the Creative Commons Attribution 4.0 License.
the Creative Commons Attribution 4.0 License.
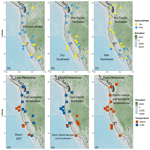
Holocene climate and oceanography of the coastal Western United States and California Current System
Veronica Padilla Vriesman
Caitlin M. Livsey
Carina R. Fish
Tessa M. Hill
Examination of climatic and oceanographic changes through the Holocene (11.75 ka–present) allows for an improved understanding and contextualization of modern climate change. Climate records of the Holocene can be utilized as a “baseline” from which to compare modern climate and can also provide insights into how environments experience and recover from change. However, individual studies on Holocene climate in the literature tend to focus on a distinct geographic location, a specific proxy record, or a certain aspect of climate (e.g., upwelling or precipitation), resulting in localized, record-specific trends rather than a comprehensive view of climate variability through the Holocene. Here we synthesize the major oceanographic and terrestrial changes that have occurred in the Western United States (bounded by 30 to 52∘ N and 115 to 130∘ W) through the most recent 11.75 kyr and explore the impacts of these changes on marine and terrestrial ecosystems. We present a novel spatiotemporal analysis of Holocene marine and terrestrial temperature, hydroclimate, and fire activity across the Early, Middle, and Late Holocene using a coded analysis of over 40 published studies. Following coded analysis of temperature, hydroclimate, and fire activity in the paper, we include a broader literature review of environmental change through the Holocene, including an examination of the impacts of multi-millennial climate trends on ecological communities. We find that the Early Holocene is characterized by warming relative to pre-Holocene conditions, including warm sea surface conditions, a warm and dry Pacific Northwest, a warm and wet Southwest, and overall spatial and temporal stability. In the Middle Holocene, these patterns reverse; this interval is characterized by cool sea surface temperatures, a cool and wet Pacific Northwest and warm and dry Southwest. The Late Holocene is the most variable interval, both spatially and temporally, and a novel spatial trend appears in terrestrial climate with warmer coastal areas and cooler inland areas. Human communities interacted with the environment throughout the entire Holocene, as evidenced in archeological and paleoenvironmental records, yet the recent Era of Colonization (1850–present) represents an unprecedented environmental interval in many records. Broadly, our analysis shows linkages between terrestrial and oceanographic conditions, distinct environmental phases through time, and emphasizes the importance of local factors in controlling climate through the dynamic Holocene.
- Article
(7664 KB) - Full-text XML
- BibTeX
- EndNote
1.1 Overview of Holocene climate
In contrast to past intervals of glacial/interglacial variability, the Holocene has largely been regarded as a climatically stable interval. However, local and global changes in Holocene climate merit further investigation. Meta-analysis of global temperatures and patterns through the Holocene show a globally warm period from 11 to 5 ka, termed the Holocene Climatic Optimum, largely driven by a concurrent peak in summer solar insolation in the Northern Hemisphere (Mayewski et al., 2004; Wanner et al., 2008; Renssen et al., 2012; Marcott et al., 2013; Bader et al., 2020). Following this period of global warmth, competing lines of evidence show divergent global temperature regimes in the last 5 kyr (some records show global warming and others global cooling); this phenomenon is termed the Holocene Conundrum (Wanner et al., 2008; Marcott et al., 2013; Liu et al., 2014; Marsicek et al., 2018; Bader et al., 2020). Currently available global reconstructions typically under-sample temperature records from the North Pacific and western North America, motivating the need for further analysis of Holocene change over time in this region (Marcott et al., 2013; Kaufman et al., 2020; Praetorius et al., 2020; Walczak et al., 2020).
Holocene climate variability on various timescales has been attributed to multiple climate forcing mechanisms, including changes in solar insolation, volcanic activity, land-use change, variations in atmospheric greenhouse gas concentrations, and climate feedbacks (Crowley, 2000; Bradley, 2003; Atwood et al., 2016). Specifically, Northern Hemisphere summer insolation was at a Holocene maximum and winter insolation was at a Holocene minimum at 9 ka, with important implications for global and regional climate (Renssen et al., 2012; Marcott et al., 2013; Marsicek et al., 2018; Swain et al., 2018; Routson et al., 2019; Bader et al., 2020; Kaufman et al., 2020). In the Early Holocene, peak summer insolation and continued melting of Northern Hemisphere ice sheets are dominant drivers of North American climate; following the collapse of the Laurentide Ice Sheet, the impact of ice sheet feedbacks on climate is reduced (Viau et al., 2006; Renssen et al., 2012; Bader et al., 2020). In North America broadly, mean summer temperatures increased from the last glacial maximum (26–19 ka) (Clark et al., 2009; Waelbroeck et al., 2009) until between 6 and 3 ka by ∼4 ∘C and subsequently decreased (Viau et al., 2006). Across the continent, millennial-scale climate variability on the order of 0.2 ∘C occurs across the entire Holocene (Viau et al., 2006). Analysis of global and continental records show a high degree of temporal and spatial climate variability highlighting the need to assess region-specific changes through time. Understanding regional Holocene variability is particularly relevant to contextualize biotic and environmental responses to anthropogenic climate change.
1.2 Modern climate and oceanography of western North America
Western North America currently experiences impacts of drought, fire, heatwaves, and fisheries, agriculture, and ecological shifts due to climate change (e.g., Ainsworth et al., 2011; Mann and Gleick, 2015; Westerling et al., 2011; Wise, 2016). Quantifying climatic and environmental changes over time in this region is of particular importance as it hosts a large modern human population, a multitude of natural resources, and highly biodiverse and productive ecosystems. By investigating climate and environmental change through the Holocene, we can better understand how environments and ecosystems that are climatically and tectonically similar to the present experience and recover from environmental change (e.g., Barnosky et al., 2017; Finnegan et al., 2015). For the purposes of this review, we divide the region into the Pacific Northwest (abbreviated as PNW; including Washington, Oregon, and Northern California) and Southwest (abbreviated as SW; including Nevada, Arizona and Central/Southern California) (Fig. 1).
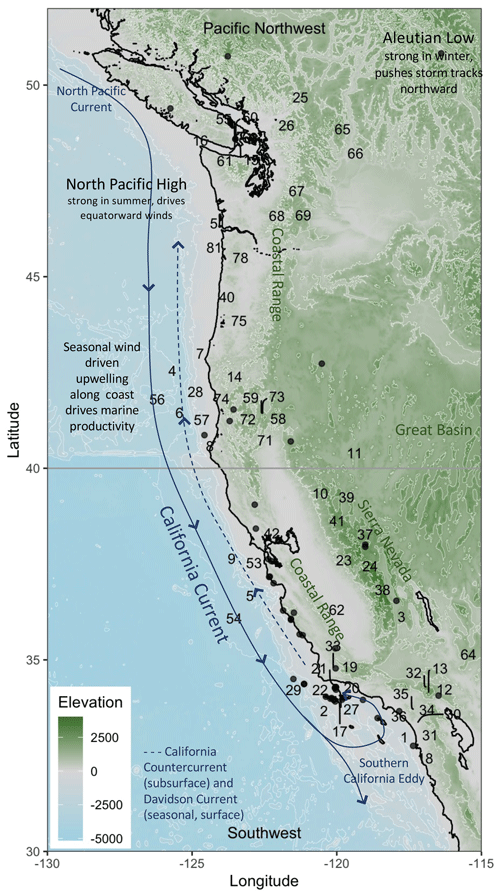
Figure 1Map of locations of all studies reviewed in the paper with simplified schematic of modern oceanographic and hydrographic conditions. Sites used in spatiotemporal analysis are numbered; numbers correspond to papers listed in Appendix A. Sites used in literature review are included as black dots. Gray horizontal line shows delineation between Pacific Northwest and Southwest for the purposes of this study.
In the modern system, the interaction between the Aleutian Low pressure system, the North Pacific High pressure system, and the continental thermal low pressure system drives temperature and hydrographic gradients (Fig. 1). Modern terrestrial climate of the Western United States varies latitudinally with cooler temperatures and wintertime precipitation in the PNW and warmer temperatures, general aridity, and relatively reduced precipitation in the SW (Fig. 1) (e.g., Sheppard et al., 2002; Adams and Comrie, 1997; Barlow et al., 1998). Hydroclimate variability is typically characterized by a north–south dipole pattern of precipitation anomalies with opposing signs and less often by a west–east pattern (e.g., Wise, 2016, 2010). Atmospheric rivers are a major source of hydrologic input and variability in this region (e.g., Dettinger et al., 2011). Drought is a recurrent and salient feature of climate in the west, particularly in the context of anthropogenic climate change (Fig. 1) (e.g., Cook, 2004; Wise, 2016). Wildfire is also a dominant feature of the landscape of the Western United States; climate, vegetation/fuel availability, and human activity interact as drivers of fire activity (e.g., Marlon et al., 2012; Westerling et al., 2003, 2011; Higuera et al., 2021; Dennison et al., 2014; Shuman et al., 2022). Variability in precipitation, air temperature, and fire regime exert strong control over plant and animal communities (e.g., Beaty and Taylor, 2009; Clark et al., 2012; Marlon et al., 2006; Shuman et al., 2018; Dingemans et al., 2014).
Marine and terrestrial climates are linked in this region; westerly winds influenced by North Pacific ocean–atmosphere dynamics result in high correlation between North Pacific sea surface temperatures (SSTs) and coastal air temperatures (e.g., LaDochy et al., 2007; Barron and Anderson, 2011). The westerly winds generated by the Aleutian Low pressure system and North Pacific High pressure system fuel the California Current System (CCS), which flows southward from ∼50 to N (Fig. 1) (e.g., Checkley and Barth, 2009; Hickey, 1979). The CCS is characterized by strong seasonal wind-driven upwelling that drives high surface productivity along western North America (e.g., Siedlecki et al., 2015; Hickey, 1979; Checkley and Barth, 2009). Respiration, remineralization, and decomposition lead to high organic carbon export and oxygen-depleted (CO2-rich) bottom waters (e.g., Checkley and Barth, 2009; Chelton et al., 1982; Siedlecki et al., 2015). The interaction of the equatorward California Current, the poleward Davidson Current, and the sub-surface, poleward California Undercurrent drives oceanographic conditions south of Point Conception (Bray et al., 1999). Seasonal, annual, and decadal events, including the Pacific Decadal Oscillation (PDO), North Pacific Gyre Oscillation, and El Niño–Southern Oscillation (ENSO), play a significant role in driving eastern North Pacific SST and productivity across multiple timescales (e.g., Chavez et al., 2003; Batchelder and Powell, 2002; Di Lorenzo et al., 2008; Lluch-Cota et al., 2001; Mantua et al., 2002). Changes in marine currents, upwelling regime, and temperature impact primary production, with cascading implications for the entire marine food web (Barron et al., 2003; Yati et al., 2020).
Climate and environmental changes through time impact human communities that have been present in western North America throughout the Holocene. Evidence from over 40 archeological sites in North America shows humans were likely present before, during, and after the Last Glacial Maximum (26.5–19 ka) (Becerra-Valdivia and Higham, 2020). Additional evidence for human presence and societal expansion within the geographic and temporal range of this review includes archeological evidence from shell middens as well as Indigenous oral histories (e.g., Braje et al., 2012; Erlandson et al., 2007, 2009; Kennett et al., 2007; McKechnie, 2015; Rick, 2011; Becerra-Valdivia and Higham, 2020). Previous work has investigated linkages between climate and human communities on a local scale through the Holocene (e.g., Braje et al., 2012; Erlandson et al., 2007; Glassow et al., 2012; Kennett et al., 2007; McKechnie, 2015; Rick, 2011).
Here, we conduct a spatiotemporal analysis of temperature, hydroclimate, and fire activity between the Early, Middle, and Late Holocene and synthesize previously published records of both marine and terrestrial systems from the Western United States and California Current System (Appendices A–C), resulting in the most comprehensive multi-proxy Holocene climate reconstruction for this region to date. This review addresses the following linked research questions: how do temperature, hydroclimate, and fire activity vary between the Early, Middle, and Late Holocene in the Western United States? How do marine and terrestrial environments interact in this region during the Holocene? How do climate and oceanographic changes impact ecological communities in the Holocene?
For the purposes of this paper we follow the standard convention formally recognized by the International Commission on Stratigraphy (Walker et al., 2018): Early Holocene (Greenlandian, 11.75–8.2 ka), Middle Holocene (Northgrippian, 8.2–4.2 ka), and Late Holocene (Meghalayan, 4.2–0 ka). We do include interpretations that span across these sub-epoch time intervals. We constrain our study to the geographic region bounded by 30 to 52∘ N and 115 to 130∘ W as this region has an abundance and variety of long-term records throughout the Holocene.
2.1 Spatiotemporal analysis
To conduct a spatiotemporal analysis of environmental change in this region, we identified studies using rigorous criteria, coded the results of previous studies through time, and visualized the results in a series of maps (Figs. 2, 3). Records were initially identified using the following topics as search terms: marine temperature, ocean circulation, marine productivity, terrestrial hydroclimate, drought, floods, terrestrial temperature, fire, marine ecology, and terrestrial ecology. For inclusion in the spatiotemporal analysis, previously published studies must be located within the defined geographic region (30 to 52∘ N and 115 to 130∘ W), have a published age model, must span at least 3000 years of the Holocene, and must present a clearly identified and described climatic pattern or patterns for an entire Holocene interval. Results from each paper were binned into time intervals: Early, Middle, and Late Holocene, and coded using the following categories: hydroclimate (wet or dry), temperature (warm or cold), and fire activity (high or low).
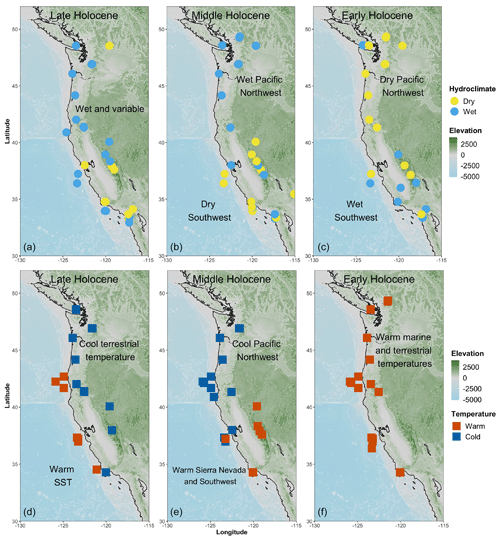
Figure 2Top panel shows hydroclimate records in Early (11.7–8.2 ka, c), Middle (8.2–4.2 ka, b), and Late (4.2–0 ka, a) Holocene: yellow dots represent dry conditions and light blue represents wet conditions. Bottom panel shows temperature records from Early (f), Middle (e), and Late (d) Holocene: dark blue squares represent cold temperatures and orange represents warm temperatures.
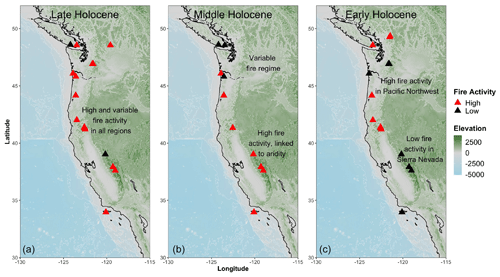
Figure 3Fire activity as reconstructed by charcoal records through the Early (11.7–8.2 ka, c), Middle (8.2–4.2 ka, b), and Late (4.2–0 ka, a) Holocene; red indicates high fire activity, and black indicates low fire activity. Multiple metrics for fire activity are used; interpretations of original authors are preserved.
Results were coded using keywords from the original authors' description of climate conditions at each point in time: temperature keywords include warm, cold, and cool; hydroclimate keywords include wet, dry, moisture, arid(ity), pluvial, fluvial, and precipitation; fire keywords include high, low, activity, frequency, return interval, and intensity. We generated a database that contains paper (author, date), latitude, longitude (of all sites within each paper), region (PNW or SW), archive type, type of chronology, number of age dates used in age model, and coded results for each category (hydroclimate, temperature, fire activity) in each interval (Early, Middle, Late). The spatiotemporal analysis includes results from 46 papers at 75 sites (Appendix A).
A wide range of archive types are represented here: dendrochronology, speleothem, lacustrine sediments, marine sediments, and other records including geomorphology, animal midden, and lichen archives. Interpretations in original papers are based on a variety of well-established proxies: alkenones, carbon and nitrogen isotopes of bulk sediments, charcoal, diatom and silicoflagellate assemblage, foraminiferal assemblage, geomorphology, hydrogen isotopes from leaf wax, oxygen and carbon isotopes of nearshore marine organisms, oxygen and carbon isotopes of biogenic and non-biogenic calcite, macrofossil abundance, pollen assemblage, radiocarbon dating, sedimentology, including grain size analysis, lithology, organic content, carbon content analysis, and tree ring/tree stump analysis.
