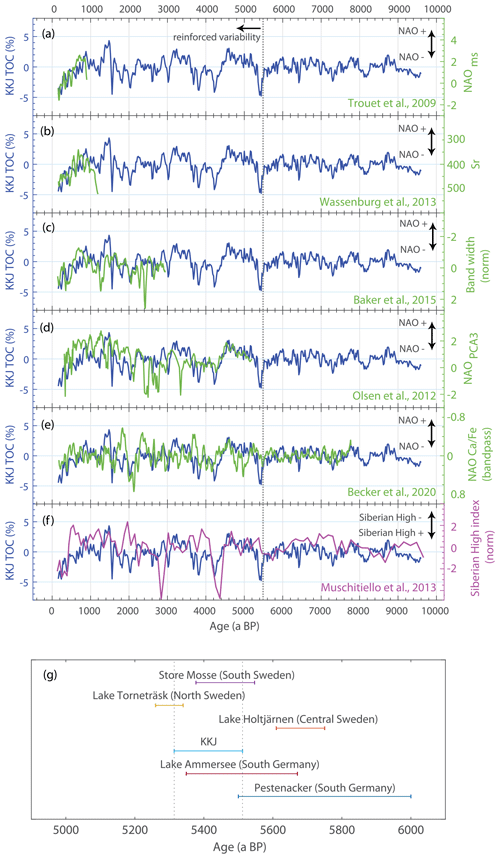
Figure 12Decadal to centennial paleoclimate records covering the last ∼ 9600 years. (a–e) Total organic carbon (TOC) record from Lake Kälksjön (KKJ) sediments with reconstructions of the North Atlantic Oscillation (NAO). (f) TOC record from KKJ with a proxy for Siberian High strength. Multi-millennial variability was removed from the KKJ TOC record by subtracting a 2500-year low-pass-filtered version from the original time series. (g) Timing of regime shifts in atmospheric circulation from north–central European paleoclimate records during the proposed reinforcement of NAO variability imprinted in KKJ sediments (Czymzik et al., 2013; Giesecke, 2005; Köhler et al., 2022; Kylander et al., 2013; Thienemann et al., 2018).
To investigate the preservation of decadal to centennial NAO polarity changes in KKJ sediments, we compare the TOC productivity record during SDUs 3 and 4 with reconstructions of the NAO from tree rings and speleothems for the Little Ice Age–Medieval Warm Period transition (Trouet et al., 2009; Wassenburg et al., 2013), speleothems from Scotland covering the last 3000 years (Baker et al., 2015), Greenland lake sediments back to 5200 a BP (Olsen et al., 2012), and marine sediments from off Norway back to 7800 a BP (Becker et al., 2020) (Fig. 12).
In particular, the NAO reconstructions by Olsen et al. (2012), Trouet et al. (2009), Wassenburg et al. (2013) and Baker et al. (2015) resemble most of the multi-decadal to centennial features in the TOC record from KKJ sediments (Fig. 12). Productivity in the lake tends to be higher, when the NAO is in a more positive mode (Fig. 12). The three latter NAO reconstructions also covary with the KKJ TOC record during the last ∼ 1200 years when the fit with the Olsen et al. (2012) NAO reconstruction is reduced. A possible reason for the differences between the KKJ TOC record and Olsen et al. (2012) NAO reconstruction during this period might be the nonstationary behavior of this atmospheric seesaw that is difficult to capture in individual archives, as well as its interplay with other modes of oceanic and atmospheric variability, like the eastern Atlantic–western Russia oscillation (Jung et al., 2003; Krichak and Alpert, 2005). Comparing our KKJ TOC record with the multi-millennial NAO reconstruction by Becker et al. (2020) reveals covariance most of the time, but also some inconsistencies around 1800, 2400 and 3700 a BP (Fig. 12). These inconsistencies might result from the proposed further influences of changes in the subpolar gyre and Atlantic Multi-decadal Oscillation on this marine NAO reconstruction (Becker et al., 2020).
A few sporadic negative peaks in the NAO records from Greenland lake sediments and Scottish speleothems that are not reflected in the TOC record from KKJ sediments might be explained by local climate or archive-specific noise (Fig. 12). Temporal inconsistencies of a few decades between the KKJ productivity record and NAO reconstructions are likely associated with the chronological uncertainties of, in particular, the 14C-dated sediment archives (Fig. 12).
