the Creative Commons Attribution 4.0 License.
the Creative Commons Attribution 4.0 License.
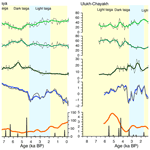
Holocene wildfire regimes in western Siberia: interaction between peatland moisture conditions and the composition of plant functional types
Angelica Feurdean
Andrei-Cosmin Diaconu
Mirjam Pfeiffer
Mariusz Gałka
Simon M. Hutchinson
Geanina Butiseaca
Natalia Gorina
Spassimir Tonkov
Aidin Niamir
Ioan Tantau
Hui Zhang
Sergey Kirpotin
Wildfire is the most common disturbance type in boreal forests and can trigger significant changes in forest composition. Waterlogging in peatlands determines the degree of tree cover and the depth of the burnt horizon associated with wildfires. However, interactions between peatland moisture, vegetation composition and flammability, and fire regime in forest and forested peatland in Eurasia remain largely unexplored, despite their huge extent in boreal regions. To address this knowledge gap, we reconstructed the Holocene fire regime, vegetation composition, and peatland hydrology at two sites located in predominantly light taiga (Pinus sylvestris Betula) with interspersed dark taiga communities (Pinus sibirica, Picea obovata, Abies sibirica) in western Siberia in the Tomsk Oblast, Russia. We found marked shifts in past water levels over the Holocene. The probability of fire occurrence and the intensification of fire frequency and severity increased at times of low water table (drier conditions), enhanced fuel dryness, and an intermediate dark-to-light taiga ratio. High water level, and thus wet peat surface conditions, prevented fires from spreading on peatland and surrounding forests. Deciduous trees (i.e. Betula) and Sphagnum were more abundant under wetter peatland conditions, and conifers and denser forests were more prevalent under drier peatland conditions. On a Holocene scale, severe fires were recorded between 7.5 and 4.5 ka with an increased proportion of dark taiga and fire avoiders (Pinus sibirica at Rybnaya and Abies sibirica at Ulukh–Chayakh) in a predominantly light taiga and fire-resister community characterised by Pinus sylvestris and lower local water level. Severe fires also occurred over the last 1.5 kyr and were associated with a declining abundance of dark taiga and fire avoiders, an expansion of fire invaders (Betula), and fluctuating water tables. These findings suggest that frequent, high-severity fires can lead to compositional and structural changes in forests when trees fail to reach reproductive maturity between fire events or where extensive forest gaps limit seed dispersal. This study also shows prolonged periods of synchronous fire activity across the sites, particularly during the early to mid-Holocene, suggesting a regional imprint of centennial- to millennial-scale Holocene climate variability on wildfire activity. Humans may have affected vegetation and fire from the Neolithic; however, increasing human presence in the region, particularly at the Ulukh–Chayakh Mire over the last 4 centuries, drastically enhanced ignitions compared to natural background levels. Frequent warm and dry spells predicted by climate change scenarios for Siberia in the future will enhance peatland drying and may convey a competitive advantage to conifer taxa. However, dry conditions will probably exacerbate the frequency and severity of wildfire, disrupt conifers' successional pathway, and accelerate shifts towards deciduous broadleaf tree cover. Furthermore, climate–disturbance–fire feedbacks will accelerate changes in the carbon balance of boreal peatlands and affect their overall future resilience to climate change.
- Article
(5676 KB) - Full-text XML
-
Supplement
(1046 KB) - BibTeX
- EndNote
Wildfire is the most common type of disturbance in boreal forests and forested peatlands (Kharuk et al., 2021 and refs therein). It can change tree community composition and accelerate climate warming via carbon release into the atmosphere and alteration of the radiative balance due to changes in land surface albedo (Rogers et al., 2015; Kharuk et al., 2021). However, the impacts of wildfire on vegetation and climate strongly depend on the fire regime. High-intensity crown fires kill most trees and alter species composition for an extended period of time. In the short term, such fires release black carbon aerosols (Rogers et al., 2015). When these aerosols persist in the atmosphere, this leads to a medium-term increase in albedo and ultimately to regional cooling. Contrastingly, surface fires typically do not kill mature trees or trigger stand-scale forest replacement and have little effect on albedo. Fire types in the Siberian boreal forests and forested peatlands are often litter-fuelled surface fires that infrequently transition to the crown, depending on forest composition (Sannikov and Goldammer, 1996; Grooth et al., 2013; Kharuk et al., 2021). A high diversity of fire-related plant functional types (PFTs), including resisters (Pinus sylvestris, Larix sibirica, L. gmelinii), avoiders (Abies sibirica, Picea obovata, Pinus sibirica), invaders (Betula pubescens, B. pendula), and endurers (Populus tremula, B. pubescens), dominate these forest communities (Rowe, 1983; Agee, 1998; Wirth, 2005). Fire resisters, invaders, and endurers are commonly associated with high-frequency but low-intensity surface fires, whereas avoiders are associated with low-frequency but high-intensity crown fires (Goldammer and Furyaev, 1996; Wirth, 2005). As these two types of fires can have a different net effect on tree community composition and climate, it is essential to understand the patterns and drivers behind each fire regime. This understanding will also improve the prediction of the impact of changes in the extent, frequency, and severity of fire in the future.
A fire regime emerges from the combination of ignition sources, climatic conditions, fuel properties, and human activities. The interactions between these factors are spatiotemporally complex (Moritz et al., 2014; Andela et al., 2017). The spatial complexity of wildfire patterns is particularly accentuated in peatlands, where the variability in the local peat moisture content, vegetation composition, and structure results in pronounced small-scale heterogeneity (Camill et al., 2009; Magnan et al., 2012; Kuosmanen et al., 2014; Remy et al., 2018; Barhoumi et al., 2019; Stivrins et al., 2019; Feurdean et al., 2020a). The waterlogged conditions that prevail in peatlands can limit the depth to which the fuel is burnt. However, this attenuation effect decreases with water table decline and increasing tree cover (Whitman et al., 2018). Unlike drained peatlands with shrub- and tree-dominated communities, well-hydrated peatlands composed of a dense Sphagnum cover remain wet despite droughts and exhibit only slight to medium fire damage (Whitman et al., 2018; Gewin, 2020). This pattern in fire dynamics is particularly concerning because climate warming accelerates woody encroachment in peatlands and increases fuel availability for peatland forest fires (Kharuk et al., 2021). Intensification of fire severity may also lead to a shift from conifer towards deciduous tree dominance (Kelly et al., 2013; Mekonnen et al., 2019). Understanding how hydrological conditions in peatlands are influenced by climate change and how this may interact with forest composition and fuel flammability is a key research priority that remains largely unexplored (Page et al., 2009; Walker et al., 2019; Kharuk et al., 2021; Dieleman et al., 2020).
Reducing uncertainty in predicting the future trajectories of fire regimes and the impact of changing fire activity on the functioning of boreal ecosystems requires approaches with a broad spatial and temporal scope (Kasischke et al., 2010). Tree-dominated ecosystems have long successional cycles ranging between decades and centuries. Palaeoecological records capture long timescales and are therefore particularly suitable to track the fire dynamics of forest ecosystems (Whitlock et al., 2018). However, studies of past millennial-scale variability in fire regimes in Siberian boreal forests, forested peatlands, and forest steppe have started to emerge only recently (Feurdean et al., 2020a; Rudaya et al., 2020; Glückler et al., 2021; Barhoumi et al., 2021; Novenko et al., 2022). Although Siberia is covered by extensive forests and forested peatlands, particularly to the west (Vompersky et al., 1994; Liss et al., 2001; Kirpotin et al., 2021), no studies have explicitly explored the interactions between fire regime, peatland moisture, and vegetation composition in this vast region.