This paper makes no attempt to evaluate the effectiveness of each proxy or to re-examine the primary data; rather, interpretations of data from original work are maintained. For example, changes in SST may be inferred from alkenones, foraminiferal assemblages, or foraminiferal isotope records in previously published studies, but all are represented as temperature in the coded database. It is important to note that some proxies are seasonally biased and thus represent only a portion of an overall climate/ecological trend. We utilize the term “fire activity” to be inclusive of multiple paleofire proxy types including fire return intervals, CHAR, charcoal accumulation, and others. Some papers contributed multiple data points to this step. For example, some records included interpretations of multiple processes (e.g., temperature and hydroclimate) and some include interpretations across multiple time intervals (Early, Middle, Late). Yet, papers that only included one interpretation in one interval are also included.
Results of coded analysis are plotted in space through the three time intervals (Early, Middle, Late Holocene) (Figs. 2, 3). Temperature (marine and terrestrial), hydroclimate, and fire activity are plotted separately (Figs. 2, 3). Base maps with elevation are generated using the R package marmap (Pante and Simon-Bouhet, 2013) accessing bathymetric and elevation data from the NOAA ETOPO1 global relief model (Amante and Eakins, 2009).
Following the spatial and temporal analysis, we highlight a subset of regionally representative time series records from the PNW (Fig. 4) and SW (Fig. 5). We prioritized continuous records and records that span at least two intervals of the Holocene (Kaufman et al., 2020). Further, we selected records that represent temperature, hydroclimate, and fire activity for each region (PNW and SW). For these purposes, data were accessed through original papers, supplemental information, or through the NOAA Paleoclimate Database (Appendix C).
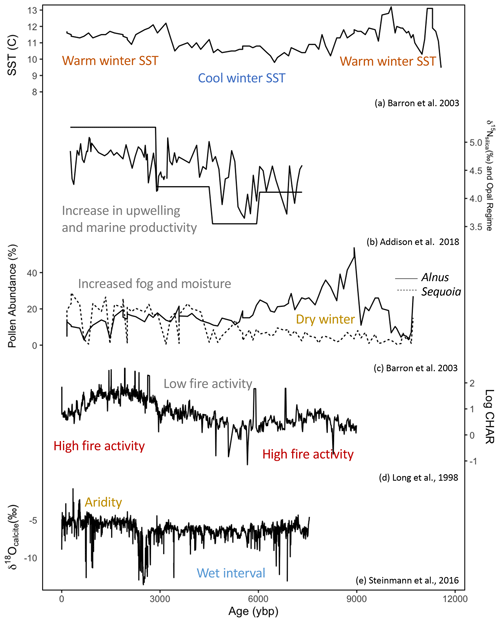
Figure 4Representative datasets from the Pacific Northwest. Panels from top to bottom alkenone sea surface temperature from core ODP 1019 (a, Barron et al., 2003). Bulk sediment δ15N and opal regime index from core TN062-O550 (b, Addison et al., 2018). Relative abundance of pollen: solid line is Alnus (alder) and dashed line is Sequoia (coastal redwood) from core ODP1019 (c, Barron et al., 2003). Log charcoal accumulation rate at Little Lake (d, Long et al., 1998). Lake sediment bulk calcite δ18O from Cleland Lake, Washington (e, Steinman et al., 2016).
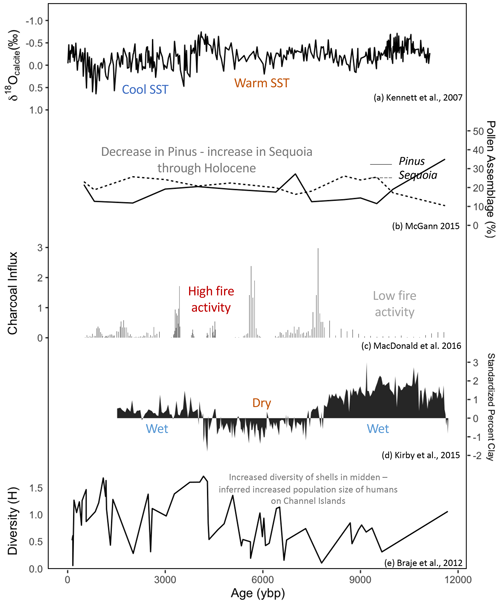
Figure 5Representative datasets from the Southwest. Oxygen isotope record from planktonic foraminifera G. bulloides from Santa Barbara Basin core ODP 893 (a, Kennett et al., 2007). Pollen assemblage (%) of Pinus and Sequoia from Central California marine sediment core (b, McGann 2015). Charcoal influx to Kirman Lake in the Sierra Nevada mountains (c, Macdonald et al., 2016). Standardized percent clay as a proxy for relative lake status for Silver Lake (d, Kirby et al., 2015). Diversity of shells from shell midden sites on Channel Islands (e, Braje et al., 2012).
2.2 Literature review
Following coded analysis of temperature, hydroclimate, and fire activity in the paper, we include a broader literature review of environmental change through the Holocene and a discussion of the impacts of climate changes to ecological communities (Appendix B). For inclusion in this step of the review, previous studies must be within the geographic range of the study and include a published age model. Papers identified in this step are discussed in the text in this article. The literature review includes 107 papers from 148 sites.
2.3 Chronological uncertainty
For this review, we rely on previously published chronologies. Each paper included here has 2–120 age dates (Appendix B). The mean number of age dates per record is 16.6 and the median is 12 (Appendix B). The most common methods of age dating were radiocarbon of organic material (68 sites) and radiocarbon of carbonate (46); tephrochronology, dendrochronology, oxygen isotope stratigraphy, varve chronology and others were less common (Appendix B). To accommodate age uncertainty and differences in age model development between papers, all interpretations utilized in the coded analysis and map visualizations (Figs. 2–3) must have been reported by original authors as a consistent trend across a full interval of the Holocene (>3 kyr). Thus, by identifying climatic codes at a multi-millennial scale, we reduce error due to variability in age model development between papers. In text and in the broader literature review, the timing of events uses the original authors published age dates. We encourage readers to reference Appendix B for additional information about age control. Importantly, timing of events such as the 8.2 ka event, Medieval Climate Anomaly, Little Ice Age, and Era of Colonization are based on the original authors' interpretations.
3.1 Early Holocene (11.75–8.2 ka)
Thirty-three papers encompassing 59 sites fit the criteria for inclusion in the spatiotemporal analysis. Archives included lake sediments (40 sites), marine sediments (15 sites), and two others (geomorphology, dendrochronology) (Appendix A, B).
3.1.1 Regional synthesis and marine–terrestrial interactions
Spatial analysis of coded hydroclimate, temperature, and fire history indicates that the Early Holocene was generally characterized by a dry and warm PNW and a wet and warm SW (Fig. 2). These trends are also observed in time series data from both regions (Figs. 4, 5). In the PNW, sea surface temperatures were warm, winters were dry, and fire activity was high (Fig. 4). In the SW, sea surface temperatures were warm, terrestrial temperatures were cold, fire activity was low, and hydroclimate was generally wet (Fig. 5).
Generalized warming in the Early Holocene relative to pre-Holocene conditions follows two abrupt Northern Hemisphere climate transitions, characterized by rapid warming during the Bølling–Allerød (14.7–12.9 ka) and rapid cooling associated with the Younger Dryas (12.9–11.7 ka) (Barron et al., 2003; McGann, 2015). Sea surface temperatures were warm across the entire coastal CCS during the Early Holocene (Fig. 2), likely driven by high summer insolation (insolation maximum at 9 ka) and warming following deglaciation. Despite warm SST across the region, terrestrial hydroclimate and temperatures varied latitudinally, indicating regional differences in marine–terrestrial climate linkages and impacts of the summer insolation maximum (Fig. 3). In the PNW, warm SSTs co-occurred with warm and dry terrestrial conditions (Heusser, 1998; Barron et al., 2003; Steinman et al., 2016), while in the SW, wet conditions were driven by a combination of more intense winter precipitation associated with reduced winter insolation and the evolution of the North American monsoon (NAM) and the resultant increase in summertime precipitation in the Early Holocene relative to pre-Holocene (Fig. 2) (Bird and Kirby, 2006; Kirby et al., 2015; Metcalfe, 2015).
3.1.2 Terrestrial climate
In the PNW, multiple records show warm and dry conditions in the Early Holocene (Fig. 2c, f) (Mohr et al., 2000; Hallett et al., 2003; Briles et al., 2005; Malamud-Roam et al., 2006; Marlon et al., 2006; Gavin et al., 2007; Long et al., 1998; Steinman et al., 2019). Drier than modern conditions existed in locations dominated by winter precipitation (largely PNW) (Fig. 2c) (McGann, 2011; Hermann et al., 2018; Leidelmeijer et al., 2021). An east–west precipitation gradient was established with warm and dry conditions in the east and wet conditions in the west (Brown and Hebda, 2002), fire activity was high at multiple locations (Hallett et al., 2003; Marlon et al., 2006; Gavin et al., 2007; Steinman et al., 2019), and the amount of biomass burning and the extent of tree cover increased during this time (Figs. 2, 3) (Marlon et al., 2006; Gavin et al., 2007). The Early Holocene increase in fire activity in the PNW relative to pre-Holocene conditions may have been caused by warmer/drier conditions resulting from amplified seasonality due to increased summer insolation relative to pre-Holocene (Walsh et al., 2017; Steinman et al., 2019). In some locations (i.e., Scanlon and Castor Lake), fire frequency increased relative to glacial but remained lower relative to the Middle or Late Holocene (Walsh et al., 2017). Few records show wet conditions in the PNW during the Early Holocene (McQuoid and Hobson, 2001).
In California's Central Valley and Sierra Nevada, the Early Holocene was wet with low or variable fire frequency (Figs. 2, 3) (Benson et al., 2002; Bacon et al., 2006; Negrini et al., 2006; Hallett and Anderson, 2010). Fuel abundance exerted a stronger control on fire than regional climate (Hallett and Anderson, 2010). In the Eastern Sierra Nevada (i.e., Lower Gaylor Lake and Barrett Lake near Mono Lake), low biomass (sparse pine dominated forests and chaparral shrubs) reinforced by low snowpack persistence due to the summer insolation maximum led to low fire frequency (Hallett and Anderson, 2010). In the Lake Tahoe basin, precipitation was high in the Early Holocene and fire frequency was low in the very Early Holocene (11–9 ka) and transitioned to higher fire frequency than modern from 9 ka through the Middle Holocene (Lindstrom, 1990; Benson et al., 2002; Beaty and Taylor, 2009).
In comparison to the arid PNW, Early Holocene conditions in the SW were largely wetter than modern (Fig. 2). Lacustrine records from across the SW show heavy precipitation in the Early Holocene (Bird and Kirby, 2006; Kirby et al., 2007, 2010, 2012, 2014, 2015; Bird et al., 2010; Du et al., 2018). Wet conditions at this time were driven by both winter precipitation and the North American monsoon (Bird and Kirby, 2006; Kirby et al., 2007; Marcott et al., 2013; Hermann et al., 2018). Prior to 8 ka, the trajectory of the North American monsoon was shifted west of its modern-day impact due to warm SST in the Gulf of California and the northern shift of the North Pacific High, resulting in some precipitation in Southern California (Barron et al., 2012). Yet monsoonal precipitation alone cannot explain the wet conditions of the Early Holocene (Kirby et al., 2007, 2012; Barron et al., 2012). The Early Holocene winter insolation minimum led to an increase in winter precipitation in Southern California (Bird and Kirby, 2006; Kirby et al., 2007; Marcott et al., 2013; Hermann et al., 2018). Atmospheric river-like storms may have played a key role in contributing to wet conditions at this time (Kirby et al., 2005, 2007, 2012; Bird et al., 2010). High moisture/precipitation is shown in both inland (desert) and coastal sites, including increased runoff into the Santa Barbara Basin from 9 to 8.5 ka and a pluvial episode synchronous across multiple sites in Southern California from 9.1 to 8.25 ka (Kirby et al., 2007, 2010, 2012, 2014; Du et al., 2018). In contrast, lake sediment records from Southern California that resolve summer evaporation show high evaporation and multiple intervals of drought in the Early Holocene; high winter precipitation and high summer evaporation may explain the differences between multiple records in this region (Kirby et al., 2019).
3.1.3 Marine conditions
Offshore of Southern Oregon through Central California, the Early Holocene was characterized by warm SSTs resulting from subtropical waters transported by currents comparable to the modern-day Davidson Current (Figs. 1, 2) (Mix et al., 1999; Barron et al., 2003; Barron and Bukry, 2007a). In this area, the oceanographic regime was characterized by overall warm conditions, moderate to high export productivity, ventilation of deeper waters, and the development of the oxygen minimum zone (Barron et al., 2003; McGann, 2011; Addison et al., 2018). During this time, the California Current was weaker than present allowing a strong northward Davidson-like current (Barron and Bukry, 2007a). Though upwelling was weaker than modern in the Early Holocene, records from offshore Oregon show moderate marine productivity and multiple records indicate an increase in coastal upwelling beginning at 9 ka (Gardner et al., 1988; Barron et al., 2003; Addison et al., 2018; Barron et al., 2018). Offshore Southern California (in Santa Barbara Basin), high productivity and overall warm SSTs occurred during the Early Holocene (Kennett et al., 2007; Fisler and Hendy, 2008). Records extending from Northern California south to the Baja California (Sur) peninsula indicate that in many locations, the onset of poorly oxygenated conditions in subsurface and intermediate waters occurred in the Early Holocene, indicating changes in surface water productivity, intermediate water ventilation, or changes in the strength or source of North Pacific Intermediate Water relative to pre-Holocene conditions (Kennett and Ingram, 1995; Mix et al., 1999; Roark et al., 2003; Moffitt et al., 2014).
3.1.4 8.2 ka event
The end of the Early Holocene is marked by a large-scale Northern Hemisphere cooling event initiated by an abrupt drainage of glacial lakes Agassiz and Ojibway into the North Atlantic that weakened Atlantic Meridional Overturning Circulation (Barber et al., 1999; Thomas et al., 2007; Matero et al., 2017; Estrella-Martínez et al., 2019; Voarintsoa et al., 2019). Though a globally well-described event, relatively few records (fewer than 10 records reviewed in this paper) from the northeastern Pacific and Western United States record impacts of the 8.2 ka event. In Central California (White Moon Cave), speleothem records show increased effective moisture at 8.2 ka which is attributed to intensification of the Pacific storm track that is synchronous on eastern and western sides of the Pacific (Oster et al., 2017). A lake record from Dry Lake in the SW (San Bernardino Mountains) shows a cooler, dryer, and enhanced erosional period during the 8.2 ka event (Bird and Kirby, 2006). Marine records from off the coast of Northern California (near the California–Oregon border) show a cold event at 8.2 ka, but this is not noted in other nearby marine records (Barron et al., 2003). We infer that the 8.2 ka event has a limited impact in this region, apart from the teleconnected impact of changes to the Pacific storm track impacting local hydroclimate.
3.1.5 Ecological responses to change
In the Early Holocene, terrestrial plant communities generally indicate warm conditions but show a process of succession out of glacial-associated ecosystems (Heusser, 1998). In the PNW, expansion of alder (Alnus) and ferns (Pteridium) began at the start of the Holocene, and Douglas fir (Pseudotsuga menziesii), western hemlock, grasses (Poaceae), and bracken fern (Pteridium) forests emerged by the end of the interval (Heusser, 1983; Pellatt et al., 2001). Southern Oregon cave records demonstrate taxonomic stability in plant communities through the Early Holocene with communities dominated by pine (Pinus) and sagebrushes (Amaranthaceae, Artemisia) (Beck et al., 2018). In the Klamath Mountains, pine and oak (Quercus) dominated the pollen record during the warm dry Early Holocene; similarly, pine, oak, and juniper dominated the record in the Siskiyou Mountains (Mohr et al., 2000; Briles et al., 2005). In Northern California, pollen assemblages indicate warm winters, including an expansion of coastal redwoods and declining alder (Alnus) forests (Barron et al., 2003; Lyle et al., 2012). The increasing upwelling during the Holocene led to enhanced coastal fog and, as a result, increasing dominance of coastal redwood (Sequoia sempervirens) (Gardner et al., 1988; Barron et al., 2003; McGann, 2015; Addison et al., 2018). In Central California, increases in redwoods, oaks, Nepenthes densiflora, and Asteraceae indicate warmer and wetter conditions relative to glacial (McGann, 2015). In Southern California, the Early Holocene maintained a forest ecosystem distinct from the modern with more pines and conifers than in the Late Holocene and modern (Heusser, 1978).
In marine systems off the coast of Northern California (Farallon Escarpment and offshore of the Russian River) transitions in planktic and benthic fauna occurred during the Early Holocene as environmental conditions transitioned out of the glacial (Gardner et al., 1988; McGann, 2011). From 11.75 to 10 ka marine sediment records show low abundance of the upwelling-associated diatoms Thalassionema nitzschioides and Thalassionema longissima, and dominance of a cool, subpolar foraminiferal assemblage (Gardner et al., 1988). By 10.5–9 ka, carbonate productivity and total carbon (organic and carbonate-bound carbon) peaked and warm water morphotypes of foraminifera were dominant across two Northern California marine sediment records during this spike in productivity (Gardner et al., 1988; McGann, 2011). In coastal systems, kelp forests were present along the entire coastline from the SW to the PNW and increased in both size and range during the Early Holocene (Erlandson et al., 2007; Graham et al., 2010). In deeper waters, the gradual development of the oxygen minimum zone in the Early Holocene led to a transformation of seafloor ecosystems to a low oxygen-adapted assemblage (Cannariato and Kennett, 1999; Moffitt et al., 2014; McGann, 2015). Off the coast of Point Conception, seafloor fauna (e.g., benthic foraminifera) were variable in the Early Holocene, with the establishment of the modern fauna by 9 ka (McGann, 2015).