Based on this comparison, we interpret our decadal to centennial TOC record from KKJ sediments during the complete 9612 () years to mainly reflect qualitative changes in NAO-like atmospheric circulation. Analogous to the millennial trend, the main mechanistic linkage for the observed decadal to centennial TOC variability in KKJ might be the influences of the NAO polarity on winter temperature, ice cover duration and lake productivity.
This interpretation is supported by meteorological studies and monitoring results from several Swedish lakes, indicating a significant influence of the NAO on annual to seasonal temperatures, ice cover duration and, consequently, productivity (Blenckner et al., 2004; Chen and Hellström, 1999; Hurrell, 1995). Winter temperatures in Sweden are warmer, ice cover is shortened and productivity higher when the NAO is in a positive mode. The importance of the NAO for ice cover duration can be exemplarily described by monitoring results from three lakes in the vicinity of KKJ (all 60∘ N in Sweden) covering the period 1961 to 2002 CE (Blenckner et al., 2004). Ice freeze on these lakes occurs from October to December, while ice break-up takes place from March to May, and the NAO is one significant driver of these major changes in ice cover duration (Blenckner et al., 2004; Ptak et al., 2019). In addition, NAO influences on ice cover duration of Swedish lakes are particularly strong south of 62∘ N where KKJ is located since the blocking of the North Atlantic zonal atmospheric circulation by the Scandinavian mountains is minor (Blenckner et al., 2004).
Further influences of changes in Siberian High (SH) strength on productivity in KKJ are possible, since the lake is situated at the western boundary of this atmospheric system. However, on the one hand, meteorological investigations and paleoclimate reconstructions indicate that NAO and Siberian High changes are interdependent, particularly on long timescales (Fig. 12). Siberian High strength tends to be reduced when the NAO is in a more positive mode (Chen et al., 2010; He et al., 2017). On the other hand, KKJ is located in the direct vicinity of the North Atlantic within the path of the westerly storm tracks. Therefore, considering the location of KKJ and intercontinental teleconnections, we prefer to relate the decadal to centennial changes in TOC as driven by NAO-like changes in atmospheric circulation.
Decadal to centennial productivity changes revealed by the KKJ sediment record indicate a shift towards reinforced variability concurrent with the onset of neoglaciation at ∼ 5450 cal a BP (Fig. 12). This shift in TOC is accompanied by the change from SDU 3 to 4 and from element cluster 3 to 4 (Fig. 4). It coincides with an increase in valley floor incision in the southern German Lech catchment (Köhler et al., 2022) and the onset of flood layer deposition in the sediment record from the pre-alpine Ammersee (Czymzik et al., 2013). It is broadly synchronous with a depletion of deuterium isotopes in Lake Torneträsk sediments (northern Sweden) and changing pollen assemblages in sediments of two lakes from central Sweden (Giesecke, 2005; Thienemann et al., 2018) (Fig. 12). All changes in the latter five central–northern European proxy records were interpreted to reflect a regime shift in atmospheric circulation. The two pollen records further rule out an anthropogenic origin of the signal (Giesecke, 2005). In addition, the recorded change in TOC variability coincides with the onset of reinforced shorter-term variability in an isotope record from Lake Bjärsträsk located east of KKJ (Gotland Island), interpreted to reflect Siberian High strength (Muschitiello et al., 2013), wind-driven dust input into the Store Mosse bog (southern Sweden) (Kylander et al., 2013) (Fig. 12) and lake level changes in Lake Bysjön (southern Sweden) (Digerfeldt, 1988).
Based on the interpretation of TOC variability in KKJ sediments as predominantly driven by NAO polarity changes on decadal to centennial scales, we hypothesize the described European climate shift at ∼ 5450 cal a BP to also be associated with a reinforcement of NAO-like atmospheric circulation variability. The proposed trigger of strengthened NAO polarity changes is more variable North Atlantic deep-water formation (Olsen et al., 2012; Trouet et al., 2009). This is supported by a record of Holocene North Atlantic deep-water formation deduced from δ13C values of benthic foraminifera in a marine sediment core, revealing strengthened multi-decadal variability since ∼ 5450 a BP (Repschläger et al., 2015).