In this study, we used multi-proxy analyses (pollen, non-pollen palynomorphs, microcharcoal, macrocharcoal morphologies, testate amoeba, geochemistry) on two new peat records from Tomsk Oblast to explore the patterns and drivers of the western Siberian fire regime throughout the Holocene. Specifically, we have explored how hydrological conditions in peatlands affect fuel dryness and how peat hydrology interacts with tree community composition with regard to plant functional types and fuel flammability in driving the frequency and type of fires. Combining our new data with our two other published records from Plotnikovo Mire (Feurdean et al., 2020a) allowed a quantification of the regional changes in biomass burning and fire frequency.
2.1 Geographical location and site selection
The study areas stretch along a west–east transect in a permafrost-free area in Tomsk Oblast, in the southern taiga of western Siberia (Bleuten and Filippov, 2008; Kirpotin et al., 2021). The region has a continental boreal climate. The mean annual temperature is 0 ∘C, the mean temperatures of the coldest and warmest season are −17 ∘C and 17 ∘C, respectively, and mean annual precipitation is ∼525 mm, falling predominantly in the warm season (https://climexp.knmi.nl, last access: 27 June 2019). Regionally, the forests are composed of both light (Pinus sylvestris, Betula pubescens, B. pendula, Populus tremula) and dark (Pinus sibirica, Picea obovata, and Abies sibirica) taiga species, the latter in greater proportions towards the east (Lashchinsky and Korolyuk, 2015). In the southern taiga of western Siberia, forests occupy 50 % of the area and peatlands 30 % with an equal proportion of forested and open peatlands (Vompersky et al., 1994; Liss et al., 2001). Our coring sites are in open peatlands surrounded by forested peatlands within a 2–6 km radius, depending on the site (Fig. 1). Fires, at present primarily ignited by humans (80 %), occur predominantly between spring and early autumn, with summer and autumn fires usually being the most severe (Kukavskaya et al., 2014, 2016; Kharuk et al., 2021).
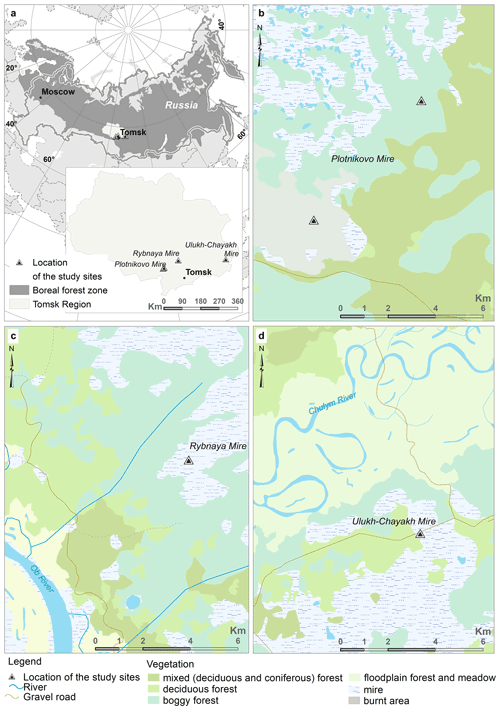
Figure 1Location of the study area in Eurasia, Russia, and the Tomsk region (a). Satellite-based images showing the location and spatial extent of vegetation types and the fire event at the previously published sites on the Plotnikovo Mire (b; Feurdean et al., 2020a) as well as the new study sites: Rybnaya (c) and Ulukh–Chayakh (d).
We cored two sites, Rybnaya Mire (57.27566800∘ N, 84.48758900∘ E) located near the Rybnaya river, a tributary of the Ob river, and Ulukh–Chayakh Mire (57.34326197∘ N, 88.32306189∘ E) located on a terrace of the Chulym river, near the village of Teguldet, about 30–40 km from the border of the Krasnoyarsk Krai (Fig. 1). The local peatland vegetation at both coring sites includes mesotrophic open-sedge Sphagnum communities with small birch and pine trees at Rybnaya Mire and standing dead Pinus tree trunks at Ulukh–Chayakh Mire. These dead trees reflect a rise in water levels across the mire due to road construction in the 1960s. Peat cores (50 cm length, 5 cm diameter) totalling 400 cm in length at Rybnaya Mire and 348 cm at Ulukh–Chayakh Mire were extracted with a Russian-type corer in 2017 and 2019, respectively.
2.2 Chronology
We established the chronology of both cores based on accelerator mass spectrometry (AMS) radiocarbon measurements performed at Isotoptech, Debrecen, Hungary (Sect. S1 in the Supplement). The 14C AMS age estimates were converted to calendar years before present (BP) using the IntCal20 dataset of Reimer et al. (2020), and we constructed the age–depth models using the smooth spline method implemented in CLAM software (Blaauw, 2010). In the age–depth models, we assigned a surface age of −67 cal yr BP (coring year 2017 for Rybnaya) and −69 cal yr BP (coring year 2019 for Ulukh–Chayakh) to the surface samples of each sequence.
2.3 Fire history
2.3.1 Charcoal-based reconstructions of fire history
We inferred changes in the local-scale fire regime based on charcoal particles identified in peat samples of 2 cm3 extracted at 1 cm contiguous intervals (Whitlock and Larsen, 2001). Preparation, identification, and categorisation of charcoal morphotypes followed Feurdean et al. (2020a) and Feurdean (2021). To deduce the predominant fire type, we pooled the charcoal morphologies into two categories: non-woody (graminoids, forbs) and woody types (deciduous leaves, wood, and resins) and calculated their ratio. These two categories represent the dominant fuel sources for surface and crown fires, respectively (Enache et al., 2006; Jensen et al., 2007; Courtney Mustaphi and Pisaric, 2014; Feurdean et al., 2017, 2020a; Feurdean, 2021). At Ulukh–Chayakh, we additionally tested the aspect ratio, length : width (L:W), of charcoal particles in selected samples to discriminate between the relative dominance of graminoids versus leaves and wood morphologies (Feurdean, 2021; Vachula et al., 2021). We grouped the charcoal fractions into two size classes (150–500 µm and >500 µm) to deduce the approximative proximity of fires, i.e. local versus on-site fire events (Adolf et al., 2018). We calculated the influx of charcoal morphotypes and size classes (particles cm−2 yr−1) by dividing their respective concentration (particles cm−3) by the sediment deposition rate determined from the age–depth models (yr cm−1).
We estimated the frequency and severity of fire episodes using charcoal peaks identified from macrocharcoal particles using the method of Higuera et al. (2009). This methodology involved interpolating CHAR values to constant time steps (30 years), decomposing the record into a background component and a peak component (local fire episodes) using a robust LOWESS smoother (900 years), and evaluating charcoal peaks using the 95th percentile of the modelled noise distribution. We used the signal-to-noise index (SNI) to assess the suitability of the record for peak detection (Kelly et al., 2011; Sect. S2).
To integrate fire history across the study area, we created composite records of CHAR and fire frequency (FF) by combining the two new records with our two published profiles from Plotnikovo Mire (Feurdean et al., 2019, 2020a; Fig. 1). Composite CHAR was created using the Power et al. (2010) protocol implemented in the R palaeofire package version 4.0 (Blarquez et al., 2014) with the following setup for Z-score transformation: 8.5 ka to present as the base period and LOWESS smoothing to a 25-year mean. The composite FF was created by pooling the identified fire events from all four records and applying a 150-year smoothing.