3.2 Mid-Holocene (8.2–4.2 ka)
Thirty-three papers encompassing 58 sites met the criteria for spatiotemporal analysis in the Middle Holocene and were coded and plotted in Figs. 2 and 3. Archives included lake sediments (36 sites), marine sediments (18 sites), and three others (terrestrial midden, dendrochronology, and geomorphology) (Appendix A, B).
3.2.1 Regional synthesis and marine–terrestrial interactions
Spatial analysis of coded hydroclimate, temperature, and fire history indicates that the Middle Holocene was characterized by a wet and cool PNW and a dry and warm SW relative to modern conditions (Fig. 2). Fire activity was high in the Sierra Nevada mountains and variable and transitional in the PNW (Fig. 3). Time series analyses support these findings: offshore of the PNW, sea surface temperatures were cool, upwelling activity was moderate and terrestrial PNW winters were wet, fire activity was low and variable, and the overall hydroclimate was wet relative to the Early Holocene (Fig. 4). In the SW, sea surface temperatures were warm, fire activity was high, and hydroclimate was generally dry (Fig. 5).
Hydroclimate and temperature trends functionally reversed in the Middle Holocene relative to the Early Holocene, and the Middle Holocene was a time of transition and variability in many records (Figs. 4, 5). In the PNW, SST transitions from warm to cool temperatures coeval with a shift from warm and dry to cool and wet terrestrial conditions. These coincident transitions suggest that the mechanisms for marine–terrestrial climate linkages persisted through the Early and Middle Holocene, namely a negative correlation between marine temperatures and terrestrial moisture/precipitation (Fig. 2). Yet nuanced changes occurring on shorter timescales may play an important role in determining hydroclimate of the region. A previously published review of hydroclimate records in western North America (PNW, Northern Rockies and most of California) at 6 ka shows aridity in areas that are dominated by winter precipitation, including multi-decadal to centuries-long “mega droughts” due to reduced winter water vapor transport (Hermann et al., 2018). However, our findings reveal that when evaluated at a broader timescale (8.2–4.2 ka), the Middle Holocene PNW is cool and wet (Fig. 2). We posit two hypotheses to reconcile these differences. First, we propose that the coastal PNW was wet and cool whereas interior areas may have been dry (Hermann et al., 2018). Alternatively, in the PNW, this time period may have been characterized by seasonal hydroclimate extremes, multiple records identify high winter precipitation and warm summers with high evaporation (Marlon et al., 2006; Whitlock et al., 2008; Steinman et al., 2016), thus exhibiting both wet and dry phases on short timescales. As such, seasonally resolved proxies are particularly advantageous to fully capture climate in the past.
The shift in SW terrestrial hydroclimate from wet in the Early Holocene to dry in the Middle Holocene (Fig. 2) may be due to several processes. A southward shift in NAM occurred between 9 and 6 ka, resulting in Mexico, rather than California, receiving a higher amount of precipitation (Barron et al., 2012; Metcalfe, 2015). Further, pluvial episodes driven by atmospheric river-type storms are rare in the Middle Holocene, with the exception of one pluvial episode from 7.0–6.4 ka that is synchronous across several Southern California records (Kirby et al., 2012). Both phenomena (atmospheric rivers and NAM) are linked to changes in eastern tropical Pacific SSTs, rather than adjacent marine conditions in the California Current System (Fisler and Hendy, 2008; Kirby et al., 2012; Metcalfe, 2015). Thus, the lack of precipitation led to overall aridity in the Southwest in the Middle Holocene and may not have been driven by local changes in SST of adjacent marine systems (Fig. 2).
3.2.2 Terrestrial climate
Between 8.2 and 4.2 ka, terrestrial conditions were generally cool and wet in the PNW and warm and dry in the SW, while marine systems were generally cool (Fig. 2), yet the Middle Holocene was a time of transition and variability in terrestrial climate. A transition to cooler, wetter conditions during the Middle Holocene occurred across several sites in the PNW (Nederbragt and Thurow, 2001; Marlon et al., 2006; Long et al., 1998; Walsh et al., 2017). For example, lake sediment records from north-central Washington (Scanlon and Castor Lake) demonstrate the variability in Middle Holocene hydroclimate including low lake stand at 7 ka and high lake stand at 5 ka (Steinman et al., 2019).
Fire records from the PNW indicate a transition in hydroclimate and vegetation (Fig. 3). Fire activity increased on Vancouver Island, in the Oregon Coast Range, and in the Klamath and Siskiyou mountains through this interval (Brown and Hebda, 2002; Briles et al., 2005; Whitlock et al., 2008; Long et al., 1998). In Oregon and the Northern Rocky Mountains, the combination of cooler, wetter conditions increased fuels and hot, dry summers led to more severe and larger fires relative to the Early Holocene (Marlon et al., 2006; Whitlock et al., 2008). In coastal areas, increases in fire activity were linked to increased summer drought over time (Briles et al., 2005; Whitlock et al., 2008). Yet, in other nearby regions, fire activity was variable: on Mount Rainier, fire activity increased from 8 to 6.6 ka and decreased from 6.6 to 4 ka (Walsh et al., 2017), in northern Washington, fire frequency decreased at the start of the Middle Holocene with the development of cooler, wetter summers, yet increased again at 4.5 ka (Gavin et al., 2007).
In the Middle Holocene, the Sierra Nevada was dry relative to the Early and Late Holocene (Lindstrom, 1990; Benson et al., 2002; Hallett and Anderson, 2010; MacDonald et al., 2016). A northerly shift in the Intertropical Convergence Zone (ITCZ) in the Middle Holocene led to reduced winter precipitation (including snowpack), which led to drier summers and increased fire frequency relative to Early Holocene (Hallett and Anderson, 2010). Previous work investigating paired marine–terrestrial records from the Sierra Nevada (Kirman Lake) and eastern tropical Pacific highlight the connection between SST cooling (in the eastern tropical Pacific) and increased aridity (MacDonald et al., 2016). The marine–terrestrial link driving precipitation patterns was strongest throughout the Middle Holocene, particularly from ∼8 to 3 ka (MacDonald et al., 2016). The combination of aridity and increased biomass led to control of fire by climate in the Sierra Nevada mountains (Fig. 3) (Hallett and Anderson, 2010). Increased fire activity was synchronous with warming in the Eastern Sierra beginning at 5 ka (Hallett and Anderson, 2010). Additionally, high fire frequency in Lake Tahoe basin in the Middle Holocene (peak at 6.5 ka) was synchronous with high fire frequency in Northern California and Oregon and is attributed to warm dry climate in the Lake Tahoe basin at this time (Beaty and Taylor, 2009).
In the SW, the Middle Holocene is characterized by generalized arid conditions. Lake records from the Mojave Desert (Silver Lake, California) indicate a distinct dry period from ∼ 4.2–7.8 ka (Kirby et al., 2015). Vegetation traces (macrofossils and pollen) from pack rat middens in the Mojave Desert indicate increased aridity compared to modern with a particularly arid period from 6.8 to 5.0 ka (Spaulding, 1991). Pollen records from marine sediments offshore Central California identify a “Middle Holocene dry period” from 8–6.4 ka (McGann, 2015). On the Channel Islands, hydroclimate conditions shifted from dry to wet at ∼6.9 ka, coincident with an increase in fire activity (Anderson et al., 2010). Despite aridity in the desert SW, marine sediment records from Santa Barbara Basin show extreme precipitation events with increased flooding occurring between 7.3–6.6 and 5.5–3.0 ka (Du et al., 2018). Finally, lake sediment records from Lake Elsinore show multiple pluvial episodes and lower relative evaporation in the Middle Holocene (Kirby et al., 2019).
3.2.3 Marine conditions
In the Middle Holocene, records indicate that off the coast of Oregon and California, sea surface temperatures were cool, while the Santa Barbara Basin was warm (Fig. 2) (Mix et al., 1999; Fisler and Hendy, 2008; Barron and Anderson, 2011; Barron et al., 2022). Generally, the North Pacific was experiencing negative PDO or La Niña-like conditions, with a weak/west-shifted winter Aleutian Low and strong/northward summer North Pacific High (Barron and Anderson, 2011). A dominant feature of Middle Holocene eastern North Pacific oceanography was an increase in coastal upwelling. Marine sediment records from offshore of Northern California (40.9∘ N, 124.6∘ W) suggests a 2-fold increase in upwelling and coastal fog beginning at 5.4 ka and a decrease in SST at 5 ka (Barron et al., 2003; Addison et al., 2018). Coastal upwelling off California was enhanced during the summer and fall but suppressed during the spring as a result of a strong North Pacific High driven by orbital forcing (Diffenbaugh et al., 2003; Diffenbaugh and Ashfaq, 2007; Barron and Anderson, 2011). The increase in upwelling is due to a strengthening of the California Current and is documented as far south as Point Conception but is not documented in Santa Barbara Basin (Barron and Bukry, 2007a). Despite a cooling trend seen in multiple records from off the coast of Oregon and Northern California, records from offshore Central California (Farallon Escarpment) show a slight warming trend through time, but at a decreased rate relative to the Early Holocene, a decrease in carbonate productivity and total carbon export, relative to the Early Holocene and continued low oxygen at depth (McGann, 2011). Warming conditions through the Middle Holocene observed offshore Central California conflict with studies from further north showing a cooling trend but compare favorably with records of warming from farther south (McGann, 2011).
Sea surface temperatures during the Middle Holocene were generally warm in Santa Barbara Basin and periodically punctuated by short cool periods at 8.2, 7.6, 5.0, and 4.2 ka (Fisler and Hendy, 2008) and an interval of cold, high productivity from 5.8–6.3 ka (Kennett et al., 2007). Additional evidence from Santa Barbara Basin shows that the Middle Holocene was warmer and more variable than the Early Holocene, which may have been due to increased decadal-scale variability (Friddell et al., 2003; Fisler and Hendy, 2008). SSTs in Santa Barbara Basin initially rose by 3–4 ∘C followed by an abrupt increase of >2 ∘C in less than 40 years at 7.5 ka (Friddell et al., 2003). Nearshore conditions on the Channel Islands were variable with an interval of cooler than modern SST during 6.3–5.3 ka preceded and followed by intervals of warmer than modern SSTs (Glassow et al., 2012). Circulation and ventilation of relatively young North Pacific Intermediate Water into Santa Barbara Basin was stable through the Middle Holocene (Roark et al., 2003). When compared to global records, SST trends from the North Pacific (generalized warming in the North Pacific from 7 ka to present) are inversely correlated with the SST in the North Atlantic (Kim et al., 2004).
3.2.4 Ecological responses to climate change
In the PNW, ecosystems responded to increases in moisture in the Middle Holocene. The establishment of rainforest taxa in Northern Cascades forest occurred during wet conditions and a variable fire regime in the Middle Holocene (Hallett et al., 2003). Lake sediment records from near Mount Rainier show a shift to cooler, wetter conditions and a transition to mesic forests, indicating an increase in effective moisture (Walsh et al., 2017). In British Columbia, mild winters led to an increase in oaks, hemlock, and grasses during this interval (Pellatt et al., 2001). In Northern Washington, lodgepole pine-dominated forest transitioned to a forest with abundant western white pines and Douglas-fir trees (Gavin et al., 2003, 2007). Near the end of the Middle Holocene (∼4.5 ka), fire frequency once again increased, despite the development of the modern western hemlock and red cedar forest that typically indicate cooler, wetter climate and mild summers (Gavin et al., 2003, 2007). In contrast, one record from the Saanich Inlet (British Columbia) shows that oak and Douglas fir increased during this time (Heusser, 1983, p. 19). In Northern California, terrestrial systems shifted from dominance by pine to an increased presence of Sequoia coastal redwoods, indicating an increase in coastal fog (Barron et al., 2018).
Farther south, pollen records from Santa Barbara Basin show numerous changes in terrestrial ecosystems during this interval, including a decrease in Pinus and conifers; a general decrease in all trees leads to a temporary peak in herbaceous plants (Asteraceae) (at 5 ka) followed by a transition to a chaparral, coastal-sage-scrub-dominated ecosystem and an increase in oaks (Heusser, 1978, 1998). A brief period of dry conditions from 8–6.4 ka led to an increase in herbs (Asteraceae) and pines with a coincident decrease in oaks and tan oak (N. densiflora) in Central California (McGann, 2015). In Southern California, salt marsh fauna peaks at 6 ka and riparian community plants generally decrease through the Holocene, indicating changes that are linked to drying trends in the SW in the Middle Holocene (Heusser, 1978).
In marine systems offshore Northern California, the increase in upwelling through the Middle Holocene impacted both planktic and benthic ecosystems. For example, T. nitzschioides dominate the diatom/silicoflagellate assemblage, which indicates a shallow thermocline, high productivity within the California Current, and increased upwelling (Gardner et al., 1988; Barron et al., 2018). In Santa Barbara Basin, an increase in warm water planktic foraminifera and a Neogloboquadrina incompta-dominated assemblage indicates a stable, warm, and stratified water column (Fisler and Hendy, 2008). The establishment of the modern low-oxygen zone continues in this interval, with important implications for seafloor ecosystems including the development of a novel community between 7–5 ka, associated with sulfide-rich, anoxic chemosynthetic seafloor ecosystems in Santa Barbara Basin (Moffitt et al., 2015).
3.3 Late Holocene (4.2 ka–present)
Thirty-four papers encompassing 57 sites met the criteria for spatiotemporal analysis in the Late Holocene and were coded and plotted in Figs. 2 and 3. Archives included lake sediments (41 sites), marine sediments (14 sites), and two others (dendrochronology and lichenometry) (Appendix A, B).
3.3.1 Regional synthesis and marine–terrestrial interactions
Analysis of coded hydroclimate, temperature, and fire history shows that the Late Holocene was characterized by a wet PNW and wet and variable SW relative to the Early and Middle Holocene (Fig. 2). Marine temperatures were warm while terrestrial temperatures were cool and variable across both regions (Fig. 2). Fire activity was high and varied across both regions (Fig. 3). In the PNW, SSTs were warm, upwelling activity was high, coastal fog and moisture increased, fire activity was high, and the overall hydroclimate was variable (Fig. 4). In the SW, SSTs were variable, fire activity was high and variable, hydroclimate was generally wet, and human–environment interactions increased as populations in this region grew (Fig. 5). Although for some records, temporal resolution increases towards the present, increased variability relative to the Early and Middle Holocene is persistent across multiple proxy types and temporal scale of record.
In comparison to the Early and Middle Holocene, the Late Holocene shows greater variability in hydroclimate, temperature, and fire history both within and among records. Marine–terrestrial linkages in the Late Holocene also show high variability and an increase in dominance of decadal to annual phenomena (Fig. 2). Coastal upwelling and precipitation are linked; intervals of stronger upwelling are linked to wetter conditions, and reduced upwelling is tied to aridity. In the Late Holocene, upwelling and precipitation increased in magnitude and variability (Ingram, 1998; Addison et al., 2018). Changes in PDO exerted control on terrestrial hydroclimate; a period of negative PDO at 1.1–0.7 ka, with cooler North Pacific SST and higher upwelling, is coeval with prolonged drought in western North America (MacDonald and Case, 2005). Southwestern droughts in Late Holocene co-occur with cooler than average SST in Santa Barbara Basin. Similarly, nearshore records from Central California show high SST variability coincident with drought during the interval from 0.7–0.5 ka (Fig. 2) (Jones and Kennett, 1999; Barron et al., 2010). Late Holocene changes to PDO and ENSO progressed northward, with enhanced ENSO/PDO effects beginning at 4 ka in Southern California and 3.4 ka in Northern California (Barron and Anderson, 2011; Arellano-Torres et al., 2019). Further, the Late Holocene exhibited alternating wet and dry cycles that may be linked oscillation of precipitation sources between tropical and North Pacific-sourced water (Feakins et al., 2014; Kirby et al., 2014). High variability within and between records (Figs. 2, 3) is a dominant trend across both the PNW and SW in the Late Holocene.
3.3.2 Terrestrial climate
Hydroclimate varies spatially and temporally through the Late Holocene with the highest and most variable fire activity relative to the Early or Middle Holocene (Figs. 2, 3). In the PNW, multiple records show a cool and wet climate, with pronounced wet periods (Mohr et al., 2000; Nederbragt and Thurow, 2001; Reyes and Clague, 2004; Briles et al., 2005; Malamud-Roam et al., 2006). However, trends of increasing aridity towards the present and intervals of drought are also documented (Steinman et al., 2016, 2019; Walsh et al., 2017; Shuman et al., 2018). In the San Francisco Bay region, hydroclimate was also variable, with intervals of aridity documented at 3.0–2.5 and 1.7–0.73 ka (Byrne et al., 2001; McGann, 2008).