Holocene sediments from KKJ provided insights into the stages and timing of lake evolution associated with postglacial landscape evolution, human interferences and climate variability in west–central Sweden. Following the isolation from ancient Lake Vänern through isostatic rebound at 9612 () cal a BP, varying TOC contents in KKJ sediments are interpreted to predominantly reflect changes in in-lake productivity modulated by the influences of winter temperature variability on ice cover duration and growth season length. An exception is the period from 1878 CE until today, in which sedimentation in KKJ has been dominated by anthropogenic lake level lowering and land use. Productivity increases in KKJ sediments are likely driven by the progressive millennial-scale winter warming in northwestern Europe, following the increasing Northern Hemisphere winter insolation, and decadal to centennial periods of a more positive NAO polarity. Strengthened productivity variability since ∼ 5450 cal a BP is hypothesized to reflect a reinforcement of NAO-like atmospheric circulation, concurrent with the onset of more vigorous variations in North Atlantic deep-water formation. Our results reveal the importance of terrestrial Holocene paleoclimate records for disentangling the time-transgressive and nonlinear interplay of internal and external forcing on regional climate variability.
The original data from this study will be available at the PANGAEA data library (data archiving is currently ongoing and no DOI is available yet).
MC initiated the study, carried out microfacies analysis and led the writing. RT carried out XRF measurements and statistical analyses. BP and SP performed geochemical measurements. PF conducted the hydro-acoustic survey. MT performed pollen analyses. MM measured 137Cs and 241Am contents. MC, RT, BP, SP, PF, MT, MM, MJS, CKMN, AB and HWA contributed to the interpretation of the data and writing of the final paper.
The contact author has declared that none of the authors has any competing interests.
Publisher's note: Copernicus Publications remains neutral with regard to jurisdictional claims in published maps and institutional affiliations.
We thank Brian Brademann (GFZ) and Hendrick Mück (IOW) for their help during the coring campaign at Lake Kälksjön. Michael Köhler (MKfactory) produced thin sections. Markus Czymzik is financed through grant CZ 227/4-1 (SyncBalt project) of the German Science Foundation (DFG). This publication is a contribution to the BaltRap project SAW-2017-IOW-2, funded by the Leibniz Association.
This research has been supported by the Deutsche Forschungsgemeinschaft (grant no. CZ 227/4-1) and the Leibniz-Gemeinschaft (grant no. SAW-2017-IOW-2).
The publication of this article was funded by the Open Access Fund of the Leibniz Association.
This paper was edited by Denis-Didier Rousseau and reviewed by Stefan Wastegård and one anonymous referee.
Aitchison, J.: The statistical analysis of compositional data, J. R. Stat. Soc. B, 44, 139–160, https://doi.org/10.1111/j.2517-6161.1982.tb01195.x, 1982.
Aitchison, J.: The statistical analysis of compositional data, Monographs, Chapman and Hall, London, ISBN 4122806046, 1986.
Andrén, E., Andrén, T., and Kunzendorf, H.: Holocene history of the Baltic Sea as a background for assessing records of human impact in the sediments of the Gotland Basin, Holocene, 10, 687–702, https://doi.org/10.1191/09596830094944, 2000.
BACC II: Second assessment of climate change for the Baltic Sea basin, Springer, Heidelberg, ISBN 978-3-319-36883-2, 2015.
Baker, A., C. Hellstrom, J., Kelly, B. F. J., Mariethoz, G., and Trouet, V.: A composite annual-resolution stalagmite record of North Atlantic climate over the last three millennia, Sci. Rep.-UK, 5, 10307, https://doi.org/10.1038/srep10307, 2015.
Becker, L. W. M., Sejrup, H. P., Hjelstuen, B. O., Haflidason, H., Kjennbakken, H., and Werner, J. P.: Palaeo-productivity record from Norwegian Sea enables North Atlantic Oscillation (NAO) reconstruction for the last 8000 years, npj Clim. Atmos. Sci., 3, 42, https://doi.org/10.1038/s41612-020-00147-6, 2020.