2.3.2 Fire type identification from satellite images and forest statistics
To evaluate the ability of charcoal records to capture the occurrence and severity of fires near the two sites, we used satellite imagery of vegetation cover from Landsat 4–5 TM, Landsat 7 ETM+, and Landsat 8 OLI/TIRS (https://earthexplorer.usgs.gov/, last access: 1 April 2021). We also used observation-based fire data from the forest department of the Tomsk region from 1950 to 2020 CE and data from MODIS sensors on board the NASA Terra-1 and Aqua-1, which detect forest fires (https://fires.ru/, last access: 30 March 2021). All maps and images were processed using ESRI ArcGIS version 10.8.
2.4 Pollen-based reconstruction of vegetation dynamics
To reconstruct past vegetation dynamics, we analysed pollen and non-pollen palynomorphs (NPPs) mainly at 4 cm intervals in both profiles following the protocol of Bennett and Willis (2001). A pollen sum exceeding 500 terrestrial pollen grains, excluding Cyperaceae, was counted for most samples and converted into percentages based on the total terrestrial pollen sum. The percentages of Cyperaceae, ferns, and mosses were calculated relative to the terrestrial pollen sum and their respective individual sum. The bottom part of the Ulukh–Chayakh profile, i.e. from 300 to 348 cm (age >6500 ka), contained extremely poorly preserved pollen and was excluded from further analysis. Microscopic charcoal particles longer than 10 µm were also counted alongside pollen, and their influx was estimated relative to the added Lycopodium marker and the sediment deposition rate.
We assigned pollen-derived tree taxa to one of the fire-related functional PFT groups – resisters (Pinus sylvestris, Larix spp.), avoiders (Picea obovata, Abies sibirica, Pinus sibirica), or invaders (Betula spp.). The two tree birch species (B. pubescens and B. pendula) and the dwarf shrub (B. nana) could not be differentiated by pollen analysis and were considered to be Betula spp. However, at present day, B. nana tends to be found only sparsely in this region. Due to its poor pollen preservation, Populus, a fire endurer, is mostly absent from our pollen records. The necessity to combine the Betula pollen signal and the paucity of pollen of Populus may have resulted in the endurer PFT group failing to be represented in our records. The PFT-specific fire-coping adaptations relate to a taxon's strategy to complete its life cycle in the context of a given fire regime (Gill, 1981; Wirth, 2005). We also separately grouped the tree pollen into taxa associated with dark taiga (Pinus sibirica, Abies sibirica, Picea obovata) and light taiga (Pinus sylvestris, Betula spp., Larix spp.) and created an index for dark-to-light taiga composition, which is an approximation of forest density, by calculating the ratio between the relative abundance of dark and light taiga tree taxa (Higuera et al., 2014). Additionally, we pooled the non-arboreal pollen types as shrubs, herbs, ferns, and mosses. To determine the regional changes in tree community composition, we created composite records of PFTs. This was done by averaging the Z scores and smoothing them to 300 years with a locally weighted regression and a 95 % confidence envelope on the same sites used for composite biomass burning reconstructions in the R palaeofire package.
2.5 Climate reconstruction based on testate amoebae and water table depth
To determine changes in peatland hydrology at each site, we used testate amoeba-based water table reconstructions. The testate amoebae preparation and identification were conducted at 4 cm intervals following the methods of Hendon and Charman (1997) and Charman et al. (2000). Based on the available literature (Grospietsch, 1958; Ogden and Hedley, 1980; Mazei and Tsyganov, 2006), we identified and counted a minimum of 150 testate amoebae per sample. No testate amoebae were present in core segments below 285 cm (age >6000 ka) in the Ulukh–Chayakh sequence. To derive the water table depth, we used two transfer functions, one developed for the pan-European region (Amesbury et al., 2016) and the other for Asia (Qin et al., 2021). The sample errors were generated using 1000 bootstrapping cycles (Line et al., 1994). The two transfer functions applied to our testate amoebae records show similar trends but different absolute water table depth values (cm). To avoid potential misinterpretation of absolute water table depth values, we followed literature recommendations (Amesbury et al., 2016; Swindles et al., 2016, 2019; Qin et al., 2021) and standardised the centimetre values and presented them as residual values (Z score). For the composite water table reconstruction, we used the same setting as for pollen.
2.6 Elemental geochemistry
The concentration of the geochemical element Ti was measured using a non-destructive Niton XL3t 900 X-ray fluorescence analyser (fpXRF) to determine the potential influence of water influx (i.e. floods) on mire water table. Sedimentary Ti NCS DC73308 was used as a Certified Reference Material (CRM). Measurements followed the procedure described by Hutchinson et al. (2016).
2.7 Numerical methods
To quantify the relationship between peatland moisture conditions, biomass burning, the relative abundances of PFTs, and dark-to-light taiga composition, we performed a correlation analysis. We used microcharcoal (CHAR particles <150 µm) and macrocharcoal (CHAR particles >150 µm) influx to determine regional and local biomass burning, pollen percentages as indicators of PFTs (resisters, invaders, avoiders, and others) at local to regional scales, and testate-amoebae-based water table depth as a proxy for local peatland moisture. The group “others” includes pollen and spores of shrubs, herbs, ferns, and mosses. Before the analysis, we interpolated all datasets fed into the model to a 100-year interval using linear interpolation.
3.1 Chronology
The age–depth model of the Rybnaya sequence spans ∼ the last 8400 years and shows a mean peat accumulation rate of 25 yr cm−1 (ranging between 6 and 36 yr cm−1). The Ulukh–Chayakh sequence may cover ∼ the last 8500 years, but the chronology of the bottom part of this site (>6000 years) relies on linear extrapolation and is therefore highly uncertain (Sect. S1). The subsequence covering the last 6000 years has an average temporal resolution of 21 yr cm−1 (ranging between 3 and 37 yr cm−1).
3.2 Fire type identification from charcoal, satellite images, and forest statistics
The comparison of sedimentary charcoal from sub-recent samples with satellite imagery of the Plotnikovo Mire (sites SP and SD) confirmed that the marked increase in charcoal particles towards the present day at the SD location closely agrees with the occurrence of high-severity fires (Fig. 1; Feurdean et al., 2020a). Satellite images and official forest statistics show no evidence of recent fires in the vicinity of the Rybnaya and Ulukh–Chayakh sites, except for a small fire documented in 1993 near the Ulukh–Chayakh site (Fig. 1). This aligns with the scarcity of charcoal pieces from subsurface samples in both cores (Fig. 2; Sect. S3). A high-severity fire event occurred in 2015 ca. 30 km from Ulukh–Chayakh, which is not documented in our subsurface charcoal record and corroborates the localised origin of charcoal found in this sedimentary profile. Samples from the Ulukh–Chayakh site show a minor increase in charcoal (2–7 pieces; up to 0.6 cm−2 yr−1) ca. 50 years ago, possibly related to a local fire event in 1993. The first clear charcoal peak at this site (250 pieces; 7.3 cm−2 yr−1), with numerous wood fragments, was found ca. 200 years ago, whereas the preceding charcoal peak (400 pieces; 9.2 cm−2 yr−1) was ca. 900 years ago (Fig. 2; Sect. S3). These two peaks therefore indicate the occurrence of infrequent, high-severity local fires that produce large quantities of charcoal, embedded in what is otherwise predominately a surface-fire-generated low level of charcoal.