In the PNW, fire activity was high and variable in the Late Holocene, despite intra-regional differences in hydroclimate and vegetation. Fire activity on Mount Rainier was highest in the past 4 kyr relative to the rest of the Holocene, and conditions became increasingly dry throughout the Late Holocene (Steinman et al., 2016, 2019; Walsh et al., 2017). Vegetation records show a surprising dominance of flora associated with cooler, wetter conditions despite the highest number of fire episodes and shortest fire return intervals during this period (Walsh et al., 2017). In North Cascades National Park in Northern Washington, high variability in fire frequency and lower synchrony among forest fire events occurred even within the same forest; a decrease in fire frequency from 3.5–2.4 ka corresponded with a cool, humid climate, while high fire frequency from 2.4–1.3 ka suggests more frequent prolonged summer drought (Hallett et al., 2003; Gavin et al., 2007). In this region, the present-day fire regime was established by 1.3 ka (Hallett et al., 2003; Gavin et al., 2007). In the Oregon Coast Range, changes in vegetation led to a decrease in fire activity beginning at 2.75 ka (Gavin et al., 2007). The increase in fire frequency and variability in the PNW may have been due to changes in forest composition, changes in temperature and precipitation, increased ENSO frequency leading to increased summer drought, or due to increases in human population and, thus, human-induced fire (Walsh et al., 2017). Despite an increase in precipitation and a decrease in temperature, charcoal accumulation rates from the Oregon Coast Range and Northern California (Klamath–Siskiyou Mountains) suggest that fires became larger and more severe in this region, with high variability during the latter part of the Holocene, likely due to increases in woody vegetation to fuel forest fires (Marlon et al., 2006, 2013; Whitlock et al., 2008).
Spatial and temporal variability in climate and fire history occurred across the Sierra Nevada mountains (Figs. 2, 3). In the Eastern Sierras, hydroclimate varied throughout the Late Holocene; Mono Lake water level fluctuated through this time (3.7 ka – highest in last 7 kyr, 1.8 ka low stand, multiple rapid fluctuations in last 1.2 kyr), Owens Lake exhibited three intervals of wetter and cooler climate at 3.6, 0.8, and 0.35 ka (Bacon et al., 2018), and increased precipitation through the Late Holocene at Pyramid Lake led to rising lake levels (Benson et al., 2002). Strong fire synchrony has occurred in the Sierra Nevada since 2.5 ka, which is linked to periods of drought (Hallett and Anderson, 2010). In the Lake Tahoe basin, changes in vegetation from open forest to mesic forest drive a decline in fire frequency from the Mid-Holocene to modern, with a particularly low fire interval from 4.0 to 3.5 ka (Beaty and Taylor, 2009). Records from the Sierra Nevada show glacial advancement linked to cooling beginning at 3.9 ka (Konrad and Clark, 1998).
In Central and Southern California, multiple wet and dry phases occurred through the Late Holocene (Fig. 2) (Feakins et al., 2014; Kirby et al., 2014). In coastal Central California, marine records show that winter precipitation increased in the Late Holocene (McGann, 2011). Several lake records from Southern California (Lower Bear Lake, Lake Elsinore, Tulare Lake, and Owens Lake) all indicate a return to wetter conditions beginning between 4.0–3.35 ka (Kirby et al., 2010, 2014). Records from coastal lagoons on Santa Rosa Island and in Southern California show multi-centennial fluctuations between wet and dry conditions (Davis, 1992; Anderson and Byrd, 1998; Cole and Wahl, 2000; Anderson et al., 2010). Similarly, records from Southern California indicate multiple wet and dry intervals that be linked to alternation of precipitation source water between more tropical and more North Pacific source water (Dingemans et al., 2014; Feakins et al., 2014; Kirby et al., 2014, 2019). A dry period between 2.5 and 2.0 ka is synchronous across multiple records from Southern California (Dingemans et al., 2014; Kirby et al., 2014, 2019; Platzman and Lund, 2019), and persistent droughts and elevated aridity are dominant across the Western US from 1.1 to 0.7 ka (Cook, 2004). In the Mojave Desert (Silver Lake, California) conditions returned to ephemeral, wet lake conditions beginning at 3.8 ka after a Middle Holocene period of aridity (Fig. 5) (Kirby et al., 2015). Yet other records show increased aridity in the Mojave Desert compared to the Middle Holocene (Spaulding, 1991).
Across the study region, extreme events (rainfall, drought, flooding) become a dominant feature across multiple records over the past 2 kyr (Cook, 2004; Malamud-Roam et al., 2006; Rasmussen et al., 2006; Kirby et al., 2015). A pronounced megadrought occurred in western North America from 1.1–0.7 ka that was previously linked to cool SST over the eastern tropical North Pacific (Cook, 2004). More variable conditions have been attributed to changes in insolation as well as shorter-term processes including ENSO and PDO (Cook, 2004; Kirby et al., 2007, 2010; Barron and Anderson, 2011). Gradual decreases in summer insolation and increases in winter insolation contribute to maximum drying in the Late Holocene relative to the Early Holocene (Kirby et al., 2007). Enhanced PDO and ENSO variability in the last 4 kyr contribute to increased terrestrial hydroclimate and temperature variability (Kirby et al., 2010; Barron and Anderson, 2011).
Human control of the fire regime led to land use and ecosystem changes across the Western US during this interval. In Washington, an increase in human artifacts in the subalpine zone beginning at 3.6 ka, combined with a change in climate and thus ranges of game species, provides some evidence for increased presence of people in subalpine terrain and potentially an increase in human-caused fire (Walsh et al., 2017). Across California, Late Holocene increases in chaparral, grassland, and sage scrub ecosystems were reinforced by intentional burning and prescribed burning played an important role in structuring ecosystems (Heusser, 1978; Anderson et al., 2010, 2013; Cowart and Byrne, 2013; Crawford et al., 2015; Lightfoot and Cuthrell, 2015; Klimaszewski-Patterson et al., 2021).
3.3.3 Marine conditions
In the Late Holocene, SST increased off the coast of Oregon and California and SST decreased in the Santa Barbara Basin relative to the Middle Holocene (Figs. 2, 4, 5) (Barron et al., 2003; Kennett et al., 2007; Barron and Anderson, 2011). Late Holocene marine conditions show increased variability in SST, intensification of the California Current, and increased upwelling relative to the Middle Holocene (Fig. 3) (Barron and Bukry, 2007a; Barron and Anderson, 2011). While global records suggest a shift in climate characteristics at 4.2 ka, several California and Oregon records experience a delayed Middle to Late Holocene shift (Barron and Bukry, 2007a; Addison et al., 2018; Barron et al., 2018). Modern seasonality within the California Current (strong spring–summer upwelling with cool SSTs, and relaxation with warmer SSTs during fall) was established between 3.5 and 3.2 ka (Barron and Bukry, 2007a). Diatom-based productivity increased at 2.9 ka offshore of Northern California, and changes in relative abundance of diatom and pollen species suggest significantly increased upwelling and productivity at this time (Addison et al., 2018). Additional evidence from offshore of Northern California shows cooler SSTs occurred from ∼3.6 and 2.8 ka, accompanied by increased precipitation, an abrupt warming of winter SSTs at 2.8 ka, and an intensification of spring upwelling beginning 2.6 ka (Barron et al., 2018). Since 2.6 ka, PDO-like warm–cool oscillations have dominated the SST regime (Barron et al., 2018).
Further south, off the coast of Santa Cruz, California, upwelling and productivity increased steadily through the Late Holocene (Barron et al., 2022). Additional records from offshore Central California indicate the Late Holocene marked the onset of warm modern offshore conditions with high productivity (total and organic carbon export) relative to the Middle and Early Holocene (McGann, 2011; Barron et al., 2022). Marine oxygenation off the coast of Central California declined through the Late Holocene (McGann, 2011). Nearshore records from Central California show that SST was 1 ∘C cooler than modern from 2–0.7 ka (Jones and Kennett, 1999).
In Southern California, Late Holocene SST cooling with high SST variability (in Santa Barbara Basin) began at ∼4 ka (Fig. 5) (Kennett et al., 2007; Fisler and Hendy, 2008). High amplitude changes in salinity due to variability of input of freshwater into the basin are documented within Santa Barbara Basin (Kennett et al., 2007). Brief cold events occurred in Santa Barbara Basin at ∼ 4.2–3.8, 1.5–1.2, and 0.8–0.3 ka and correspond to glacial advances in the Sierras (Fisler and Hendy, 2008). Variable oceanographic conditions in the Late Holocene may be due to increasing ENSO variability in the tropical Pacific (Barron and Bukry, 2007b). Intensification of the California Current and increased east–west seasonal gradients (driving increased winds that enhance upwelling) are documented offshore Northern California (Barron et al., 2003; Barron and Bukry, 2007a, b) and across the Southern California Borderlands. Nearshore records from Santa Cruz Island show persistently cold SSTs and persistent upwelling on the western coast of Santa Cruz Island in Late Holocene compared to a wider range of nearshore conditions on the south side of the island (Flores, 2017). In deeper waters, a drastic change in the age of North Pacific Intermediate Water in Santa Barbara Basin (shift to older water and lower bioturbation) at 2 ka with a subsequent stable age of water entering from 2 ka to present suggests changes in the North Pacific Intermediate Water circulation pattern (Roark et al., 2003). This shift is concurrent with a decrease in Greenland temperature and increase in ice accumulation rate suggesting trends in Santa Barbara Basin relate to larger atmospheric circulation patterns (Roark et al., 2003). Further, the oxygen minimum zone persists in intermediate waters off the coast of Southern California but is reduced in intensity relative to the Middle Holocene (Balestra et al., 2018; Wang et al., 2020). Importantly, most records from this region are within the Southern California Bight and shoreward of the California Current. Further work is needed to understand the southern portion of the California Current and the impact of ENSO and PDO through this time interval (Arellano-Torres et al., 2019).
3.3.4 Ecological responses to change
Forested ecosystems in the PNW and Northern California transitioned to modern temperate, cool, wet, highly fire-adapted systems with increased fir and pine (Heusser, 1983; Mohr et al., 2000; Pellatt et al., 2001; Barron et al., 2003, 2022; Briles et al., 2005; Anderson et al., 2013) while SW systems became increasingly dominated by chaparral and sage scrub ecosystems (particularly since ∼2.5 ka) (Heusser, 1978; Anderson and Byrd, 1998; Cole and Wahl, 2000; Anderson et al., 2010; Dingemans et al., 2014). In the Siskiyou mountains, a shift in hydroclimate to wet, cool conditions at 2.1 ka led to the development of the modern forest dominated by firs (Abies) and cypress (Pseudotsuga) and moderated by a modern fire regime (Briles et al., 2005). In parallel with increases in hydroclimate and temperature variability, in Northern California the amplitude and frequency of changes between pine and alder intensified in the Late Holocene and coastal scrub expanded (Fig. 4) (Barron et al., 2003; Anderson et al., 2013). In Central California, changes to forest assemblages occurred gradually through time, trending towards decreased dominance of pine in the modern (Fig. 5) (McGann, 2015; Barron et al., 2022). Finally, in Southern California, ecological change varied locally, with transitions in pollen regimes indicating increased moisture occurring around 2 ka in multiple records (Anderson and Byrd, 1998; Cole and Wahl, 2000; Dingemans et al., 2014).
Marine ecosystems reflect the establishment of modern levels of coastal upwelling and productivity in the Late Holocene (Addison et al., 2018; Barron et al., 2018). Offshore Northern California, the gyre-associated diatom species Pseudoeunotia doliolus increased threefold indicating the onset of modern oceanographic conditions in this region (Barron et al., 2003). Offshore Central California, upwelling evolved gradually over time and the upwelling-indicator diatom species T. nitzschioides gradually increased through this interval (Barron et al., 2022). In Santa Barbara Basin, Late Holocene cooling led to an increase in the abundance of cold-associated (N. pachyderma) and upwelling-associated (Globigerina quinqueloba) planktic foraminifera (Fisler and Hendy, 2008). Oxygen deficient zones were well established by the Late Holocene and persisted throughout the interval, structuring seafloor and pelagic ecosystems (Ohkushi et al., 2013; Moffitt et al., 2014, 2015; Palmer et al., 2022). As human populations increased throughout the Late Holocene, human harvesting pressure on marine ecosystems increased, including an expansion of consumption of finfish and pinnipeds and a diversification of taxa in middens (e.g., Erlandson et al., 2009; Rick, 2011; Braje et al., 2012).
3.4 Medieval Climate Anomaly and Little Ice Age
Specific investigation of the Medieval Climate Anomaly (MCA) and the Little Ice Age (LIA) is included in some of the papers reviewed here. Generally, the MCA was an anomalously warm event that is linked to pronounced drought conditions throughout the Western United States. Alternatively, the LIA was a brief period of cooling predominantly identified in Northern Hemisphere records (Cook, 2004). Evidence of dry conditions during the MCA is indicated in a breadth of records: from Mono Lake and Owens Lake in the Eastern Sierra Nevada (Stine, 1990; Bacon et al., 2018), freshwater input into San Francisco Bay, Abbot Lake and Zaca Lake in coastal Central California (Dingemans et al., 2014; Hiner et al., 2016; Platzman and Lund, 2019), Central California pollen history (McGann, 2015), flood events in Santa Barbara Basin (Schimmelmann et al., 2013; Du et al., 2018), tree stump dating in the Sierra Nevada (Stine, 1994), and a synthesis of tree-ring records (Cook, 2004). Dry conditions were likely caused by anomalously warm terrestrial temperatures and are linked to cool SSTs over the eastern tropical Pacific (Cook, 2004) and in the adjacent Santa Barbara Basin (Kennett and Kennett, 2000; Fisler and Hendy, 2008; Barron et al., 2010; Asmerom et al., 2013). Fire activity increased in the MCA in the Eastern Sierra and Lake Tahoe basin, which may be due to warm summer enhanced growth and decreased snowpack, but in western North America more broadly, fire activity was reduced during the MCA and LIA relative to the past 3 kyr (Beaty and Taylor, 2009; Hallett and Anderson, 2010). The LIA was characterized by warm SSTs in Southern California, wetter conditions in the Sierra Nevada and Southern California, and minimum fire frequency in western North America (Reyes and Clague, 2004; Whitlock et al., 2008; Barron et al., 2010; Feakins et al., 2014; Kirby et al., 2019).
3.5 Era of Colonization
Humans have been interacting with the environment in western North America throughout the entirety of the Holocene. Indigenous peoples exerted control of fire and interacted with, managed, and cultivated marine and terrestrial plants and animals throughout the Holocene (e.g., Minnis, 2004; Braje et al., 2012; McKechnie, 2015; Taylor et al., 2016; Edinborough et al., 2017; Beck et al., 2018; Platzman and Lund, 2019; Ellis et al., 2021; Klimaszewski-Patterson et al., 2021). As populations of Indigenous North Americans increased throughout the Holocene, human–environment interactions including marine and terrestrial resource use and prescribed fire increased (Heusser, 1978; Anderson et al., 2010, 2013; Cowart and Byrne, 2013; Crawford et al., 2015; Lightfoot and Cuthrell, 2015; Klimaszewski-Patterson et al., 2021). Additionally, climate changes impacted communities throughout the Holocene; intervals of climate stability are correlated with consistent resource use, while intervals of climate instability and drought are related to migration, changing resource use, and conflict (Grenda and Benitez, 1997; Ingram, 1998; Jones and Kennett, 1999; Kennett and Kennett, 2000; deMenocal, 2001; Kennett et al., 2007). While human–environment interactions and Indigenous land stewardship were environmental and ecological drivers throughout the Holocene, the onset of colonization, genocide of Indigenous people, land-use change, urbanization, industrialization, and anthropogenic climate change are evident in many records examined here and represent an unprecedented environmental regime in multiple records (e.g., Ellis et al., 2021).
Impacts of land-use change and human control of fire at the time of colonization precede changes due to urbanization and anthropogenic climate change (Crawford et al., 2015; Waters et al., 2016). Changes in the view and management of fire have shaped the landscape and climate in the last several hundred years, beginning with a measurable increase in fire (relative to Indigenous prescribed burning) following settler colonization and a subsequent measurable decrease in fire in the last 100 years due to fire suppression (Gavin et al., 2007; Anderson et al., 2010, 2013; Dingemans et al., 2014; Crawford et al., 2015; Taylor et al., 2016; Walsh et al., 2017; Platzman and Lund, 2019). Prior to 1860, fire activity in the Sierra Nevada was positively correlated with temperature, but in the last 150 years (after 1860 CE) fires have been linked to human activity and socio-ecological change, rather than temperature or moisture (Anderson et al., 2013; Taylor et al., 2016). In the Sierra Nevada the highest fire activity in the last 400 years occurred following Spanish colonialism and the lowest fire activity occurred with the onset of fire suppression beginning in 1904 CE (Taylor et al., 2016). In the Klamath Mountains, fire activity increased as Native American population increased in the last 1.5 kyr and then abruptly decreased with the onset of colonization (Crawford et al., 2015). In coastal Central California recent increases in fire beginning in the mid 19th century are attributed to burning of logging slash (Cowart and Byrne, 2013).