Berglund, M.: The Holocene shore displacement and chronology in Ångermanland, eastern Sweden, the Scandinavian glacio-isostatic uplift centre, Boreas, 33, 48–60, https://doi.org/10.1002/jqs.928, 2004.
Björck, S.: A review of the history of the Baltic Sea, 13.0–8.0 ka BP, Quatern. Int., 27, 19–40, https://doi.org/10.1016/1040-6182(94)00057-C, 1995.
Blenckner, T., Järvinen, M., and Weyhenmeyer, G. A.: Atmospheric circulation and its impact on ice phenology in Scandinavia, Boreal Environ. Res., 9, 371–380, 2004.
Bronk Ramsey, C.: Deposition models for chronological records, Quaternary Sci. Rev., 27, 42–60, https://doi.org/10.1016/j.quascirev.2007.01.019, 2008.
Caress, D. W. and Chayes, D. N.: Improved processing of hydrosweep DS multibeam data on the R/V Maurice Ewing, Mar. Geophys. Res., 18, 631–650, https://doi.org/10.1007/BF00313878, 1996.
Chen, D. and Hellström, C.: The influence of the North Atlantic Oscillation on the regional temperature variability in Sweden: spatial and temporal variations, Tellus A, 51, 505–516, https://doi.org/10.3402/tellusa.v51i4.14086, 1999.
Chen, H. F., Song, S. R., Lee, T. Q., Löwemark, L., Chi, Z., Wang, Y., and Hong, E.: A multiproxy lake record from Inner Mongolia displays a late Holocene teleconnection between Central Asian and North Atlantic climates, Quatern. Int., 227, 170–182, https://doi.org/10.1016/j.quaint.2010.03.005, 2010.
Croudace, I. W., Löwemark, L., Tjallingii, R., and Zolitschka, B.: Current perspectives on the capabilities of high resolution XRF core scanners, Quatern. Int., 514, 5–15, https://doi.org/10.1016/j.quaint.2019.04.002, 2019.
Czymzik, M., Dulski, P., Plessen, B., Von Grafenstein, U., Naumann, R., and Brauer, A.: A 450 year record of spring-summer flood layers in annually laminated sediments from Lake Ammersee (southern Germany), Water Resour. Res., 46, W11528, https://doi.org/10.1029/2009WR008360, 2010.
Czymzik, M., Brauer, A., Dulski, P., Plessen, B., Naumann, R., von Grafenstein, U., and Scheffler, R.: Orbital and solar forcing of shifts in Mid- to Late Holocene flood intensity from varved sediments of pre-alpine Lake Ammersee (southern Germany), Quaternary Sci. Rev., 61, 96–110, https://doi.org/10.1016/j.quascirev.2012.11.010, 2013.
Czymzik, M., Haltia, E., Saarni, S., Saarinen, T., and Brauer, A.: Differential North Atlantic control of winter hydroclimate in late Holocene varved sediments of Lake Kortejärvi, eastern Finland, Boreas, 47, 926–937, https://doi.org/10.1111/bor.12315, 2018.
Davis, B. A. S., Brewer, S., Stevenson, A. C., and Guiot, J.: The temperature of Europe during the Holocene reconstructed from pollen data, Quaternary Sci. Rev., 22, 1701–1716, https://doi.org/10.1016/S0277-3791(03)00173-2, 2003.
Digerfeldt, G.: Reconstruction and regional correlation of Holocene lake-level fluctuations in Lake Bysjön, South Sweden, Boreas, 17, 165–182, https://doi.org/10.1111/j.1502-3885.1988.tb00544.x, 1988.
Eddudóttir, S. D., Svensson, E., Nilsson, S., Ekblom, A., Lindholm, K.-J., and Johansson, A.: The history of settlement and agrarian land use in a boreal forest in Värmland, Sweden, new evidence from pollen analysis, Veg. Hist. Archaeobot., 30, 759–771, https://doi.org/10.1007/s00334-021-00829-y, 2021.
Fægri, K. and Iversen, J.: Textbook of Pollen Analysis, John Wiley and Sons, Chichester, ISBN 9781930665019, 1989.
Giesecke, T.: Holocene dynamics of the southern boreal forest in Sweden, Holocene, 15, 858–872, https://doi.org/10.1191/0959683605hl859ra, 2005.