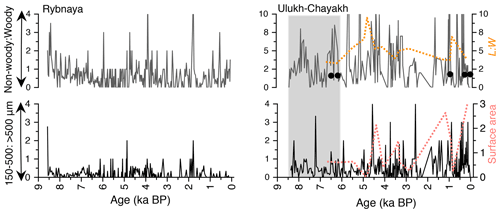
Figure 2The ratio of charcoal morphotype influx (particles cm−2 yr−1) of the two main categories: woody (wood, deciduous leaves, resins) and non-woody type (forbs, grass) in the Rybnaya and Ulukh–Chayakh sequences. The aspect ratio (L:W) at Ulukh–Chayakh is used for fuel type identification. A high L:W ratio is typical for a higher abundance of graminoid charcoal, whereas a lower L:W ratio is typical for charcoal from wood and leaves. The ratio of charcoal influx (particles cm−2 yr−1) of the two main size classes is as follows: small 150–500 µm and large >500 µm in the two sequences and the charcoal surface area (µm2) at Ulukh–Chayakh (see Sect. S3 for a full range of size classes). Bullets at Ulukh–Chayakh represent the extra-large charcoal fraction (>1000 µm) identified during routine plant macrofossil analysis of sediment volumes of ca. 20 cm3. The grey time window highlights the period with an uncertain chronology. Note that some high-amplitude charcoal peaks have been truncated to enhance the visibility of long-term trends in charcoal values.
3.3 Site-specific and composite record of biomass burning and fire frequency
Both records displayed a high signal-to-noise index (Sect. S2) with almost all peaks well above 3 (a mean of 4.98 at Rybnaya and 6.45 at Ulukh–Chayakh), the theoretical minimum value for justification of peak analysis (Kelly et al., 2011). At Rybnaya, CHAR was highest between 7.5 and 6 ka and around 4.5 ka, whereas the frequency of high-severity fires inferred from the charcoal peak magnitude and abundance of woody morphologies ranged between one and two fires per 900 years (Figs. 2, 4; Sects. S2, S3). At Ulukh–Chayakh, CHAR was highest >6 and 3.5 ka, and the fire frequency varied between two and five fires per 900 years. Both profiles showed a decline towards a minimum in CHAR and frequency of severe fires, i.e. low peak magnitude and reduced occurrence of woody charcoal morphologies, approximately between 4 and 1.5 ka (3–1.5 ka at Ulukh–Chayakh). The CHAR increased towards the present at both sites, but the charcoal peak amplitude was considerably higher at Ulukh–Chayakh.
The composite CHAR record showed augmented regional biomass burning between 7.5 and 4 ka (positive Z scores) with peaks centred at 7.5 and 4.5 ka (Fig. 5). We found a pattern of CHAR decline towards a minimum in the composite profiles between 4 and 2 ka (negative Z scores), followed by another increase between 2 ka and the present (positive Z scores). The composite fire frequency showed an increase until ca. 5–4.5 ka and then again from 2 ka to the present.
3.4 Site-specific and composite record of vegetation composition and forest density
Early Holocene data are only available from the Rybnaya site (8.5 and 7.5 ka), indicating that light taiga and the fire invader Betula were most abundant (up to 60 %) during that period, whereas other light taiga fire resisters (Pinus sylvestris) and dark taiga fire avoiders (mainly Picea obovata) had an abundance of 10 % and 5 %, respectively (Fig. 3; Sect. S4a). The high proportion of light taiga resulted in a low dark-to-light taiga ratio of 0.1 and perhaps a low forest density. An increased dark-to-light taiga ratio (>0.2) was identified between 7.5 and 4 ka at Rybnaya and between 5 and 2.5 ka at Ulukh–Chayakh (Fig. 5; Sect. S4a, b). The light taiga was composed of up to 70 % fire resisters (Pinus sylvestris and Larix spp.), while the dark taiga, with its fire avoiders (Pinus sibirica, Picea obovata, and Abies sibirica), made up 25 % of the forest. A decline in fire resisters (down to 50 %), avoiders (below 10 %), and dark-to-light taiga ratio as well as increasing invader abundance (up to 60 %) occurred after 4.5 ka at Rybnaya and after 2.5 ka at Ulukh–Chayakh. Between-site variability was highest within the avoider group. Picea obovata had higher values at Rybnaya (up to 10 %) than at Ulukh–Chayakh (3 %), whereas Abies sibirica was more abundant at Ulukh–Chayakh (15 %) than at Rybnaya (7 %; Fig. 4). The composite vegetation record showed a higher proportion of fire resisters between 7.5 and 5 ka, fire avoiders between 7.5 and 3 ka, and fire invaders over the last 4.5 kyr (Fig. 5).
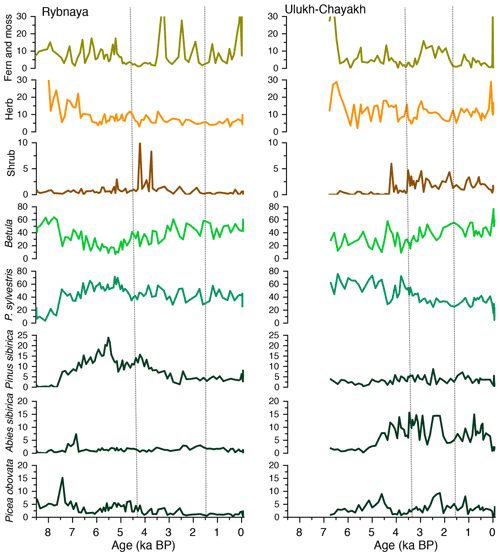
Figure 3Temporal trends in the pollen and spore percentages of individual tree taxa and lumped group-wise for shrub, herb, fern, and moss at Rybnaya and Ulukh–Chayakh. Periods with substantial changes in fire regime are indicated by the dotted vertical lines.
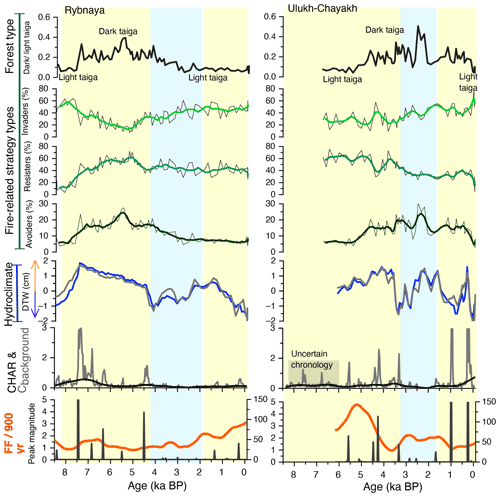
Figure 4Temporal trends in the pollen percentages of the fire-related plant functional groups (invaders, resisters, and avoiders) and the forest type determined from the ratio between pollen percentages of dark and light taiga tree taxa in the Rybnaya and Ulukh–Chayakh sequences. Hydrological conditions of the peatlands were derived from testate-amoeba-based estimates of the water table depth using the European transfer function (blue) and the Asia transfer function (grey); positive Z-score values represent greater-than-mean water table (deep water table), and negative Z-score values represent lower-than-mean water level (shallow water table). The fire metrics include burned biomass (CHAR), fire frequency (number of fires per 900 years), and charcoal peak magnitude (the higher the values, the greater the fire severity and/or the closer to the site) derived from macrocharcoal particles >150 µm. Colours in the background denote periods with marked changes in fire regime and vegetation composition; a change from blue to yellow indicates an intensification of the fire episodes.