Increases in extractive processes and land-use conversions for ranching and agriculture have also contributed to changing fire regimes over the last several hundred years and also led to changes in hydrology and landscape geomorphology (Heusser, 1983; Byrne et al., 2001; Cowart and Byrne, 2013; Platzman and Lund, 2019). Pollen records in British Columbia show the impacts of colonization, logging, farming, and urbanization on local terrestrial ecosystems (Heusser, 1983). Influx of freshwater to the San Francisco Bay decreased dramatically relative to the Late Holocene at 1930 CE due to diversion of upstream water flow (Byrne et al., 2001). The extractive processes of the Gold Rush Era (beginning at 1850) in Northern California led to increased sedimentation and heavy metal pollution in the San Francisco Bay delta; post-colonization lead (Pb) levels are unprecedented throughout the rest of the Holocene and concentrations of Sr, Ti, Cu, Ni, and Zn also increased following colonization (Fard et al., 2021). The onset of logging and ranching caused landscape conversion including transition of redwood forest to grasses and oak landscapes in coastal Central California (Cowart and Byrne, 2013). Further, the introduction of non-native species is well documented across the region (Anderson and Byrd, 1998; Cole and Wahl, 2000; Anderson et al., 2010, 2013; Dingemans et al., 2014). The impact of both a changing fire regime and land use change resulted in shifts in terrestrial ecosystems (Cowart and Byrne, 2013; Dingemans et al., 2014; Platzman and Lund, 2019).
Human impacts on nearshore marine ecosystems are documented throughout the Holocene. Yet widespread and detectable impacts of land use change and anthropogenic climate change on marine systems do not appear until the last several hundred years, following colonization and the Industrial Revolution, respectively. Land use change and the conversion of land to rangeland and cultivated agricultural land beginning around 1800 CE led to the collapse of benthic marine ecosystems and may have contributed to a decrease in oxygenation across the Southern California Bight (Christensen et al., 1994; Tomasovych and Kidwell, 2017; Wang et al., 2017; Palmer et al., 2020). Impacts of anthropogenic climate change on marine systems in this region include species range shifts caused by rising SSTs and geochemical and structural changes to organisms due to ocean acidification; these changes represent a divergence from scales of past variability (Field et al., 2006; Pak et al., 2018; Osborne et al., 2020). Human activity, including intentional use and alteration of marine and terrestrial systems, has been a feature of western North America throughout the Holocene that is detectable in multiple archives (lake sediments, marine sediments, archeological records), yet colonization, urbanization, and industrialization led to a novel environmental interval.
Understanding Holocene climate and oceanographic patterns in the Western United States and California Current System is critical for contextualizing and predicting modern changes. Relative to pre-Holocene conditions, the Early Holocene was dry and warm in the PNW and wet and warm in the SW; sea surface temperature was warm along the coast (Fig. 2). The Middle Holocene was characterized by a wet, cool PNW and dry, warm SW with generalized cool SST, except for Santa Barbara Basin (Fig. 2). The Late Holocene is the most variable interval with a general pattern of warm marine conditions and cool terrestrial conditions (Fig. 2). Control of fire regime shifts throughout the Holocene; in the Early Holocene, fire activity is linked to changes in vegetation following the deglacial, but in multiple locations it shifts to control by climate in the Middle Holocene and in most records is highest and most variable in the Late Holocene (Fig. 3). While human–environment interactions persist throughout the Holocene and impact the climate, landscape, and ecosystems of western North America, the impacts of settler colonization, urbanization, and industrialization in recent centuries are documented broadly and, in some cases, represent divergences from scales of expected variability as recorded throughout the rest of the Holocene.
Multiple factors combine and interact to drive climate changes through the Holocene including orbital forcing, ice sheet dynamics, solar forcing, annual to decadal phenomena, and marine–terrestrial interactions. Orbital forcing, including the Northern Hemisphere summer insolation peak in the Early Holocene, drives climate change on millennial timescales. Annual to decadal phenomena such as ENSO and PDO become dominant drivers of climate in the Late Holocene and lead to increased temporal variability. The relationship between marine and terrestrial systems evolves through time; notably, continental conditions in the Pacific Northwest and Southwest respond differently to changes in marine conditions. In the PNW broadly, marine temperatures are inversely correlated with precipitation. In the Early Holocene, warm SSTs in the PNW coeval with warm, dry inland conditions; in the Middle Holocene, the trend reverses. Yet, the increase in upwelling as a dominant oceanographic feature throughout the Holocene complicates this relationship, as upwelling in the PNW is positively correlated with precipitation yet characterized by cool SSTs. Thus, in the Late Holocene, the interaction between an enhanced upwelling regime yet generalized warm SST patterns may both play a role in influencing terrestrial hydroclimate. In contrast, in the SW, marine temperatures are generally positively correlated with precipitation; warm intervals (such as the Early Holocene) coeval with wet intervals and periods of drought coeval with cool SSTs offshore Southern California. In addition to marine–terrestrial interactions on regional scales, changes in source and strength of precipitation impact hydroclimate of the region; for example, changes in the intensity or geographic extent of the North American monsoon and occurrence of atmospheric–river storms are both linked to processes in the eastern tropical North Pacific and have implications for hydroclimate across the region studied here. Future studies should fill in geographic gaps in sampling of the region and continue to distinguish between trends across temporal scales, including seasonal, annual, and decadal, based on proxy type and preservation.
Although general trends can be identified across broad regions (PNW and SW), local factors play a key role in determining the exact conditions for a particular location. Additionally, age control challenges and proxy sensitivity issues (including seasonality) complicate Holocene climate reconstruction. Complete synchrony between records across a full time period for marine conditions, for terrestrial climate, or for fire activity is functionally absent; multiple anomalous or contradictory records exist (Figs. 2, 3). For example, fire activity in adjacent lake basins shows different trends and nearshore vs. offshore records of marine conditions present apparently competing evidence (Fig. 3). Marine sediment records from Santa Barbara Basin are often utilized as records of the entire North Pacific, but here we find that trends in Santa Barbara Basin are not always synchronous with other records in this region (Fig. 2). We posit that these instances do not present competing evidence but rather that local factors are critical in determining local climate. This is of critical importance for interpreting present and future climate change. This synthesis of climate and oceanographic processes and resultant impacts on ecosystems provides a window into understanding modern patterns of climate and climate change in the Western United States and Northeast Pacific and amplifies the need for future research investigating marine–terrestrial interactions, local- and regional-scale processes, and integrated physical–ecological–human systems.
Data generated for this article are included in the Appendix.
HMP and TMH conceptualized and designed the project. All authors completed data curation and literature review. HMP conducted data analysis and wrote the manuscript. All authors contributed to editing of the manuscript.
The contact author has declared that none of the authors has any competing interests.
Publisher’s note: Copernicus Publications remains neutral with regard to jurisdictional claims in published maps and institutional affiliations.
We acknowledge all members of the UC Davis Geology Oceans and Climate Change Course (GEL 232) in spring 2018 for their contributions to initial data gathering and framing of this project. We thank the two anonymous reviewers and John Barron, whose input significantly improved this paper. Finally, we acknowledge that this paper includes histories and discussion of Indigenous peoples of western North America (Turtle Island) who have stewarded the land and sea for millennia. We aim to document the broad histories of interactions between people and environment, but we acknowledge that this record is incomplete due to the erasure of Indigenous histories and oral traditions, genocide of knowledge keepers, and destruction of cultural artifacts. We acknowledge the keepers of intergenerational Indigenous knowledge who maintain stories and data of the human, ecosystem, and climate impacts of settler colonization described here. We do not attempt to name all tribes whose ancestral and present homelands make up this study area, but we acknowledge that the majority of the geographic area covered here represents unceded land of Indigenous tribes and that data used here from previously published studies may have been acquired without consent from Indigenous peoples. We direct readers to the open-source resource https://nativelands.ca/ (last access: August 2022) to identify the homelands of the diverse Indigenous people of this region and encourage readers to use this resource as a starting place to learn about the land and marine stewardship of Indigenous peoples past and present.
This research has been supported by the National Science Foundation (grant no. OCE 1832812) to Tessa M. Hill and the UC Davis Dissertation Year Fellowship to Hannah M. Palmer.
This paper was edited by Marit-Solveig Seidenkrantz and reviewed by two anonymous referees.
Adams, D. K. and Comrie, A. C.: The North American Monsoon, 78, 2197–2214, B. Am. Meteorol. Soc., https://doi.org/10.1175/1520-0477(1997)078<2197:TNAM>2.0.CO;2, 1997.
Addison, J. A., Barron, J., Finney, B., Kusler, J., Bukry, D., Heusser, L. E., and Alexander, C. R.: A Holocene record of ocean productivity and upwelling from the northern California continental slope, Quaternary International, 469, 96–108, https://doi.org/10.1016/j.quaint.2017.02.021, 2018.
Ainsworth, C. H., Samhouri, J. F., Busch, D. S., Cheung, W. W. L., Dunne, J., and Okey, T. A.: Potential impacts of climate change on Northeast Pacific marine foodwebs and fisheries, ICES J. Mar. Sci., 68, 1217–1229, https://doi.org/10.1093/icesjms/fsr043, 2011.
Amante, C. and Eakins, B. E.: ETOPO1 1 Arc-Minute Global Relief Model: Procedures, Data Sources And Analysis, 25, 2009.
Anderson, R. S. and Byrd, B. F.: Late-Holocene Vegetation Changes From The Las Flores Creek Coastal Lowlands, San Diego County, California, 45, 171–182, ISBN 0024-9637, 1998.
Anderson, R. S., Starratt, S., Jass, R. M. B., and Pinter, N.: Fire and vegetation history on Santa Rosa Island, Channel Islands, and long-term environmental change in southern California, J. Quaternary Sci., 25, 782–797, https://doi.org/10.1002/jqs.1358, 2010.
Anderson, R. S., Ejarque, A., Brown, P. M., and Hallett, D. J.: Holocene and historical vegetation change and fire history on the north-central coast of California, USA, The Holocene, 23, 1797–1810, https://doi.org/10.1177/0959683613505344, 2013.
Arellano-Torres, E., Álvarez-Covelli, C., Kasper-Zubillaga, J. J., and Lozano-García, M. del S.: A 14-ka Record of Dust Input and Phytoplankton Regime Changes in the Subtropical NE Pacific: Oceanic and Terrestrial Processes Linked by Teleconnections at Suborbital Scales, Paleoceanography and Paleoclimatology, 34, 35–53, https://doi.org/10.1029/2018PA003479, 2019.
Asmerom, Y., Polyak, V. J., Rasmussen, J. B. T., Burns, S. J., and Lachniet, M.: Multidecadal to multicentury scale collapses of Northern Hemisphere monsoons over the past millennium, P. Natl. Acad. Sci. USA, 110, 9651–9656, https://doi.org/10.1073/pnas.1214870110, 2013.
Atwood, A. R., Wu, E., Frierson, D. M. W., Battisti, D. S., and Sachs, J. P.: Quantifying Climate Forcings and Feedbacks over the Last Millennium in the CMIP5–PMIP3 Models, J. Climate, 29, 1161–1178, https://doi.org/10.1175/JCLI-D-15-0063.1, 2016.
Bacon, S. N., Burke, R. M., Pezzopane, S. K., and Jayko, A. S.: Last glacial maximum and Holocene lake levels of Owens Lake, eastern California, USA, Quaternary Sci. Rev., 25, 1264–1282, https://doi.org/10.1016/j.quascirev.2005.10.014, 2006.
Bacon, S. N., Lancaster, N., Stine, S., Rhodes, E. J., and McCarley Holder, G. A.: A continuous 4000-year lake-level record of Owens Lake, south-central Sierra Nevada, California, USA, Quat. Res., 90, 276–302, https://doi.org/10.1017/qua.2018.50, 2018.
Bader, J., Jungclaus, J., Krivova, N., Lorenz, S., Maycock, A., Raddatz, T., Schmidt, H., Toohey, M., Wu, C.-J., and Claussen, M.: Global temperature modes shed light on the Holocene temperature conundrum, Nat. Commun., 11, 4726, https://doi.org/10.1038/s41467-020-18478-6, 2020.
Balestra, B., Krupinski, N. B. Q., Erohina, T., Fessenden-Rahn, J., Rahn, T., and Paytan, A.: Bottom-water oxygenation and environmental change in Santa Monica Basin, Southern California during the last 23 kyr, 490, 17–37, 2018.
Barber, D. C., Dyke, A., Hillaire-Marcel, C., Jennings, A. E., Andrews, J. T., Kerwin, M. W., Bilodeau, G., McNeely, R., Southon, J., Morehead, M. D., and Gagnon, J.-M.: Forcing of the cold event of 8,200 years ago by catastrophic drainage of Laurentide lakes, Nature, 400, 344–348, https://doi.org/10.1038/22504, 1999.
Barlow, M., Nigam, S., and Berbery, E. H.: Evolution of the North American Monsoon System, J. Climate, 11, 2238–2257, https://doi.org/10.1175/1520-0442(1998)011<2238:EOTNAM>2.0.CO;2, 1998.
Barnosky, A. D., Hadly, E. A., Gonzalez, P., Head, J., Polly, P. D., Lawing, A. M., Eronen, J. T., Ackerly, D. D., Alex, K., Biber, E., Blois, J., Brashares, J., Ceballos, G., Davis, E., Dietl, G. P., Dirzo, R., Doremus, H., Fortelius, M., Greene, H. W., Hellmann, J., Hickler, T., Jackson, S. T., Kemp, M., Koch, P. L., Kremen, C., Lindsey, E. L., Looy, C., Marshall, C. R., Mendenhall, C., Mulch, A., Mychajliw, A. M., Nowak, C., Ramakrishnan, U., Schnitzler, J., Das Shrestha, K., Solari, K., Stegner, L., Stegner, M. A., Stenseth, N. C., Wake, M. H., and Zhang, Z. B.: Merging paleobiology with conservation biology to guide the future of terrestrial ecosystems, Science, 355, 594–604, https://doi.org/10.1126/science.aah4787, 2017.
Barron, J. A. and Anderson, L.: Enhanced Late Holocene ENSO/PDO expression along the margins of the eastern North Pacific, Quatern. Int., 235, 3–12, https://doi.org/10.1016/j.quaint.2010.02.026, 2011.
Barron, J. A. and Bukry, D.: Development of the California Current during the past 12,000 yr based on diatoms and silicoflagellates, Palaeogeogr. Palaeocl., 248, 313–338, https://doi.org/10.1016/j.palaeo.2006.12.009, 2007a.
Barron, J. A. and Bukry, D.: Solar forcing of Gulf of California climate during the past 2000 yr suggested by diatoms and silicoflagellates, Mar. Micropaleontol., 62, 115–139, https://doi.org/10.1016/j.marmicro.2006.08.003, 2007b.
Barron, J. A., Heusser, L., Herbert, T., and Lyle, M.: High-resolution climatic evolution of coastal northern California during the past 16,000 years, Paleoceanography, 18, 1020, https://doi.org/10.1029/2002PA000768, 2003.
Barron, J. A., Bukry, D., and Field, D.: Santa Barbara Basin diatom and silicoflagellate response to global climate anomalies during the past 2200 years, Quatern. Int., 215, 34–44, https://doi.org/10.1016/j.quaint.2008.08.007, 2010.
Barron, J. A., Metcalfe, S. E., and Addison, J. A.: Response of the North American monsoon to regional changes in ocean surface temperature, Paleoceanography, 27, https://doi.org/10.1029/2011PA002235, 2012.
Barron, J. A., Bukry, D., Heusser, L. E., Addison, J. A., and Alexander, C. R.: High-resolution climate of the past ∼7300 years of coastal northernmost California: Results from diatoms, silicoflagellates, and pollen, Quaternary Int., 469, 109–119, https://doi.org/10.1016/j.quaint.2016.10.039, 2018.
Barron, J. A., Addison, J. A., Heusser, L. E., Bukry, D., Schwartz, V., and Wagner, A.: An 11,300 yr record of paleoclimatology and paleoceanography of the central California coast in a gravity core from Pioneer Seamount, Quaternary International, 621, 74–83, https://doi.org/10.1016/j.quaint.2019.12.019, 2022.
Batchelder, H. P. and Powell, T. M.: Physical and biological conditions and processes in the northeast Pacific Ocean, Prog. Oceanogr., 53, 105–114, https://doi.org/10.1016/S0079-6611(02)00026-5, 2002.
Beaty, R. M. and Taylor, A. H.: A 14 000 year sedimentary charcoal record of fire from the northern Sierra Nevada, Lake Tahoe Basin, California, USA, The Holocene, 19, 347–358, https://doi.org/10.1177/0959683608101386, 2009.