Hammarlund, D., Björck, S., Buchardt, B., Israelson, C., and Thomsen, C. T.: Rapid hydrological changes during the Holocene revealed by stable isotope records of lacustrine carbonates from Lake Igelsjön, southern Sweden, Quaternary Sci. Rev., 22, 353–370, https://doi.org/10.1016/S0277-3791(02)00091-4, 2003.
He, S., Gao, Y., Li, F., Wang, H., and He, Y.: Impact of Arctic Oscillation on the East Asian climate: A review, Earth-Sci. Rev., 164, 48–62, https://doi.org/10.1016/j.earscirev.2016.10.014, 2017.
Hersbach, H., Bell, B., Berrisford, P., Hirahara, S., Horányi, A., Muñoz-Sabater, J., Nicolas, J., Peubey, C., Radu, R., Schepers, D., Simmons, A., Soci, C., Abdalla, S., Abellan, X., Balsamo, G., Bechtold, P., Biavati, G., Bidlot, J., Bonavita, M., De Chiara, G., Dahlgren, P., Dee, D., Diamantakis, M., Dragani, R., Flemming, J., Forbes, R., Fuentes, M., Geer, A., Haimberger, L., Healy, S., Hogan, R. J., Hólm, E., Janisková, M., Keeley, S., Laloyaux, P., Lopez, P., Lupu, C., Radnoti, G., de Rosnay, P., Rozum, I., Vamborg, F., Villaume, S., and Thépaut, J. N.: The ERA5 global reanalysis, Q. J. Roy. Meteor. Soc., 146, 1999–2049, https://doi.org/10.1002/qj.3803, 2020.
Hurrell, J. W.: Decadal trends in the North Atlantic Oscillation: Regional temperatures and precipitation, Science, 269, 676–679, https://doi.org/10.1126/science.269.5224.676, 1995.
Jones, P. D., Jonsson, T., and Wheeler, D.: Extension to the North Atlantic Oscillation using early instrumental pressure observations from Gibraltar and south-west Iceland, Int. J. Climatol., 17, 1433–1450, https://doi.org/10.1002/(SICI)1097-0088(19971115)17:13<1433::AID-JOC203>3.0.CO;2-P, 1997.
Jung, T., Hilmer, M., Ruprecht, E., Kleppek, S., Gulev, S. K., and Zolina, O.: Characteristics of the recent eastward shift of interannual NAO variability, J. Climate, 16, 3371–3382, https://doi.org/10.1175/1520-0442(2003)016<3371:COTRES>2.0.CO;2, 2003.
Karlsson, J., Jonsson, A., and Jansson, M.: Productivity of high-latitude lakes: Climate effect inferred from altitude gradient, Glob. Change Biol., 11, 710–715, https://doi.org/10.1111/j.1365-2486.2005.00945.x, 2005.
Köhler, A., Wanger-O'Neill, A., Rabiger-Völlmer, J., Herzig, F., Schneider, B., Nebel, S., Werban, U., Pohle, M., Kreck, M., Dietrich, P., Werther, L., Gronenborn, D., Berg, S., and Zielhofer, C.: A hydrological tipping point and onset of Neolithic wetland occupation in Pestenacker (Lech catchment, S Germany), Quaternary Sci. Rev., 278, 107370, https://doi.org/10.1016/j.quascirev.2022.107370, 2022.
Krichak, S. O. and Alpert, P.: Decadal trends in the east Atlantic-west Russia pattern and Mediterranean precipitation, Int. J. Climatol., 25, 183–192, https://doi.org/10.1002/joc.1124, 2005.
Kylander, M. E., Bindler, R., Cortizas, A. M., Gallagher, K., Mörth, C. M., and Rauch, S.: A novel geochemical approach to paleorecords of dust deposition and effective humidity: 8500 years of peat accumulation at Store Mosse (the “Great Bog”), Sweden, Quaternary Sci. Rev., 69, 69–82, https://doi.org/10.1016/j.quascirev.2013.02.010, 2013.