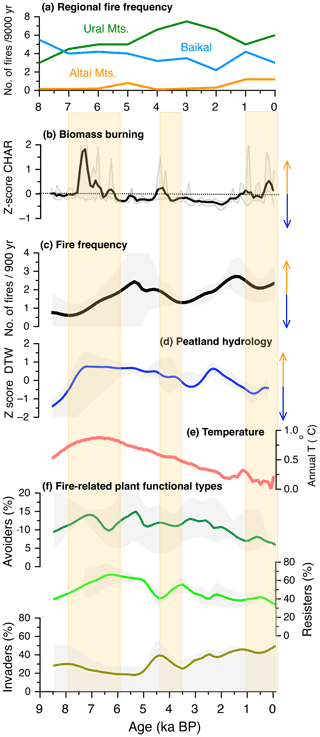
Figure 5Charcoal-based fire frequency at locations in Pinus–Betula- dominated boreal forests in Russia: northern Ural region (Barhoumi et al., 2019), Lake Baikal (Barhoumi et al., 2021), and the western Siberian Plain (Rudaya et al., 2020) (a). Composite record of biomass burning (n=4) based on Z-score charcoal influx; positive and negative Z-score values represent greater-than-mean and lower-than-mean charcoal influx over the base period (b). Composite record of fire frequency (n=4) (c). Composite record of peatland hydrology (n=4) from testate amoeba; positive and negative Z-score values represent lower- and higher-than-mean water level, respectively (d). Annual temperature (anomalies) for 30–60∘ N (Kaufman et al., 2020) (e). Composite record (n=4) of the relative abundance of avoiders, resisters, and invaders determined from pollen percentages (f). Grey curves represent confidence intervals. Colours highlight the periods with higher biomass burning in the composite record.
Understory vegetation primarily reflects local peatland development. It was predominantly composed of herbs–sedges (Cyperaceae; up to 20 %), ferns (Polypodiaceae, up to 20 %), and Equisetum (up to 25 %) at both sites until ca. 4.5 ka. An increase in the abundance of shrubs (Ericaceae; up to 10 %) and moss (Sphagnum; highly fluctuating between 10 % and 100 %) at Rybnaya as well as Ericaceae (up to 5 %) and moss (Sphagnum; up to 100 %) at Ulukh–Chayakh was noted over the last 4.5 kyr (Fig. 3; Sect. S4a, b). The sum and individual levels of herbaceous taxa, including those potentially related to human impact (Artemisia, Asteraceae, Chenopodiaceae, Urticaceae, Brassicaceae, Plantago lanceolata), were constant throughout the profiles. However, their abundance increased slightly over the last 1.5 kyr (Fig. 3; Sect. S4a, b). Coprophilous dung spores, Podospora and Sporormiela, were present throughout both records, although they became more abundant between 4.5 and 3.5 ka and between 3.2 and 1.0 ka at Ulukh–Chayakh as well as over the past millennium at Rybnaya (Sect. S4a, b).
3.5 Site-specific and composite records of peatland moisture conditions
The two testate amoebae transfer functions returned similar trends for the water table depth, but the absolute values (cm) were different; the Asian transfer function yielded higher reconstructed water tables than the European transfer function (Fig. 4; Sect. S5). At Rybnaya, the standardised values of water table depth indicated relatively high levels (negative Z score) between 8.5 and 7.5 ka, between 4 and 2.5 ka, and over the last 1 kyr (Fig. 4). We reconstructed low water levels (positive Z score) for the time interval between 7.5 and 4.5 ka and between 2.5 and 1 ka (Fig. 4). At Ulukh–Chayakh, the water level was high (negative Z score) between 3.5 and 2.5 ka, between 2 and 0.5 ka, and towards the present day. There, times of low water levels (positive Z score) occurred between 6 and 3.5 ka, between 2.5 and 2 ka, and around 0.5 ka. The composite record of the water table showed high levels at approximately 8.5–7.5, 3.5, and 3 ka as well as during the last 1.5 kyr and low levels between 7.5 and 4.5 ka as well as around 2.5 ka (Fig. 5).
3.6 Numerical analysis
The correlation analysis at Rybnaya showed that microcharcoal and macrocharcoal were highly correlated and positively associated with the proportion of fire avoiders and light-to-dark taiga index as well as negatively with resisters and invaders (Fig. 6a). However, not all these relationships were statically significant (Fig. A1). At Ulukh–Chayakh, microcharcoal and macrocharcoal were weakly correlated. Microcharcoal was positively associated with fire avoiders, resisters, others, and light-to-dark taiga index and negatively with invaders (mostly statically significant; Fig. 6a, b). All other relationships, i.e. between PFTs and macrocharcoal as well as depth to the water table, were statistically non-significant (Figs. 6a, A1).
4.1 Changes in fuel, fire type, and fire frequency over the Holocene
Stand-replacing fires combust substantial amounts of biomass because they burn entire trees. Such fires manifest themselves in a greater abundance of charcoal particles that exceed the quantities typically produced by surface fires (van Marle et al., 2017). This feature allows a distinct separation of charcoal peaks from charcoal background levels, which yields a higher signal-to-noise index (Higuera et al., 2005, 2009; Courtney Mustaphi and Pisaric, 2014). The comparison of sedimentary charcoal from sub-recent samples with satellite imagery of the Plotnikovo Mire (Feurdean et al., 2020a), Rybnaya, and Ulukh–Chayakh shows that high-severity local fires produce large quantities of charcoal; thus, the charcoal peak extraction method applied here reliably reflects the occurrence of such fires.
We reason that the prevalence of a high charcoal influx with well-defined charcoal peaks and abundant woody morphotypes found between ca. 7.5 and 4(3) ka at both sites, as well as over the past 1.5 kyr at Ulukh–Chayakh, is indicative of the predominance of high-severity local fires (Fig. 2; Sect. S3). Between 4(3) and 1.5 ka, the observed low charcoal influx and peak magnitude were coupled with a lower abundance of woody morphologies at both sites, although the latter feature was more pronounced at Ulukh–Chayakh (Fig. 2; Sect. S3). For this period, we inferred predominately low-temperature surface fires that mostly burned understory biomass. Experimental production of charcoal additionally provides evidence that graminoids and Sphagnum retain a high charred mass only during low-temperature fires and that their charred mass declined rapidly with increasing fire temperature (Hudspith et al., 2018; Feurdean, 2021). Measurements of aspect ratio (L:W) and surface area on selected charcoal samples at Ulukh–Chayakh show that the aspect ratio only partially agrees with the predominant fuel type inferred from charcoal morphologies. A lower aspect ratio of charcoal particles, which is typical for wood and leaf morphotypes, was what we expected at times of increased woody morphologies and an increased aspect ratio with a rise in the relative proportion of graminoids (Feurdean, 2021; Vachula et al., 2021). This is probably the result of a strongly mixed morphology of charcoal types in the same sample (Fig. 2). Our measurements of charcoal surface area indicate high values at times of an increased abundance of leaves (Fig. 2; Sect. S3) with a typically high surface area (Crawford and Belcher, 2014; Feurdean, 2021).
The reconstructed fire return interval is ∼450 years (two fires per 900 years) for both sites throughout most of the Holocene (Fig. 4). However, fire frequency (at Rybnaya) and severity (at Ulukh–Chayakh) increased over the last 1.5–2 kyr compared to the long-term averages of both records, suggesting an intensification of fire activity during the most recent 2 millennia. The composite fire frequency derived from all four records also shows increasing biomass burning and fire frequency over the last 2–1.5 kyr (Fig. 5). The reconstructed fire return interval (FRI) in the study area is largely in line with other sites located in forested peatlands (Pinus–Betula-dominated, Picea obovata, Abies sibirica) in Russia, which report an FRI range between 100 and 600 years (Barhoumi et al., 2019, 2020, 2021). In contrast to a study in the northern Ural region (Barhoumi et al., 2019), we have not found a gradual increase in fire frequency from the early Holocene towards the present, but rather two distinct periods of enhanced activity between 7.5 and 4 ka and approximately over the last 2 kyr (Fig. 5). This is a pattern more similar to sites in the western Siberian Plain (Rudaya et al., 2020) and Lake Baikal area (Barhoumi et al., 2021).