Becerra-Valdivia, L. and Higham, T.: The timing and effect of the earliest human arrivals in North America, Nature, 584, 93–97, https://doi.org/10.1038/s41586-020-2491-6, 2020.
Beck, C. W., Bryant, V. M., and Jenkins, D. L.: Analysis of Younger Dryas–Early Holocene pollen in sediments of Paisley Cave 2, south-central Oregon, Palynology, 42, 168–179, https://doi.org/10.1080/01916122.2017.1319883, 2018.
Benson, L., Kashgarian, M., Rye, R., Lund, S., Paillet, F., Smoot, J., Kester, C., Mensing, S., Meko, D., and Lindström, S.: Holocene multidecadal and multicentennial droughts affecting Northern California and Nevada, Quaternary Sci. Rev., 21, 659–682, https://doi.org/10.1016/S0277-3791(01)00048-8, 2002.
Bird, B. W. and Kirby, M. E.: An Alpine Lacustrine Record of Early Holocene North American Monsoon Dynamics from Dry Lake, Southern California (USA), J. Paleolimnol., 35, 179–192, https://doi.org/10.1007/s10933-005-8514-3, 2006.
Bird, B. W., Kirby, M. E., Howat, I. M., and Tulaczyk, S.: Geophysical evidence for Holocene lake-level change in southern California (Dry Lake), Boreas, 39, 131–144, https://doi.org/10.1111/j.1502-3885.2009.00114.x, 2010.
Bradley, R. S.: Climate Forcing During the Holocene, PAGES news, 11, 18–19, https://doi.org/10.22498/pages.11.2-3.18, 2003.
Braje, T. J., Rick, T. C., and Erlandson, J. M.: A trans-Holocene historical ecological record of shellfish harvesting on California's Northern Channel Islands, Quaternary Int., 264, 109–120, 2012.
Bray, N. A., Keyes, A., and Morawitz, W. M. L.: The California Current system in the Southern California Bight and the Santa Barbara Channel, J. Geophys. Res.-Oceans, 104, 7695–7714, https://doi.org/10.1029/1998JC900038, 1999.
Briles, C. E., Whitlock, C., and Bartlein, P. J.: Postglacial vegetation, fire, and climate history of the Siskiyou Mountains, Oregon, USA, Quat. res., 64, 44–56, https://doi.org/10.1016/j.yqres.2005.03.001, 2005.
Brown, K. J. and Hebda, R. J.: Origin, development, and dynamics of coastal temperate conifer rainforests of southern Vancouver Island, Canada, Can. J. For. Res., 32, 353–372, https://doi.org/10.1139/x01-197, 2002.
Byrne, R., Ingram, B. L., Starratt, S., Malamud-Roam, F., Collins, J. N., and Conrad, M. E.: Carbon-Isotope, Diatom, and Pollen Evidence for Late Holocene Salinity Change in a Brackish Marsh in the San Francisco Estuary, Quaternary Res., 55, 66–76, https://doi.org/10.1006/qres.2000.2199, 2001.
Cannariato, K. G. and Kennett, J. P.: Climatically related millennial-scale fluctuations in strength of California margin oxygen-minimum zone during the past 60 k.y., Geology, 27, 975–978, https://doi.org/10.1130/0091-7613(1999)027<0975:Crmsfi>2.3.Co;2, 1999.
Chavez, F., Ryan, J., Lluch-Cota, S., and Niquen, M.: From Anchovies to Sardines and Back: multidecadal CHANGE in the Pacific Ocean, Science, 299, 217–21, https://doi.org/10.1126/science.1075880, 2003.
Checkley, D. M. and Barth, J. A.: Patterns and processes in the California Current System, Prog. Oceanogr., 83, 49–64, https://doi.org/10.1016/j.pocean.2009.07.028, 2009.
Chelton, D., Bernal, P., and Mcgowan, J.: Large-scale interannual physical and biological interaction in the California Current (El Nino), J. Mar. Res., 1095–1125, 40, 1982.
Christensen, C. J., Gorsline, D. S., Hammond, D. E., and Lund, S. P.: Non-annual laminations and expansion of anoxic basin-floor conditions in Santa Monica Basin, California Borderland, over the past four centuries, Mar. Geol, 116, 399–418, 1994.
Clark, P. U., Dyke, A. S., Shakun, J. D., Carlson, A. E., Clark, J., Wohlfarth, B., Mitrovica, J. X., Hostetler, S. W., and McCabe, A. M.: The Last Glacial Maximum, Science, 325, 710–714, https://doi.org/10.1126/science.1172873, 2009.
Clark, P. U., Shakun, J. D., Baker, P. A., Bartlein, P. J., Brewer, S., Brook, E., Carlson, A. E., Cheng, H., Kaufman, D. S., Liu, Z., Marchitto, T. M., Mix, A. C., Morrill, C., Otto-Bliesner, B. L., Pahnke, K., Russell, J. M., Whitlock, C., Adkins, J. F., Blois, J. L., Clark, J., Colman, S. M., Curry, W. B., Flower, B. P., He, F., Johnson, T. C., Lynch-Stieglitz, J., Markgraf, V., McManus, J., Mitrovica, J. X., Moreno, P. I., and Williams, J. W.: Global climate evolution during the last deglaciation, P. Natl. Acad. Sci. USA, 109, E1134–E1142, https://doi.org/10.1073/pnas.1116619109, 2012.
Cole, K. L. and Liu, G.-W.: Holocene Paleoecology of an Estuary on Santa Rosa Island, California, Quaternary Research, 41, 326–335, https://doi.org/10.1006/qres.1994.1037, 1994.
Cole, K. L. and Wahl, E.: A Late Holocene Paleoecological Record from Torrey Pines State Reserve, California, Quaternary Res., 53, 341–351, https://doi.org/10.1006/qres.1999.2121, 2000.
Cook, E. R.: Long-Term Aridity Changes in the Western United States, Science, 306, 1015–1018, https://doi.org/10.1126/science.1102586, 2004.
Cowart, A. and Byrne, R.: A Paleolimnological Record of Late Holocene Vegetation Change from the Central California Coast, California Archaeology, 5, 337–352, https://doi.org/10.1179/1947461X13Z.00000000018, 2013.
Crawford, J. N., Mensing, S. A., Lake, F. K., and Zimmerman, S. R.: Late Holocene fire and vegetation reconstruction from the western Klamath Mountains, California, USA: A multi-disciplinary approach for examining potential human land-use impacts, The Holocene, 25, 1341–1357, https://doi.org/10.1177/0959683615584205, 2015.
Crowley, T. J.: Causes of Climate Change Over the Past 1000 Years, Science, 289, 270–277, https://doi.org/10.1126/science.289.5477.270, 2000.
Davis, O. K.: Rapid Climatic Change in Coastal Southern California Inferred from Pollen Analysis of San Joaquin Marsh, Quat. Res., 37, 89–100, https://doi.org/10.1016/0033-5894(92)90008-7, 1992.
deMenocal, P. B.: Cultural Responses to Climate Change During the Late Holocene, Science, 292, 667–673, https://doi.org/10.1126/science.1059827, 2001.
Dennison, P. E., Brewer, S. C., Arnold, J. D., and Moritz, M. A.: Large wildfire trends in the western United States, 1984–2011, Geophys. Res. Lett., 41, 2928–2933, https://doi.org/10.1002/2014GL059576, 2014.
Dettinger, M. D., Ralph, F. M., Das, T., Neiman, P. J., and Cayan, D. R.: Atmospheric Rivers, Floods and the Water Resources of California, Water, 3, 445–478, https://doi.org/10.3390/w3020445, 2011.
Di Lorenzo, E., Schneider, N., Cobb, K. M., Franks, P. J. S., Chhak, K., Miller, A. J., McWilliams, J. C., Bograd, S. J., Arango, H., Curchitser, E., Powell, T. M., and Rivière, P.: North Pacific Gyre Oscillation links ocean climate and ecosystem change, Geophys. Res. Lett., 35, L08607, https://doi.org/10.1029/2007GL032838, 2008.
Diffenbaugh, N. S. and Ashfaq, M.: Response of California Current forcing to mid-Holocene insolation and sea surface temperatures: Wind Forcing Of The California Current, Paleoceanography, 22, PA3101, https://doi.org/10.1029/2006PA001382, 2007.
Diffenbaugh, N. S., Sloan, L. C., and Snyder, M. A.: Orbital suppression of wind-driven upwelling in the California Current at 6 ka, Paleoceanography, 18, 1051, https://doi.org/10.1029/2002pa000865, 2003.
Dingemans, T., Mensing, S. A., Feakins, S. J., Kirby, M. E., and Zimmerman, S. R. H.: 3000 years of environmental change at Zaca Lake, California, USA, Front. Ecol. Evol., 34, 2, https://doi.org/10.3389/fevo.2014.00034, 2014.
Du, X., Hendy, I., and Schimmelmann, A.: A 9000-year flood history for Southern California: A revised stratigraphy of varved sediments in Santa Barbara Basin, Mar. Geol., 397, 29–42, https://doi.org/10.1016/j.margeo.2017.11.014, 2018.
Edinborough, K., Porèiæ, M., Martindale, A., Brown, T. J., Supernant, K., and Ames, K. M.: Radiocarbon test for demographic events in written and oral history, PNAS, 114, 12436–12441, 2017.
Ellis, E. C., Gauthier, N., Goldewijk, K. K., Bird, R. B., Boivin, N., Díaz, S., Fuller, D. Q., Gill, J. L., Kaplan, J. O., Kingston, N., Locke, H., McMichael, C. N. H., Ranco, D., Rick, T. C., Shaw, M. R., Stephens, L., Svenning, J.-C., and Watson, J. E. M.: People have shaped most of terrestrial nature for at least 12,000 years, PNAS, 118, 17, https://doi.org/10.1073/pnas.2023483118, 2021.
Erlandson, J. M., Graham, M. H., Bourque, B. J., Corbett, D., Estes, J. A., and Steneck, R. S.: The Kelp Highway Hypothesis: Marine Ecology, the Coastal Migration Theory, and the Peopling of the Americas, The Journal of Island and Coastal Archaeology, 2, 161–174, https://doi.org/10.1080/15564890701628612, 2007.
Erlandson, J. M., Rick, T. C., and Braje, T. J.: Fishing up the Food Web?: 12,000 Years of Maritime Subsistence and Adaptive Adjustments on California's Channel Islands, Pac. Sci., 63, 711–724, https://doi.org/10.2984/049.063.0411, 2009.
Estrella-Martínez, J., Ascough, P. L., Schöne, B. R., Scourse, J. D., and Butler, P. G.: 8.2 ka event North Sea hydrography determined by bivalve shell stable isotope geochemistry, Sci. Rep., 9, 6753, https://doi.org/10.1038/s41598-019-43219-1, 2019.
Fard, E., Brown, L. N., Lydon, S., Smol, J. P., and MacDonald, G. M.: High-resolution sedimentological and geochemical records of three marshes in San Francisco Bay, California, Quaternary Int., 602, 49–65, https://doi.org/10.1016/j.quaint.2021.05.002, 2021.
Feakins, S. J., Kirby, M. E., Cheetham, M. I., Ibarra, Y., and Zimmerman, S. R. H.: Fluctuation in leaf wax D/H ratio from a southern California lake records significant variability in isotopes in precipitation during the late Holocene, Org. Geochem., 66, 48–59, https://doi.org/10.1016/j.orggeochem.2013.10.015, 2014.
Field, D. B., Baumgartner, T. R., Charles, C. D., Ferreira-Bartrina, V., and Ohman, M. D.: Planktonic foraminifera of the California Current reflect 20th-century warming, Science, 311, 63–66, https://doi.org/10.1126/science.1116220, 2006.
Finnegan, S., Anderson, S. C., Harnik, P. G., Simpson, C., Tittensor, D. P., Byrnes, J. E., Finkel, Z. V., Lindberg, D. R., Liow, L. H., Lockwood, R., Lotze, H. K., McClain, C. R., McGuire, J. L., O'Dea, A., and Pandolfi, J. M.: Paleontological baselines for evaluating extinction risk in the modern oceans, Science, 348, 567–570, https://doi.org/10.1126/science.aaa6635, 2015.
Fisler, J. and Hendy, I. L.: California Current System response to late Holocene climate cooling in southern California, Geophys. Res. Lett., 35, L09702, https://doi.org/10.1029/2008gl033902, 2008.
Flores, C.: Importance of small-scale paleo-oceanographic conditions to interpret changes in size of California mussel (Mytilus californianus). Late Holocene, Santa Cruz island, California, Quatern. Int., 427, 137–150, https://doi.org/10.1016/j.quaint.2016.01.036, 2017.
Friddell, J. E., Thunell, R. C., Guilderson, T. P., and Kashgarian, M.: Increased northeast Pacific climatic variability during the warm middle Holocene, Geophys. Res. Lett., 30, 1560, https://doi.org/10.1029/2002GL016834, 2003.
Gardner, J. V., Heusser, L. E., Quinterno, P. J., Stone, S. M., Barron, J. A., and Poore, R. Z.: Clear Lake record vs. the adjacent marine record; A correlation of their past 20,000 years of paleoclimatic and paleoceanographic responses, in: Geological Society of America Special Papers, vol. 214, Geological Society of America, 171–182, https://doi.org/10.1130/SPE214-p171, 1988.
Gavin, D. G., Brubaker, L. B., and Lertzman, K. P.: Holocene Fire History Of A Coastal Temperate Rain Forest Based On Soil Charcoal Radiocarbon Dates, Ecology, 84, 186–201, https://doi.org/10.1890/0012-9658(2003)084[0186:HFHOAC]2.0.CO;2, 2003.
Gavin, D. G., Hallett, D. J., Hu, F. S., Lertzman, K. P., Prichard, S. J., Brown, K. J., Lynch, J. A., Bartlein, P., and Peterson, D. L.: Forest fire and climate change in western North America: insights from sediment charcoal records, Front. Ecol. Environ., 5, 499–506, https://doi.org/10.1890/060161, 2007.
Glassow, M. A., Thakar, H. B., and Kennett, D. J.: Red abalone collecting and marine water temperature during the Middle Holocene occupation of Santa Cruz Island, California, J. Archaeol. Sci., 39, 2574–2582, https://doi.org/10.1016/j.jas.2012.03.017, 2012.
Graham, M. H., Kinlan, B. P., and Grosberg, R. K.: Post-glacial redistribution and shifts in productivity of giant kelp forests, Philos. T. R. Soc. B, 399–406, https://doi.org/10.1098/rspb.2009.1664, 2010.
Grenda, D. R. and Benitez, A. V.: Continuity and change: 8,500 years of lacustrine adaptation on the shores of Lake Elsinore, Statistical Research, 1–324, 1997.
Hallett, D. J. and Anderson, R. S.: Paleofire reconstruction for high-elevation forests in the Sierra Nevada, California, with implications for wildfire synchrony and climate variability in the late Holocene, Quat. res., 73, 180–190, https://doi.org/10.1016/j.yqres.2009.11.008, 2010.
Hallett, D. J., Lepofsky, D. S., Mathewes, R. W., and Lertzman, K. P.: 11 000 years of fire history and climate in the mountain hemlock rain forests of southwestern British Columbia based on sedimentary charcoal, Can. J. For. Res., 33, 292–312, https://doi.org/10.1139/x02-177, 2003.
Hermann, N. W., Oster, J. L., and Ibarra, D. E.: Spatial patterns and driving mechanisms of mid-Holocene hydroclimate in western North America: Mid-Holocene Hydroclimate In Western North America, J. Quaternary Sci., 33, 421–434, https://doi.org/10.1002/jqs.3023, 2018.
Heusser, L.: Direct correlation of millennial-scale changes in western North American vegetation and climate with changes in the California Current System over the past ∼60 kyr, Paleoceanography, 13, 252–262, https://doi.org/10.1029/98PA00670, 1998.
Heusser, L. E.: Pollen in Santa Barbara Basin, California: A 12,000 year record, Geol. Soc. Am. Bull., 89, 673–678, 1978.
Heusser, L. E.: Palynology and paleoecology of postglacial sediments in an anoxic basin, Saanich Inlet, British Columbia, https://doi.org/10.1139/e83-077, 13, 1983.
Hickey, B. M.: The California current system–hypotheses and facts, Prog. Oceanogr., 8, 191–279, https://doi.org/10.1016/0079-6611(79)90002-8, 1979.
Higuera, P. E., Shuman, B. N., and Wolf, K. D.: Rocky Mountain subalpine forests now burning more than any time in recent millennia, PNAS, 25, 118, https://doi.org/10.1073/pnas.2103135118, 2021.
Hiner, C. A., Kirby, M. E., Bonuso, N., Patterson, W. P., Palermo, J., and Silveira, E.: Late Holocene hydroclimatic variability linked to Pacific forcing: evidence from Abbott Lake, coastal central California, J. Paleolimnol., 56, 299–313, https://doi.org/10.1007/s10933-016-9912-4, 2016.
Ingram, B. L.: Differences in Radiocarbon Age between Shell and Charcoal from a Holocene Shellmound in Northern California, Quat. Res., 49, 102–110, https://doi.org/10.1006/qres.1997.1944, 1998.