Labuhn, I., Hammarlund, D., Chapron, E., Czymzik, M., Dumoulin, J.-P., Nilsson, A., Edouard, R., Robygd, J., and von Grafenstein, U.: Holocene hydroclimate variability in Central Scandinavia inferred from flood layers in contourite drift deposits in Lake Storsjön, Quaternary, 1, 2, https://doi.org/10.3390/quat1010002, 2018.
Laskar, J., Robutel, P., Joutel, F., Gastineau, M., Correia, A. C. M., and Levrard, B.: A long-term numerical solution for the insolation quantities of the Earth, Astron. Astrophys., 428, 261–285, https://doi.org/10.1051/0004-6361:20041335, 2004.
Martin-Puertas, C., Tjallingii, R., Bloemsma, M., and Brauer, A.: Varved sediment responses to early Holocene climate and environmental changes in Lake Meerfelder Maar (Germany) obtained from multivariate analyses of micro X-ray fluoresence core scanning data, J. Quaternary Sci., 32, 427–436, https://doi.org/10.1002/jqs.2935, 2017.
Meyers, P. A.: Preservation of elemental and isotopic source identification of sedimentary organic matter, Chem. Geol., 114, 289–302, https://doi.org/10.1016/0009-2541(94)90059-0, 1994.
Meyers, P. A. and Teranes, J. L.: Sediment Organic Matter, in: Tracking Environmental Change Using Lake Sediments, Volume 2: Physical and Geochemical Methods, vol. 2, edited by: Last, W. M. and Smol, J. P., Kluwer Academic Publishers, Dordrecht, 239–269, ISBN 978-90-481-6021-1, 2001.
Moros, M., Andersen, T. J., Schulz-Bull, D., Häusler, K., Bunke, D., Snowball, I., Kotilainen, A., Zillén, L., Jensen, J. B., Kabel, K., Hand, I., Leipe, T., Lougheed, B. C., Wagner, B., and Arz, H. W.: Towards an event stratigraphy for Baltic Sea sediments deposited since AD 1900: approaches and challenges, Boreas, 46, 129–142, https://doi.org/10.1111/bor.12193, 2017.
Moros, M., Kotilainen, A. T., Snowball, I., Neumann, T., Perner, K., Meier, H. E. M., Leipe, T., Zillén, L., Sinninghe Damsté, J. S., and Schneider, R.: Is “deep-water formation” in the Baltic Sea a key to understanding seabed dynamics and ventilation changes over the past 7,000 years?, Quatern. Int., 550, 55–65, https://doi.org/10.1016/j.quaint.2020.03.031, 2020.
Muschitiello, F., Schwark, L., Wohlfarth, B., Sturm, C., and Hammarlund, D.: New evidence of Holocene atmospheric circulation dynamics based on lake sediments from southern Sweden: A link to the Siberian High, Quaternary Sci. Rev., 77, 113–124, https://doi.org/10.1016/j.quascirev.2013.07.026, 2013.
Olsen, J., John Anderson, N., and Knudsen, M. F.: Variability of the North Atlantic Oscillation over the past 5,200 years, Nat. Geosci., 5, 808–812, https://doi.org/10.1038/ngeo1589, 2012.
Pennington, W., Tutin, T. G., Cambray, R. S., and Fisher, E. M.: Observations on lake sediments using fallout 137Cs as a tracer, Nature, 242, 324–326, 1973.
Povinec, P. P., Bailly Du Bois, P., Kershaw, P. J., Nies, H., and Scotto, P.: Temporal and spatial trends in the distribution of 137Cs in surface waters of Northern European Seas – a record of 40 years of investigations, Deep.-Sea Res. Pt. II, 50, 2785–2801, https://doi.org/10.1016/S0967-0645(03)00148-6, 2003.
Ptak, M., Tomczyk, A. M., Wrzesiński, D., and Bednorz, E.: Effect of teleconnection patterns on ice conditions in lakes in lowland Poland, Theor. Appl. Climatol., 138, 1961–1969, https://doi.org/10.1007/s00704-019-02929-2, 2019.
Randsalu-Wendrup, L., Conley, D. J., Carstensen, J., Snowball, I., Jessen, C., and Fritz, S. C.: Ecological regime shifts in Lake Kälksjön, Sweden, in response to abrupt climate change around the 8.2 ka cooling event, Ecosystems, 15, 1336–1350, https://doi.org/10.1007/s10021-012-9588-1, 2012.