4.2 Drivers of vegetation and fire regime change
Climate conditions influence peatland hydrology, fuel dryness, and flammability, as well as vegetation composition with regard to moisture- and fire-related PFTs. The relative abundances of the fire-related PFTs, in turn, influence the prevalence of specific fire regime types, e.g. low-intensity vs. high-intensity regimes. Below, we discuss how hydrological conditions in peatlands affect fuel dryness and flammability as well as feedback between tree community composition (PFTs) and fire regime.
The influence of peatland moisture on fuel type and flammability
Waterlogging in peatlands and the occurrence of a dense Sphagnum cushion can limit fire severity and reduce the depth of the burnt horizon. Moreover, a waterlogged horizon provides a substrate that serves as a seedbed for post-fire regeneration (Whitman et al., 2018; Gewin, 2020). However, increasingly dry peatland conditions and consequently greater tree cover can reduce the limiting effect of waterlogging on fire severity (Magnan et al., 2012; Kettridge et al., 2015; Whitman et al., 2018, 2019; Loisel et al., 2021). Deep burns can even smoulder over winter and re-ignite the following spring (Scholten et al., 2021). We found that the probability of fire occurrence and the intensification of fire severity were greater at times of low water level (drier peatland conditions) and decreased at high water levels (wetter peatland conditions; Figs. 4, 6). Wet peat surface conditions likely prevented the fire from spreading on the peatland and in the surrounding forests, but also the propagation of fires from the surrounding forests across the peatland. The stronger association between peatland moisture conditions and burning at Rybnaya may be related to the higher amplitude of changes in local hydrological conditions and the overall drier conditions at this site compared to Ulukh–Chayakh (Fig. 4; Sect. S5).
Water levels of mesotrophic mires are co-regulated by the balance between precipitation (P) and evapotranspiration (ET) as well as external water inflow (Chambers and Charman, 2004). The detrital element Ti, a possible indicator of water influx in minerogenic mires, increased notably between 4 and 3 ka and between 1.5 and 0.1 ka at Ulukh–Chayakh but only slightly over the last 1 kyr at Rybnaya (Sect. S5). This suggests increasing fluvial input, reflecting possible flooding or channel position change as a transport mechanism for the delivery of such material at Ulukh–Chayakh (Sect. S5; Leshchinskiy et al., 2011). Mire type development and changes in peat plant communities could have also influenced peatland hydrology (Gałka et al., 2016; Kurina and Li, 2019; Blyakharchuk and Kurina, 2021). At Rybnaya, the minerogenic stage of the mire occurred from 8 to 4.5 ka and the water level was more stable. With the meso-oligotrophisation of the mire, indicated by the appearance of Ericaceae at 4.5 ka and the dominance of Sphagnum from 3.6 ka to the present, the water level and the amplitude of its fluctuation rose and the occurrence of peat fires declined (Figs. 3, 4; Sect. S4a, b). At the Ulukh–Chayakh site, Ericaceae developed at 4.5 ka and remained dominant up to the present, whereas Sphagnum established around 2 ka and became dominant only in the past decades (Fig. S4 in the Supplement). Further, mire margins with thinner peat depth burn more frequently than thicker peat (Turunen et al., 2001). Thus, the location of the Ulukh–Chayakh sampling point close to the mineral margin of the mire (ca. 160 m), as opposed to a few kilometres at Rybnaya, could have sustained a high fire occurrence at this site until recently. Lastly, fire itself can influence peatland hydrology, with field measurements indicating an age-dependent water table lowering after burning (Holden et al., 2015).
At a Holocene scale, the intensity of fires and/or fire size was greatest between 7.5 and 6.5 ka and between 5 and 4 ka, but the timing was not fully synchronous between the sites (Figs. 4, 5). From 9 to 4.5 ka, annual temperatures in the Northern Hemisphere were up to 3.5 ∘C warmer than at present (Fig. 5) and exhibited a more pronounced seasonality, i.e. higher summer and lower winter temperatures (Kaufman et al., 2020; Bova et al., 2021). Pollen-based climate reconstructions in southern Siberia show warmer- and drier-than-present climate conditions in the early Holocene (until 8 ka), a continuous increase in moisture conditions to the present, and a temperature and moisture optimum approximately between 6 and 4.5 ka (Borisova et al., 2011; Groisman et al., 2012; Zhang and Feng, 2018). A review of multi-proxy palaeohydrological conditions along a west to east gradient in southern Siberia shows hydrological conditions strikingly similar to ours for the western part, i.e. moist between ca. 8.1 and 7.4 ka, followed by dry conditions between ca. 7.4 and 5.0 ka, associated with a diminished North Atlantic influence (Mikhailova et al., 2021). Testate-amoebae-based hydrological reconstructions in the southern taiga of western Siberia, however, show considerable temporal variability in peat moisture conditions between sites and only a coeval shift to dry conditions between 7.0 and 6.0 ka (Kurina et al., 2018), primarily linked to declining precipitation.
The interval of lowest biomass burning and fire severity approximately between 4 (3) and 1.5 ka coincides with one of the wettest peatland conditions during the Holocene at both sites (4.5–2.5 ka) followed by mostly dry peatland conditions (Fig. 4). Annual and summer temperatures declined after 4.5 ka in the Northern Hemisphere (Kaufman et al., 2020; Bova et al., 2021), and moisture was high for most of the past 4.5 kyr in the southern part of western Siberia (Zhang and Feng, 2018; Mikhailova et al., 2021). Testate-amoebae-based hydrological reconstructions in the southern taiga of western Siberia, however, show long periods with dry conditions over the past 4.5 kyr, with short-term wet spells occurring at 2.6–2.3 ka, 1.3–1.1 ka, and 0.9–0.2 ka (Kurina et al., 2018) or over the past 2.5 kyr (Blyakharchuk and Kurina, 2021). Although biomass burning and the frequency of severe fires show more centennial-scale variability over the past 1.5 kyr, this matches known climatic fluctuations only partially, such as the Medieval Climate Anomaly (MCA; 1.2–0.65 ka) and the Little Ice Age (LIA; 0.7–0.4 ka). Notably, an increased charcoal peak amplitude occurred during the second part of the LIA (0.4–0.2 ka) at a time of extremely wet conditions (Fig. 4; Feurdean et al., 2019). We hypothesise that the climatic linkage between the cool and wet conditions, which implies high fuel moisture conditions and typically low fire activity, was likely disrupted by anthropogenic activity towards the present day (Fig. 3; Sect. S4a, b), as documented by other pollen records in the region (Blyakharchuk et al., 2019). Historical records indicate the widespread colonisation of the region by Russia (i.e. from the west) at this time, associated with the conversion of forest to arable land and pastures, as well as likely increased anthropogenic ignitions (Naumov, 2006). Most recently, local fire suppression near the village of Teguldet (near Ulukh–Chayakh mire) may have hampered contemporary fire spread on the mire, leading to the recent pattern of low fire activity.