Jones, T. L. and Kennett, D. J.: Late Holocene Sea Temperatures along the Central California Coast, Quat. Res., 51, 74–82, https://doi.org/10.1006/qres.1998.2000, 1999.
Jones, T. L., Kennett, D. J., Kennett, J. P., and Codding, B. F.: Seasonal stability in Late Holocene shellfish harvesting on the central California coast, J. Archaeol. Sci., 35, 2286–2294, https://doi.org/10.1016/j.jas.2008.03.002, 2008.
Kaufman, D., McKay, N., Routson, C., Erb, M., Davis, B., Heiri, O., Jaccard, S., Tierney, J., Dätwyler, C., Axford, Y., Brussel, T., Cartapanis, O., Chase, B., Dawson, A., de Vernal, A., Engels, S., Jonkers, L., Marsicek, J., Moffa-Sánchez, P., Morrill, C., Orsi, A., Rehfeld, K., Saunders, K., Sommer, P. S., Thomas, E., Tonello, M., Tóth, M., Vachula, R., Andreev, A., Bertrand, S., Biskaborn, B., Bringué, M., Brooks, S., Caniupán, M., Chevalier, M., Cwynar, L., Emile-Geay, J., Fegyveresi, J., Feurdean, A., Finsinger, W., Fortin, M.-C., Foster, L., Fox, M., Gajewski, K., Grosjean, M., Hausmann, S., Heinrichs, M., Holmes, N., Ilyashuk, B., Ilyashuk, E., Juggins, S., Khider, D., Koinig, K., Langdon, P., Larocque-Tobler, I., Li, J., Lotter, A., Luoto, T., Mackay, A., Magyari, E., Malevich, S., Mark, B., Massaferro, J., Montade, V., Nazarova, L., Novenko, E., Paøil, P., Pearson, E., Peros, M., Pienitz, R., Płóciennik, M., Porinchu, D., Potito, A., Rees, A., Reinemann, S., Roberts, S., Rolland, N., Salonen, S., Self, A., Seppä, H., Shala, S., St-Jacques, J.-M., Stenni, B., Syrykh, L., Tarrats, P., Taylor, K., van den Bos, V., Velle, G., Wahl, E., Walker, I., Wilmshurst, J., Zhang, E., and Zhilich, S.: A global database of Holocene paleotemperature records, Sci. Data, 7, 115, https://doi.org/10.1038/s41597-020-0445-3, 2020.
Kennett, D. J. and Kennett, J. P.: Competitive and cooperative responses to climatic instability in coastal southern California, Am. Antiquity, 65, 379–395, https://doi.org/10.2307/2694065, 2000.
Kennett, D. J., Kennett, J. P., Erlandson, J. M., and Cannariato, K. G.: Human responses to Middle Holocene climate change on California's Channel Islands, Quaternary Sci. Rev., 26, 351–367, https://doi.org/10.1016/j.quascirev.2006.07.019, 2007.
Kennett, J. P. and Ingram, B. L.: A 20,000 Year Record of Ocean Circulation and Climate-Change from the Santa-Barbara Basin, Nature, 377, 510–514, https://doi.org/10.1038/377510a0, 1995.
Kim, J.-H., Rimbu, N., Lorenz, S. J., Lohmann, G., Nam, S.-I., Schouten, S., Rühlemann, C., and Schneider, R. R.: North Pacific and North Atlantic sea-surface temperature variability during the Holocene, Quaternary Sci. Rev., 23, 2141–2154, https://doi.org/10.1016/j.quascirev.2004.08.010, 2004.
Kirby, M., Lund, S., and Poulsen, C.: Hydrologic variability and the onset of modern El Niño-Southern Oscillation: A 19 250-year record from Lake Elsinore, southern California, J. Quaternary Sci., 20, 239–254, https://doi.org/10.1002/jqs.906, 2005.
Kirby, M. E., Lund, S. P., Anderson, M. A., and Bird, B. W.: Insolation forcing of Holocene climate change in Southern California: a sediment study from Lake Elsinore, J. Paleolimnol., 38, 395–417, https://doi.org/10.1007/s10933-006-9085-7, 2007.
Kirby, M. E., Lund, S. P., Patterson, W. P., Anderson, M. A., Bird, B. W., Ivanovici, L., Monarrez, P., and Nielsen, S.: A Holocene record of Pacific Decadal Oscillation (PDO)-related hydrologic variability in Southern California (Lake Elsinore, CA), J. Paleolimnol., 44, 819–839, https://doi.org/10.1007/s10933-010-9454-0, 2010.
Kirby, M. E., Zimmerman, S. R. H., Patterson, W. P., and Rivera, J. J.: A 9170-year record of decadal-to-multi-centennial scale pluvial episodes from the coastal Southwest United States: a role for atmospheric rivers?, Quaternary Sci. Rev., 46, 57–65, https://doi.org/10.1016/j.quascirev.2012.05.008, 2012.
Kirby, M. E., Feakins, S. J., Hiner, C. A., Fantozzi, J., Zimmerman, S. R. H., Dingemans, T., and Mensing, S. A.: Tropical Pacific forcing of Late-Holocene hydrologic variability in the coastal southwest United States, Quaternary Sci. Rev., 102, 27–38, https://doi.org/10.1016/j.quascirev.2014.08.005, 2014.
Kirby, M. E., Knell, E. J., Anderson, W. T., Lachniet, M. S., Palermo, J., Eeg, H., Lucero, R., Murrieta, R., Arevalo, A., Silveira, E., and Hiner, C. A.: Evidence for insolation and Pacific forcing of late glacial through Holocene climate in the Central Mojave Desert (Silver Lake, CA), Quat. Res., 84, 174–186, https://doi.org/10.1016/j.yqres.2015.07.003, 2015.
Kirby, M. E. C., Patterson, W. P., Lachniet, M., Noblet, J. A., Anderson, M. A., Nichols, K., and Avila, J.: Pacific Southwest United States Holocene Droughts and Pluvials Inferred From Sediment δ18O(calcite) and Grain Size Data (Lake Elsinore, California), Front. Earth Sci., 7, 74, https://doi.org/10.3389/feart.2019.00074, 2019.
Klimaszewski-Patterson, A., Morgan, C. T., and Mensing, S.: Identifying a Pre-Columbian Anthropocene in California, Ann. Am. Assoc. Geogr., 111, 784–794, https://doi.org/10.1080/24694452.2020.1846488, 2021.
Konrad, S. K. and Clark, D. H.: Evidence for an Early Neoglacial Glacier Advance from Rock Glaciers and Lake Sediments in the Sierra Nevada, California, U.S.A., Arctic Alpine Res., 30, 272–284, https://doi.org/10.1080/00040851.1998.12002901, 1998.
LaDochy, S., Medina, R., and Patzert, W.: Recent California climate variability: spatial and temporal patterns in temperature trends, Clim. Res., 33, 159–169, https://doi.org/10.3354/cr033159, 2007.
Leidelmeijer, J. A., Kirby, M. E. C., MacDonald, G., Carlin, J. A., Avila, J., Han, J., Nauman, B., Loyd, S., Nichols, K., and Ramezan, R.: Younger Dryas to early Holocene (12.9 to 8.1 ka) limnological and hydrological change at Barley Lake, California (northern California Coast Range), Quaternary Res., 103, 193–207, https://doi.org/10.1017/qua.2021.9, 2021.
Lightfoot, K. G. and Cuthrell, R. Q.: Anthropogenic burning and the Anthropocene in late-Holocene California, The Holocene, 25, 1581–1587, https://doi.org/10.1177/0959683615588376, 2015.
Lindström, S.: Submerged Tree Stumps as Indicators of Mid-Holocene Aridity in the Lake Tahoe Basin, Journal of California and Great Basin Anthropology, 12, 146–157, 1990.
Liu, Z., Zhu, J., Rosenthal, Y., Zhang, X., Otto-Bliesner, B. L., Timmermann, A., Smith, R. S., Lohmann, G., Zheng, W., and Timm, O. E.: The Holocene temperature conundrum, PNAS, 111, E3501–E3505, https://doi.org/10.1073/pnas.1407229111, 2014.
Lluch-Cota, D. B., Wooster, W. S., and Hare, S. R.: Sea surface temperature variability in coastal areas of the northeastern Pacific related to the El Niño-Southern Oscillation and the Pacific Decadal Oscillation, Geophys. Res. Lett., 28, 2029–2032, https://doi.org/10.1029/2000GL012429, 2001.
Long, C. J., Whitlock, C., Bartlein, P. J., and Millspaugh, S. H.: A 9000-year fire history from the Oregon Coast Range, based on a high-resolution charcoal study, Can. J. For. Res., 28, 774–787, https://doi.org/10.1139/x98-051, 1998.
Lyle, M., Heusser, L., Ravelo, C., Yamamoto, M., Barron, J., Diffenbaugh, N. S., Herbert, T., and Andreasen, D.: Out of the Tropics: The Pacific, Great Basin Lakes, and Late Pleistocene Water Cycle in the Western United States, Science, 337, 1629–1633, https://doi.org/10.1126/science.1218390, 2012.
MacDonald, G. M. and Case, R. A.: Variations in the Pacific Decadal Oscillation over the past millennium, Geophys. Res. Lett., 32, L08703, https://doi.org/10.1029/2005gl022478, 2005.
MacDonald, G. M., Moser, K. A., Bloom, A. M., Potito, A. P., Porinchu, D. F., Holmquist, J. R., Hughes, J., and Kremenetski, K. V.: Prolonged California aridity linked to climate warming and Pacific sea surface temperature, Sci. Rep., 6, 33325, https://doi.org/10.1038/srep33325, 2016.
Malamud-Roam, F. P., Lynn Ingram, B., Hughes, M., and Florsheim, J. L.: Holocene paleoclimate records from a large California estuarine system and its watershed region: linking watershed climate and bay conditions, Quaternary Sci. Rev., 25, 1570–1598, https://doi.org/10.1016/j.quascirev.2005.11.012, 2006.
Mann, M. E. and Gleick, P. H.: Climate change and California drought in the 21st century, PNAS, 112, 3858–3859, https://doi.org/10.1073/pnas.1503667112, 2015.
Mantua, N. J., Munn, T., Wiley, J., and Mantua, N. J.: Pacific–Decadal Oscillation, ISBN 0-471-97796-9, Encyclopedia of Global Environmental Change, John Wiley & Sons, Ltd, Chichester, 2002.
Marchitto, T. M., Muscheler, R., Ortiz, J. D., Carriquiry, J. D., and van Geen, A.: Dynamical Response of the Tropical Pacific Ocean to Solar Forcing During the Early Holocene, Science, 330, 1378–1381, https://doi.org/10.1126/science.1194887, 2010.
Marcott, S. A., Shakun, J. D., Clark, P. U., and Mix, A. C.: A Reconstruction of Regional and Global Temperature for the Past 11,300 Years, Science, 339, 1198–1201, https://doi.org/10.1126/science.1228026, 2013.
Marlon, J., Bartlein, P. J., and Whitlock, C.: Fire-fuel-climate linkages in the northwestern USA during the Holocene, The Holocene, 16, 1059–1071, https://doi.org/10.1177/0959683606069396, 2006.
Marlon, J. R., Bartlein, P. J., Gavin, D. G., Long, C. J., Anderson, R. S., Briles, C. E., Brown, K. J., Colombaroli, D., Hallett, D. J., Power, M. J., Scharf, E. A., and Walsh, M. K.: Long-term perspective on wildfires in the western USA, PNAS, 109, E535–E543, https://doi.org/10.1073/pnas.1112839109, 2012.
Marlon, J. R., Bartlein, P. J., Daniau, A.-L., Harrison, S. P., Maezumi, S. Y., Power, M. J., Tinner, W., and Vanniére, B.: Global biomass burning: a synthesis and review of Holocene paleofire records and their controls, Quaternary Sci. Rev., 65, 5–25, https://doi.org/10.1016/j.quascirev.2012.11.029, 2013.
Marsicek, J., Shuman, B. N., Bartlein, P. J., Shafer, S. L., and Brewer, S.: Reconciling divergent trends and millennial variations in Holocene temperatures, Nature, 554, 92–96, https://doi.org/10.1038/nature25464, 2018.
Matero, I. S. O., Gregoire, L. J., Ivanovic, R. F., Tindall, J. C., and Haywood, A. M.: The 8.2 ka cooling event caused by Laurentide ice saddle collapse, Earth Planet. Sci. Lett., 473, 205–214, https://doi.org/10.1016/j.epsl.2017.06.011, 2017.
Mayewski, P. A., Rohling, E. E., Curt Stager, J., Karlén, W., Maasch, K. A., Meeker, L. D., Meyerson, E. A., Gasse, F., van Kreveld, S., Holmgren, K., Lee-Thorp, J., Rosqvist, G., Rack, F., Staubwasser, M., Schneider, R. R., and Steig, E. J.: Holocene climate variability, Quat. Res., 62, 243–255, https://doi.org/10.1016/j.yqres.2004.07.001, 2004.
McGann, M.: High-Resolution Foraminiferal, Isotopic, and Trace Element Records from Holocene Estuarine Deposits of San Francisco Bay, California, J. Coastal Res., 245, 1092–1109, https://doi.org/10.2112/08A-0003.1, 2008.
McGann, M.: Paleoceanographic changes on the Farallon Escarpment off central California during the last 16,000 years, Quatern. Int., 235, 26–39, https://doi.org/10.1016/j.quaint.2010.09.005, 2011.
McGann, M.: Correlation of marine and coastal terrestrial records of central California: Response to paleoceanographic and paleoclimatic change during the past 19,000 years, Quatern. Int., 387, 58–71, https://doi.org/10.1016/j.quaint.2015.01.037, 2015.
McKechnie, I.: Indigenous Oral History and Settlement Archaeology in Barkley Sound, Western Vancouver Island, BC Studies, 187, https://doi.org/10.14288/bcs.v0i187.186162, 36, 2015.
McQuoid, M. R. and Hobson, L. A.: A Holocene record of diatom and silicoflagellate microfossils in sediments of Saanich Inlet, ODP Leg 169S, Mar. Geol., 174, 111–123, https://doi.org/10.1016/S0025-3227(00)00145-6, 2001.
Metcalfe, S. E.: The Holocene history of the North American Monsoon: “known knowns” and “known unknowns” in understanding its spatial and temporal complexity, 27, 2015.
Minnis, P. E.: People and plants in ancient western North America, University of Arizona Press, 492 pp., University of Arizona Press, ISBN 978-0-8165-0223-3, 2004.
Mix, A. C., Lund, D. C., Pisias, N. G., Bodén, P., Bornmalm, L., Lyle, M., and Pike, J.: Rapid climate oscillations in the Northeast Pacific during the last deglaciation reflect Northern and Southern Hemisphere sources, in: Geophysical Monograph Series, vol. 112, edited by: Clark, U., Webb, S., and Keigwin, D., American Geophysical Union, Washington, D. C., 127–148, https://doi.org/10.1029/GM112p0127, 1999.
Moffitt, S. E., Hill, T. M., Ohkushi, K., Kennett, J. P., and Behl, R. J.: Vertical oxygen minimum zone oscillations since 20 ka in Santa Barbara Basin: A benthic foraminiferal community perspective, Paleoceanography, 29, 44–57, https://doi.org/10.1002/2013pa002483, 2014.
Moffitt, S. E., Hill, T. M., Roopnarine, P. D., and Kennett, J. P.: Response of seafloor ecosystems to abrupt global climate change, P. Natl. Acad. Sci. USA, 112, 4684–4689, https://doi.org/10.1073/pnas.1417130112, 2015.
Mohr, J. A., Whitlock, C., and Skinner, C. N.: Postglacial vegetation and fire history, eastern Klamath Mountains, California, USA, The Holocene, 10, 587–601, https://doi.org/10.1191/095968300675837671, 2000.
Nederbragt, A. J. and Thurow, J. W.: A 6000 yr varve record of Holocene climate in Saanich Inlet, British Columbia, from digital sediment colour analysis of ODP Leg 169S cores, Mar. Geol., 174, 95–110, https://doi.org/10.1016/S0025-3227(00)00144-4, 2001.
Negrini, R. M., Wigand, P. E., Draucker, S., Gobalet, K., Gardner, J. K., Sutton, M. Q., and Yohe, R. M.: The Rambla highstand shoreline and the Holocene lake-level history of Tulare Lake, California, USA, Quaternary Sci. Rev., 25, 1599–1618, https://doi.org/10.1016/j.quascirev.2005.11.014, 2006.
Ohkushi, K., Kennett, J. P., Zeleski, C. M., Moffitt, S. E., Hill, T. M., Robert, C., Beaufort, L., and Behl, R. J.: Quantified intermediate water oxygenation history of the NE Pacific: A new benthic foraminiferal record from Santa Barbara basin, Paleoceanography, 28, 453–467, https://doi.org/10.1002/palo.20043, 2013.