Reimer, P. J., Austin, W. E. N., Bard, E., Bayliss, A., Blackwell, P. G., Bronk Ramsey, C., Butzin, M., Cheng, H., Edwards, R. L., Friedrich, M., Grootes, P. M., Guilderson, T. P., Hajdas, I., Heaton, T. J., Hogg, A. G., Hughen, K. A., Kromer, B., Manning, S. W., Muscheler, R., Palmer, J. G., Pearson, C., van der Plicht, J., Reimer, R. W., Richards, D. A., Scott, E. M., Southon, J. R., Turney, C. S. M., Wacker, L., Adolphi, F., Büntgen, U., Capano, M., Fahrni, S. M., Fogtmann-Schulz, A., Friedrich, R., Köhler, P., Kudsk, S., Miyake, F., Olsen, J., Reinig, F., Sakamoto, M., Sookdeo, A., and Talamo, S.: The IntCal20 Northern Hemisphere radiocarbon age calibration curve (0–55 cal kBP), Radiocarbon, 62, 725–757, https://doi.org/10.1017/rdc.2020.41, 2020.
Repschläger, J., Weinelt, M., Kinkel, H., Andersen, N., Garbe-Schönberg, D., and Schwab, C.: Response of the subtropical North Atlantic surface hydrography on deglacial and Holocene AMOC changes, Paleoceanography, 30, 456–476, https://doi.org/10.1002/2014PA002637, 2015.
Risberg, J., Sandgren, P., and Andrén, E.: Early Holocene shore displacement and evidence of irregular isostatic uplift northwest of Lake Vänern, western Sweden, J. Paleolimnol., 15, 47–63, 1996.
Snowball, I., Sandgren, P., and Petterson, G.: The mineral magnetic properties of an annually laminated Holocene lake-sediment sequence in northern Sweden, Holocene, 9, 353–362, https://doi.org/10.1191/095968399670520633, 1999.
Snowball, I., Muscheler, R., Zillén, L., Sandgren, P., Stanton, T., and Ljung, K.: Radiocarbon wiggle matching of Swedish lake varves reveals asynchronous climate changes around the 8.2 kyr cold event, Boreas, 39, 720–733, https://doi.org/10.1111/j.1502-3885.2010.00167.x, 2010.
Stanton, T., Snowball, I., Zillén, L., and Wastegård, S.: Validating a Swedish varve chronology using radiocarbon, palaeomagnetic secular variation, lead pollution history and statistical correlation, Quat. Geochronol., 5, 611–624, https://doi.org/10.1016/j.quageo.2010.03.004, 2010.
Stanton, T., Nilsson, A., Snowball, I., and Muscheler, R.: Assessing the reliability of Holocene relative palaeointensity estimates: A case study from Swedish varved lake sediments, Geophys. J. Int., 187, 1195–1214, https://doi.org/10.1111/j.1365-246X.2011.05049.x, 2011.
Stockwell, J. W.: The CWP/SU: Seismic Un*x package, Comput. Geosci., 25, 415–419, 1999.
Stuiver, M.: Climate versus changes in 13C content of the organic component of lake sediments during the Late Quarternary, Quaternary Res., 5, 251–262, 1975.
Teranes, J. L. and Bernasconi, S. M.: Factors controlling δ13C values of sedimentary carbon in hypertrophic Baldeggersee, Switzerland, and implications for interpreting isotope excursions in lake sedimentary records, Limnol. Oceanogr., 50, 914–922, https://doi.org/10.4319/lo.2005.50.3.0914, 2005.
Thienemann, M., Kusch, S., Vogel, H., Ritter, B., Schefu?, E., and Rethemeyer, J.: Neoglacial transition of atmospheric circulation patterns over Fennoscandia recorded in Holocene Lake Torneträsk sediments, Boreas, 48, 287–298, https://doi.org/10.1111/bor.12365, 2018.
Tiljander, M., Saarnisto, M., Ojala, A. E. K., and Saarinen, T.: A 3000-year palaeoenvironmental record from annually laminated sediment of Lake Korttajärvi, central Finland, Boreas, 32, 566–577, https://doi.org/10.1111/j.1502-3885.2003.tb01236.x, 2003.