Nevertheless, humans may have altered the frequency of fires earlier than the widespread Russian colonisation of the region (Marlon et al., 2016). A GIS survey of archaeological finds in the Tomsk region reveals a high density of sites but no distinctions between the cultural phases (Zolnikov et al., 2020). In parallel with modern settlement distribution, most of these ancient sites were situated near rivers favouring the floodplain terraces of river valleys (Zolnikov et al., 2020). Another study in the lower Tom Basin attests to the presence of tools that have the characteristics of sites commonly associated with the Neolithic and Bronze Age close to Rybnaya (Idimeshev et al., 2018). There is little evidence of pollen indicative of human impact at Rybnaya until 400 years ago, but coprophilous-based evidence of herbivores (Podospora) is present from 7 ka onward and increased over the past 400 years, whereas fire activity was high from 7 to 5 ka (Figs. 4, S4). At the Ulukh–Chayakh site, coprophilous spores became more abundant between 4.5 and 3.5 ka (Sporormiella) and between 3.2 and 1.0 ka (Podospora and Sporormiella), while fire activity increased between 4 and 3 ka and over the last millennium. However, it is difficult to say from our records whether the coprophilous remains originated from wild or domesticated animals and how much herbivores contributed to the opening of the forest and facilitated the fire spread (Morales-Molino et al., 2019). Given the locations of our sites near rivers, the archaeological evidence suggests the possibility of some level of human impact on vegetation and fire.
4.3 Feedbacks between the PFT composition of vegetation and fire regime
Climate conditions affect the relative abundances of PFTs. Fire-related PFTs, in turn, create positive or negative feedbacks for fire regimes via specific fire-related traits (Feurdean et al., 2020a; Kharuk et al., 2021). We found a dominance of open light taiga and fire-invader species (Betula spp.) between 8.5 and 7.5 ka and over the past 4.5 kyr. The occurrence of Betula-dominated forests coincides with marked variability in the frequency and severity of fire, i.e. moderate between 8.5 and 7.5 ka, low between 4.5 and 1.5 ka, and high over the past 1.5 kyr (Figs. 2, 4). B. pubescens grows on wet peatlands, whereas B. pendula grows more frequently on drier sandy soils and peatlands with higher fire incidence (Wirth, 2005; Groisman et al., 2012; Blyakharchuk, 2003). The prevalence of birch species with contrasting hydrological and fire requirements (unable to be differentiated by pollen) may explain such variable association of Betula and fire regime. Denser forests of light taiga and fire resisters (Pinus sylvestris), interspaced with dark taiga and fire avoiders, primarily Pinus sibirica at Rybnaya and Abies sibirica at Ulukh Chayakh (only from 5.5 ka), prevailed between 7.5 and 4.5 ka (Fig. 3; Sect. S4a, b). This forest composition coincides with increased fire severity. Visual trends and correlation reveal that biomass burning increases at intermediate dark-to-light taiga index values (ranging from 0.15 to 0.3; Figs. 4, 6). This is consistent with emerging findings pointing to a higher fire hazard in less dense as opposed to denser boreal forests, which allow fuel to dry (Scheffer et al., 2012; Feurdean et al., 2020b). The dominance of dark taiga forests in western Siberia was associated with increased moisture conditions (Blyakharchuk and Sulerzhitsky, 1999; Zhang and Feng, 2018; Barhoumi et al., 2021). Our correlations, however, suggest a greater tolerance of deciduous trees to shallow water level (wetter conditions) compared to conifers (Fig. 6a, b). Note that testate amoebae and macrocharcoal reveal local changes in peatland hydrology and fire activity, whereas pollen and microcharcoal reflect local to regional changes in vegetation composition (the tree composition on the peatlands and adjacent forests) and fire activity (Bennet and Willis, 2001; Whitlock and Larsen, 2001; Lamentowicz et al., 2015; Blyakharchuk and Kurina, 2021). The deviating scale dependency of the reconstructions may induce some uncertainty in linking the local peat moisture conditions and the dominant local to regional PFTs.
The presence of the fire resisters (i.e. Pinus sylvestris) in Siberia is associated with a light surface fire regime (mean FRI of 28 years) that is occasionally interrupted by longer-term (mean FRI of 200 years) stand-replacing crown fire events (Ivanova, 2005; Kukavskaya et al., 2016). Fire avoiders typically experience severe stand-replacing crown fires at relatively long return intervals (mean FRI 150 years; range 99 and 300 years; Goldammer and Furyaev, 1996; Wirth, 2005; Kharuk et al., 2021). The post-fire regeneration pathway of conifer species involves recruitment directly after burning (P. sylvestris) or after an early successional phase initiated by Betula (Pinus sibirica, Picea obovata, and Abies sibirica). A mixed taiga forest with deciduous trees and dark taiga conifer taxa typically develops between 60 and 120 years after a stand-replacing fire, followed by the dominance of conifers as a late successional stage (Tautenhahn et al., 2016; Coop et al., 2020; Kharuk et al., 2021). We reason that the prevailing periodicity of severe fires (every 180 to 450 years) between 7.5 and 4.5 ka provided fire-free periods that were long enough for the fire avoiders Pinus sibirica, Picea obovata, and Abies sibirica to reach reproductive age. Alternatively, burning may have been too patchy to create sufficiently large forest gaps to limit seed dispersal and inhibit post-fire recovery. Pinus sibirica is typically classified as a fire avoider but behaves like a fire resister when reaching old age, which could also explain its increased presence at times of high fire activity. Fire also allows dark taiga species to compete with P. sylvestris and become established beyond poor soils and boggy areas (Kharuk et al., 2021).
A different fire regime and altered vegetation feedbacks emerged over the last 2 millennia at Ulukh–Chayakh, the site that experienced more severe fire episodes. The intensification of local fire severity at Ulukh–Chayakh paralleled a decline in the proportion of fire avoiders (mostly Abies sibirica and Picea obovata), an increase in fire invaders (Betula), and the abundant occurrence of heathland shrubs (Figs. 3, 4). Shrubs burn hotter than other surface fuel types and reach temperatures that kill most conifer seedlings (Tautenhahn et al., 2016). High-severity burned areas have also been found to be more prone to repeated burning and have a more negative impact on conifers (Kukavskaya et al., 2016; Whitman et al., 2019; Coop et al., 2020). We propose that the intensification in fire severity at Ulukh–Chayakh may have eliminated mature Abies sibirica and Picea obovata, limited their seed dispersal, and disrupted their successional pathways. These dynamics resulted in a shift in tree community composition towards more post-fire-adapted invader communities with better dispersal capabilities, recruitment strategies for burned areas, and rapidly maturing taxa. Our results support emerging findings of increases in deciduous trees (Betula or Populus) at the expense of evergreen conifers that are associated with contemporary warming and increasing fire severity in boreal forests and forested peatlands in Siberia and North America (Kelly et al., 2013; Tautenhahn et al., 2016; Mekkonen et al., 2019; Whitman et al., 2019; Kharuk et al., 2021). At Rybnaya, the modest increase in biomass burning over the past 2 millennia coincided with wet peatland conditions, the occurrence of Sphagnum, and a forest composition that remained dominated by Betula, indicating that fire has played a more limited role in tree community dynamics at this site. The post-disturbance (fire, insect outbreak) forest regeneration pathway in Siberia also involves Populus tremula. Eurasian aspen forest communities can reach hundreds of years in age and change forest composition to Populus dominance where regeneration of dark taiga conifers is absent (Tautenhahn et al., 2016; Kharuk et al., 2021). Although we have not encountered Populus in our pollen record due to its poor pollen preservation, the possibility that Populus may have been a part of post-fire forest succession pathways in the past cannot be discounted.
This study provides novel insights into past fire regimes based on Holocene records from western Siberia. It demonstrates that peatland hydrology is a critical factor of fuel dryness, peat plant composition, fire-related plant functional types, and fire regime. We found that the probability of fire occurrence and intensification of fire frequency and severity increased at times of low water table (drier conditions), enhanced fuel dryness and flammability, and an intermediate dark-to-light taiga ratio. Wet peatland conditions promoted deciduous trees typically classified as light taiga taxa and fire invaders (Betula), whereas dry peatland conditions facilitated conifers and therefore a high dark-to-light taiga forest ratio.