Osborne, E. B., Thunell, R. C., Gruber, N., Feely, R. A., and Benitez-Nelson, C. R.: Decadal variability in twentieth-century ocean acidification in the California Current Ecosystem, Nat. Geosci., 13, 43–49, https://doi.org/10.1038/s41561-019-0499-z, 2020.
Oster, J. L., Sharp, W. D., Covey, A. K., Gibson, J., Rogers, B., and Mix, H.: Climate response to the 8.2 ka event in coastal California, Sci. Rep., 7, 3886, https://doi.org/10.1038/s41598-017-04215-5, 2017.
Pak, D. K., Hendy, I. L., Weaver, J. C., Schimmelmann, A., and Clayman, L.: Foraminiferal proxy response to ocean temperature variability and acidification over the last 150 years in the Santa Barbara Basin (California), Quaternary Int., 469, 141–150, https://doi.org/10.1016/j.quaint.2016.07.049, 2018.
Palmer, H. M., Hill, T. M., Roopnarine, P. D., Myhre, S. E., Reyes, K. R., and Donnenfield, J. T.: Southern California margin benthic foraminiferal assemblages record recent centennial-scale changes in oxygen minimum zone, Biogeosciences, 17, 2923–2937, https://doi.org/10.5194/bg-17-2923-2020, 2020.
Palmer, H. M., Hill, T. M., Kennedy, E. G., Roopnarine, P. D., Langlois, S., Reyes, K. R., and Stott, L. D.: Ecological and Environmental Stability in Offshore Southern California Marine Basins Through the Holocene, Paleoceanography and Paleoclimatology, 37, e2021PA004373, https://doi.org/10.1029/2021PA004373, 2022.
Pante, E. and Simon-Bouhet, B.: marmap: A Package for Importing, Plotting and Analyzing Bathymetric and Topographic Data in R, PLOS ONE, 8, e73051, https://doi.org/10.1371/journal.pone.0073051, 2013.
Pellatt, M. G., Hebda, R. J., and Mathewes, R. W.: High-resolution Holocene vegetation history and climate from Hole 1034B, ODP leg 169S, Saanich Inlet, Canada, Mar. Geol., 174, 211–226, https://doi.org/10.1016/S0025-3227(00)00151-1, 2001.
Platzman, E. and Lund, S.: High-resolution environmental magnetic study of a Holocene sedimentary record from Zaca Lake, California, The Holocene, 29, 17–25, https://doi.org/10.1177/0959683618804636, 2019.
Praetorius, S. K., Condron, A., Mix, A. C., Walczak, M. H., McKay, J. L., and Du, J.: The role of Northeast Pacific meltwater events in deglacial climate change, Sci. Adv., 6, eaay2915, https://doi.org/10.1126/sciadv.aay2915, 2020.
Rasmussen, J. B. T., Polyak, V. J., and Asmerom, Y.: Evidence for Pacific-modulated precipitation variability during the late Holocene from the southwestern USA, Geophys. Res. Lett., 33, L08701, https://doi.org/10.1029/2006GL025714, 2006.
Renssen, H., Seppä, H., Crosta, X., Goosse, H., and Roche, D. M.: Global characterization of the Holocene Thermal Maximum, Quaternary Sci. Rev., 48, 7–19, https://doi.org/10.1016/j.quascirev.2012.05.022, 2012.
Reyes, A. V. and Clague, J. J.: Stratigraphic evidence for multiple Holocene advances of Lillooet Glacier, southern Coast Mountains, British Columbia, Can. J. Earth Sci., 41, 903–918, https://doi.org/10.1139/e04-039, 2004.
Rick, T. C.: Weathering the storm: Coastal subsistence and ecological resilience on Late Holocene Santa Rosa Island, California, Quatern. Int., 239, 135–146, https://doi.org/10.1016/j.quaint.2010.06.008, 2011.
Roark, E. B., Ingram, B. L., Southon, J., and Kennett, J. P.: Holocene foraminiferal radiocarbon record of paleocirculation in the Santa Barbara Basin, Geology, 31, 379–382, https://doi.org/10.1130/0091-7613(2003)031<0379:Hfrrop>2.0.Co;2, 2003.
Routson, C. C., McKay, N. P., Kaufman, D. S., Erb, M. P., Goosse, H., Shuman, B. N., Rodysill, J. R., and Ault, T.: Mid-latitude net precipitation decreased with Arctic warming during the Holocene, Nature, 568, 83–87, https://doi.org/10.1038/s41586-019-1060-3, 2019.
Schimmelmann, A., Hendy, I. L., Dunn, L., Pak, D. K., and Lange, C. B.: Revised similar to 2000-year chronostratigraphy of partially varved marine sediment in Santa Barbara Basin, California, Gff, 135, 258–264, https://doi.org/10.1080/11035897.2013.773066, 2013.
Sheppard, P. R., Comrie, A. C., Packin, G. D., Angersbach, K., and Hughes, M. K.: The climate of the US Southwest, Nature, 21, 219–238, https://doi.org/10.3354/cr021219, 2002.
Shuman, B. N., Routson, C., McKay, N., Fritz, S., Kaufman, D., Kirby, M. E., Nolan, C., Pederson, G. T., and St-Jacques, J.-M.: Placing the Common Era in a Holocene context: millennial to centennial patterns and trends in the hydroclimate of North America over the past 2000 years, Clim. Past, 14, 665–686, https://doi.org/10.5194/cp-14-665-2018, 2018.
Shuman, J. K., Balch, J. K., Barnes, R. T., Higuera, P. E., Roos, C. I., Schwilk, D. W., Stavros, E. N., Banerjee, T., Bela, M. M., Bendix, J., Bertolino, S., Bililign, S., Bladon, K. D., Brando, P., Breidenthal, R. E., Buma, B., Calhoun, D., Carvalho, L. M. V., Cattau, M. E., Cawley, K. M., Chandra, S., Chipman, M. L., Cobian-Iñiguez, J., Conlisk, E., Coop, J. D., Cullen, A., Davis, K. T., Dayalu, A., De Sales, F., Dolman, M., Ellsworth, L. M., Franklin, S., Guiterman, C. H., Hamilton, M., Hanan, E. J., Hansen, W. D., Hantson, S., Harvey, B. J., Holz, A., Huang, T., Hurteau, M. D., Ilangakoon, N. T., Jennings, M., Jones, C., Klimaszewski-Patterson, A., Kobziar, L. N., Kominoski, J., Kosovic, B., Krawchuk, M. A., Laris, P., Leonard, J., Loria-Salazar, S. M., Lucash, M., Mahmoud, H., Margolis, E., Maxwell, T., McCarty, J. L., McWethy, D. B., Meyer, R. S., Miesel, J. R., Moser, W. K., Nagy, R. C., Niyogi, D., Palmer, H. M., Pellegrini, A., Poulter, B., Robertson, K., Rocha, A. V., Sadegh, M., Santos, F., Scordo, F., Sexton, J. O., Sharma, A. S., Smith, A. M. S., Soja, A. J., Still, C., Swetnam, T., Syphard, A. D., Tingley, M. W., Tohidi, A., Trugman, A. T., Turetsky, M., Varner, J. M., Wang, Y., Whitman, T., Yelenik, S., and Zhang, X.: Reimagine fire science for the anthropocene, PNAS Nexus, 1, pgac115, https://doi.org/10.1093/pnasnexus/pgac115, 2022.
Siedlecki, S. A., Banas, N. S., Davis, K. A., Giddings, S., Hickey, B. M., MacCready, P., Connolly, T., and Geier, S.: Seasonal and interannual oxygen variability on the Washington and Oregon continental shelves, J. Geophys. Res.-Oceans, 120, 608–633, https://doi.org/10.1002/2014JC010254, 2015.
Spaulding, W. G.: A Middle Holocene Vegetation Record from the Mojave Desert of North America and its Paleoclimatic Significance, Quat. res., 35, 427–437, https://doi.org/10.1016/0033-5894(91)90055-A, 1991.
Steinman, B. A., Pompeani, D. P., Abbott, M. B., Ortiz, J. D., Stansell, N. D., Finkenbinder, M. S., Mihindukulasooriya, L. N., and Hillman, A. L.: Oxygen isotope records of Holocene climate variability in the Pacific Northwest, Quaternary Sci. Rev., 142, 40–60, https://doi.org/10.1016/j.quascirev.2016.04.012, 2016.
Steinman, B. A., Nelson, D. B., Abbott, M. B., Stansell, N. D., Finkenbinder, M. S., and Finney, B. P.: Lake sediment records of Holocene hydroclimate and impacts of the Mount Mazama eruption, north-central Washington, USA, Quaternary Sci. Rev., 204, 17–36, https://doi.org/10.1016/j.quascirev.2018.09.018, 2019.
Stine, S.: Late holocene fluctuations of Mono Lake, eastern California, Palaeogeogr. Palaeocl., 78, 333–381, https://doi.org/10.1016/0031-0182(90)90221-R, 1990.
Stine, S.: Extreme and persistent drought in California and Patagonia during mediaeval time, Nature, 369, 546–549, https://doi.org/10.1038/369546a0, 1994.
Swain, D. L., Langenbrunner, B., Neelin, J. D., and Hall, A.: Increasing precipitation volatility in twenty-first-century California, Nature Clim. Change, 8, 427–433, https://doi.org/10.1038/s41558-018-0140-y, 2018.
Taylor, A. H., Trouet, V., Skinner, C. N., and Stephens, S.: Socioecological transitions trigger fire regime shifts and modulate fire–climate interactions in the Sierra Nevada, USA, 1600–2015 CE, P. Natl. Acad Sci. USA, 113, 13684–13689, https://doi.org/10.1073/pnas.1609775113, 2016.
Thomas, E. R., Wolff, E. W., Mulvaney, R., Steffensen, J. P., Johnsen, S. J., Arrowsmith, C., White, J. W. C., Vaughn, B., and Popp, T.: The 8.2 ka event from Greenland ice cores, Quaternary Sci. Rev., 26, 70–81, https://doi.org/10.1016/j.quascirev.2006.07.017, 2007.
Tomasovych, A. and Kidwell, S. M.: Nineteenth-century collapse of a benthic marine ecosystem on the open continental shelf, P. Roy. Soc. B-Biol. Sci., 284, 20170328, https://doi.org/10.1098/rspb.2017.0328, 2017.
Tunnicliffe, V., O'Connell, J. M., and McQuoid, M. R.: A Holocene record of marine fish remains from the Northeastern Pacific, Mar. Geol., 174, 197–210, https://doi.org/10.1016/S0025-3227(00)00150-X, 2001.
Viau, A. E., Gajewski, K., Sawada, M. C., and Fines, P.: Millennial-scale temperature variations in North America during the Holocene, J. Geophys. Res., 111, D09102, https://doi.org/10.1029/2005JD006031, 2006.
Voarintsoa, N. R. G., Matero, I. S. O., Railsback, L. B., Gregoire, L. J., Tindall, J., Sime, L., Cheng, H., Edwards, R. L., Brook, G. A., Kathayat, G., Li, X., Michel Rakotondrazafy, A. F., and Madison Razanatseheno, M. O.: Investigating the 8.2 ka event in northwestern Madagascar: Insight from data–model comparisons, Quaternary Sci. Rev., 204, 172–186, https://doi.org/10.1016/j.quascirev.2018.11.030, 2019.
Waelbroeck, C., Paul, A., Kucera, M., Rosell-Melé, A., Weinelt, M., Schneider, R., Mix, A. C., Abelmann, A., Armand, L., Bard, E., Barker, S., Barrows, T. T., Benway, H., Cacho, I., Chen, M.-T., Cortijo, E., Crosta, X., de Vernal, A., Dokken, T., Duprat, J., Elderfield, H., Eynaud, F., Gersonde, R., Hayes, A., Henry, M., Hillaire-Marcel, C., Huang, C.-C., Jansen, E., Juggins, S., Kallel, N., Kiefer, T., Kienast, M., Labeyrie, L., Leclaire, H., Londeix, L., Mangin, S., Matthiessen, J., Marret, F., Meland, M., Morey, A. E., Mulitza, S., Pflaumann, U., Pisias, N. G., Radi, T., Rochon, A., Rohling, E. J., Sbaffi, L., Schäfer-Neth, C., Solignac, S., Spero, H., Tachikawa, K., Turon, J.-L., and MARGO Project Members: Constraints on the magnitude and patterns of ocean cooling at the Last Glacial Maximum, Nature Geosci., 2, 127–132, https://doi.org/10.1038/ngeo411, 2009.
Walczak, M. H., Mix, A. C., Cowan, E. A., Fallon, S., Fifield, L. K., Alder, J. R., Du, J., Haley, B., Hobern, T., Padman, J., Praetorius, S. K., Schmittner, A., Stoner, J. S., and Zellers, S. D.: Phasing of millennial-scale climate variability in the Pacific and Atlantic Oceans, Science, 370, 716–720, https://doi.org/10.1126/science.aba7096, 2020.
Walker, M., Head, M. J., Berkelhammer, M., Björck, S., Cheng, H., Cwynar, L., Fisher, D., Gkinis, V., Long, A., Lowe, J., Newnham, R., Rasmussen, S. O., and Weiss, H.: Formal ratification of the subdivision of the Holocene Series/Epoch (Quaternary System/Period): two new Global Boundary Stratotype Sections and Points (GSSPs) and three new stages/subseries, Episodes, 41, 213–223, https://doi.org/10.18814/epiiugs/2018/018016, 2018.
Walsh, M. K., Lukens, M. L., McCutcheon, P. T., and Burtchard, G. C.: Fire-climate-human interactions during the postglacial period at Sunrise Ridge, Mount Rainier National Park, Washington (USA), Quaternary Sci. Rev., 177, 246–264, https://doi.org/10.1016/j.quascirev.2017.10.032, 2017.
Wang, Y., Hendy, I., and Napier, T. J.: Climate and Anthropogenic Controls of Coastal Deoxygenation on Interannual to Centennial Timescales, Geophys. Res. Lett., 44, 11528–11536, https://doi.org/10.1002/2017GL075443, 2017.
Wang, Y., Hendy, I. L., and Zhu, J.: Expansion of the Southern California oxygen minimum zone during the early-to mid-Holocene due to reduced ventilation of the Northeast Pacific, Quaternary Sci. Rev., 238, 106326, https://doi.org/10.1016/j.quascirev.2020.106326, 2020.
Wanner, H., Beer, J., Bütikofer, J., Crowley, T. J., Cubasch, U., Flückiger, J., Goosse, H., Grosjean, M., Joos, F., Kaplan, J. O., Küttel, M., Müller, S. A., Prentice, I. C., Solomina, O., Stocker, T. F., Tarasov, P., Wagner, M., and Widmann, M.: Mid- to Late Holocene climate change: an overview, Quaternary Sci. Rev., 27, 1791–1828, https://doi.org/10.1016/j.quascirev.2008.06.013, 2008.
Waters, C. N., Zalasiewicz, J., Summerhayes, C., Barnosky, A. D., Poirier, C., Galuszka, A., Cearreta, A., Edgeworth, M., Ellis, E. C., Ellis, M., Jeandel, C., Leinfelder, R., McNeill, J. R., Richter, D., Steffen, W., Syvitski, J., Vidas, D., Wagreich, M., Williams, M., Zhisheng, A., Grinevald, J., Odada, E., Oreskes, N., and Wolfe, A. P.: The Anthropocene is functionally and stratigraphically distinct from the Holocene, Science, 351, aad2622, https://doi.org/10.1126/science.aad2622, 2016.
Westerling, A. L., Gershunov, A., Brown, T. J., Cayan, D. R., and Dettinger, M. D.: Climate and Wildfire in the Western United States, B. Am. Meteorol. Soc., 84, 595–604, https://doi.org/10.1175/BAMS-84-5-595, 2003.
Westerling, A. L., Bryant, B. P., Preisler, H. K., Holmes, T. P., Hidalgo, H. G., Das, T., and Shrestha, S. R.: Climate change and growth scenarios for California wildfire, Clim. Change, 109, 445–463, https://doi.org/10.1007/s10584-011-0329-9, 2011.
Whitlock, C., Marlon, J., Briles, C., Brunelle, A., Long, C., and Bartlein, P.: Long-term relations among fire, fuel, and climate in the north-western US based on lake-sediment studies, Int. J. Wildland Fire, 17, 72, https://doi.org/10.1071/WF07025, 2008.
Wise, E. K.: Spatiotemporal variability of the precipitation dipole transition zone in the western United States, Geophys. Res. Lett., 37, L07706, https://doi.org/10.1029/2009GL042193, 2010.
Wise, E. K.: Five centuries of U.S. West Coast drought: Occurrence, spatial distribution, and associated atmospheric circulation patterns, Geophys. Res. Lett., 43, 4539–4546, https://doi.org/10.1002/2016GL068487, 2016.
Yati, E., Minobe, S., Mantua, N., Ito, S., and Di Lorenzo, E.: Marine Ecosystem Variations Over the North Pacific and Their Linkage to Large-Scale Climate Variability and Change, Front. Mar. Sci., 7, 578165, https://doi.org/10.3389/fmars.2020.578165, 2020.