Trouet, V., Esper, J., Graham, N. E., Baker, A., Scourse, J. D., and Frank, D. C.: Persistent positive North Atlantic Oscillation mode dominated the Medieval Climate Anomaly, Science, 324, 78–80, https://doi.org/10.1126/science.1166349, 2009.
Uvo, C. B.: Analysis and regionalization of northern European winter precipitation based on its relationship with the North Atlantic Oscillation, Int. J. Climatol., 23, 1185–1194, https://doi.org/10.1002/joc.930, 2003.
Wanner, H., Beer, J., Butikofer, J., Crowley, T. J., Cubasch, U., Fluckiger, J., Goosse, H., Grosjean, M., Joos, F., Kaplan, J. O., Kuttel, M., Muller, S. A., Prentice, I. C., Solomina, O., Stocker, T. F., Tarasov, P., Wagner, M., Widmann, M., Bütikofer, J., Crowley, T. J., Cubasch, U., Flückiger, J., Goosse, H., Grosjean, M., Joos, F., Kaplan, J. O., Küttel, M., Müller, S. a., Colin Prentice, I., Solomina, O., Stocker, T. F., Tarasov, P., Wagner, M., and Widmann, M.: Mid- to Late Holocene climate change: an overview, Quaternary Sci. Rev., 27, 1791–1828, https://doi.org/10.1016/j.quascirev.2008.06.013, 2008.
Warden, L., Moros, M., Neumann, T., Shennan, S., Timpson, A., Manning, K., Sollai, M., Wacker, L., Perner, K., Häusler, K., Leipe, T., Zillén, L., Kotilainen, A., Jansen, E., Schneider, R. R., Oeberst, R., Arz, H., and Sinninghe Damsté, J. S.: Climate induced human demographic and cultural change in northern Europe during the mid-Holocene, Sci. Rep.-UK, 7, 15251, https://doi.org/10.1038/s41598-017-14353-5, 2017.
Wassenburg, J. A., Immenhauser, A., Richter, D. K., Niedermayr, A., Riechelmann, S., Fietzke, J., Scholz, D., Jochum, K. P., Fohlmeister, J., Schröder-Ritzrau, A., Sabaoui, A., Riechelmann, D. F. C., Schneider, L., and Esper, J.: Moroccan speleothem and tree ring records suggest a variable positive state of the North Atlantic Oscillation during the Medieval Warm Period, Earth Planet. Sc. Lett., 375, 291–302, https://doi.org/10.1016/j.epsl.2013.05.048, 2013.
Weltje, G. J., Bloemsma, M. R., Tjallingii, R., Heslop, D., Röhl, U., and Croudace, I. W.: Prediction of geochemical composition from XRF core scanner data: A new multivariate approach including automatic selection of calibration samples and quantification of uncertainties, in: micro-XRF studies of sediment cores. Developments in Paleoenvironmental Research, vol 17, edited by: Croudace, I. and Rothwell, R., Springer, Dordrecht, 507–534, https://doi.org/10.1007/978-94-017-9849-5_21, 2015.
Willemse, N. W. and Törnqvist, T. E.: Holocene century-scale temperature variability from West Greenland lake records, Geology, 27, 580–584, 1999.
Zillén, L., Snowball, I., Sandgren, P., and Stanton, T.: Occurrence of varved lake sediment sequences in Värmland, west central Sweden: Lake characteristics, varve chronology and AMS radiocarbon dating, Boreas, 32, 612–626, https://doi.org/10.1111/j.1502-3885.2003.tb01239.x, 2003.
Zillén, L., Conley, D. J., Andrén, T., Andrén, E., and Björck, S.: Past occurrences of hypoxia in the Baltic Sea and the role of climate variability, environmental change and human impact, Earth-Sci. Rev., 91, 77–92, https://doi.org/10.1016/j.earscirev.2008.10.001, 2008.
Zolitschka, B., Francus, P., Ojala, A. E. K., and Schimmelmann, A.: Varves in lake sediments – a review, Quaternary Sci. Rev., 117, 1–41, https://doi.org/10.1016/j.quascirev.2015.03.019, 2015.