At a Holocene timescale, we found enhanced fire severity between 7.5 and 4.5 ka associated with a higher proportion of dark taiga and fire-avoider taxa (mainly Pinus sibirica at Rybnaya and Abies sibirica at Ulukh Chayakh) in a light-taiga- and fire-resister-dominant (Pinus sylvestris) community along with drier peatland conditions. The second period of increased fire severity (i.e. last ca. 1.5 kyr) coincided with a reduced abundance of fire avoiders (mostly Abies sibirica and Picea obovata) and an expansion of fire invaders (Betula). These community changes demonstrate that frequent fires of higher severity can lead to compositional changes in forest tree communities, either because the trees were unable to reach their reproductive age between burning events, or the fires created substantial forest gaps that hindered seed dispersal. This study also shows certain prolonged periods of synchronous fire activity across the sites, suggesting that the magnitude of centennial- to millennial-scale climate variability over the Holocene was marked enough to drive fire regimes on a regional scale.
Based on our findings from fossil records, the frequent warm and dry spells predicted by climate change scenarios in Siberia for the future (IPCC, 2021) will likely enhance peatland drying and may convey a competitive advantage to some conifers, especially Pinus sylvestris. But dry peatland conditions will also exacerbate the likelihood of greater fire frequency, increase fire severity (i.e. stand-replacing fires), and likely disrupt the successional pathway of typical taiga conifers, notably that of fire avoiders. Such a fire regime change may accelerate a shift towards communities dominated by deciduous fire-invader-type trees. Future climate–disturbance–fire feedbacks will not only accelerate changes in the boreal forest structure and composition, but also impact the carbon balance of the forested peatlands and ultimately lead to albedo-mediated feedbacks on the regional climate system.
All data generated for this paper will be deposited in the Neotoma database following publication.
The supplement related to this article is available online at: https://doi.org/10.5194/cp-18-1255-2022-supplement.
AF was responsible for the methodology. AF, ACD, MG, SMH, GB, NG, ST, IT, and SK contributed to investigation and writing. AF prepared the paper with contributions from MP and SMH. Editing was conducted by ACD, MP, MG, GB, NG, SMH, ST, AN, IO, and SK. Funding acquisition was handled by AF.
The contact author has declared that neither they nor their co-authors have any competing interests.
Publisher's note: Copernicus Publications remains neutral with regard to jurisdictional claims in published maps and institutional affiliations.
We thank Rosa Rytkönen, University of Manchester, for her help during fieldwork and the reviewers for useful comments to improve the paper. Sergey Loiko also provided archaeological literature from the region.
This research was supported by the German Research Foundation (grant no. FE-1096/6-1). Sergey Kirpotin was supported by the Russian Science Foundation (grant no. 20-67-46018) and the Tomsk State University Development Programme (Priority-2030). Publisher's note: the article processing charges for this publication were not paid by a Russian or Belarusian institution.
This open-access publication was funded by the Goethe University Frankfurt.
This paper was edited by Nathalie Combourieu Nebout and reviewed by three anonymous referees.
Adolf, C., Wunderle, S., Colombaroli, D., Weber, H., Gobet, E., Heiri, O., van Leeuwen, J. F., Bigler, C., Connor, S. E., Gałka, M., La Mantia, T., and Tinner, W.: The sedimentary and remote sensing reflection of biomass burning in Europe, Global Ecol. Biogeogr., 27, 199–212, https://doi.org/10.1111/geb.12682, 2018.
Agee, J. K.: Fire and Pine Ecosystems, in: Ecology and Biogeography of Pinus, edited by: Richardson, D. M., Cambridge Univ Press, Cambridge, 193–218, ISBN: 0521551765 9780521551762, 1998.
Amesbury, M. J., Swindles, G. T., Bobrov, A., Charman, D. J., Holden, J., Lamentowicz, M., Mallon, G., Mazei, Y., Mitchell, E. A. D., Payne, R. J., Roland, T. P., Turner, T. E., and Warner, B. G.: Development of a new pan-European testate amoeba transfer function for reconstructing peatland palaeohydrology, Quaternary Sci. Rev., 152, 132–151, https://doi.org/10.1016/j.quascirev.2016.09.024, 2018.
Andela, N., Morton, D. C., Giglio, L., Chen, Y., van der Werf, G. R., Kasibhatla, P. S., DeFries, R. S., Collatz, G. J., Hantson, S., Kloster, S., Bachelet, D., Forrest, M., Lasslop, G., Li, F., Mangeon, S., Melton, J. R., Yue, C., and Randerson, J. T.: A human-driven decline in 10 global burned area, Science, 356, 1356–1362, https://doi.org/10.1126/science.aal4108, 2017.
Barhoumi, C., Peyron, O., Joannin, S., Subetto, D., Kryshen, A., Drobyshev, I., Girardin, M. P., Brossier, B., Paradis, L., Pastor, T., and Alleaume, S.: Gradually increasing forest fire activity during the Holocene in the northern Ural region (Komi Republic, Russia), The Holocene, 29, 1906–1920, https://doi.org/10.1177/0959683619865593, 2019.
Barhoumi, C., Ali, A. A., Peyron, O., Dugerdil, L., Borisova, O., Golubeva, Y., Subetto, D., Kryshen, A., Drobyshev, I., Ryzhkova, N., and Joannin, S.: Did long-term fire control the coniferous boreal forest composition of the northern Ural region (Komi Republic, Russia), J. Biogeogr., 47, 2426–2441, https://doi.org/10.1111/jbi.13922, 2020.
Barhoumi, C., Vogel, M., Dugerdil, L., Limani, H., Joannin, S., Peyron, O., and Ali, A. A.: Holocene Fire Regime Changes in the Southern Lake Baikal Region Influenced by Climate-Vegetation-Anthropogenic Activity Interactions, Forests, 12, 978, https://doi.org/10.3390/f12080978, 2021.
Bennett, K. D. and Willis, K. J.: Pollen, in: Tracking Environmental Change Using Lake Sediments, edited by: Smol, J. S., Birks, H. J. B., and Last, W. M., Kluwer Academic Publishers, Dordrecht, 5–32, https://doi.org/10.1007/0-306-47668-1_2, 2001.
Blaauw, M.: Methods and code for `classical' age-modelling of radiocarbon sequences, Quat. Geochronol., 5, 512–518, https://doi.org/10.1016/j.quageo.2010.01.002, 2010.
Blarquez, O., Vannière, B., Marlon, J. R., Daniau, A.-L., Power, M. J., Brewer, S., and Bartlein, P. J.: Paleofire An R package to analyse sedimentary charcoal records from the Global Charcoal Database to reconstruct past biomass burning, Comput. Geosci., 72, 255–261, https://doi.org/10.1016/j.cageo.2014.07.020, 2014.
Bleuten, W. and Filippov, I.: Hydrology of mire ecosystems in central West Siberia: the Mukhrino field station, edited by: Glagolev, M. V. and Lapshina, E. D., Transactions of UNESCO Department of Yugorsky State University Environmental Dynamics and Global Climate Change, 1, 208–224, https://doi.org/10.17816/edgcc11S208-224, 2008.
Blyakharchuk, T. A.: Four new pollen sections tracing the Holocene vegetational development of the southern part of the West Siberan Lowland. The Holocene 13, 715–731. https://doi.org/10.1191/0959683603hl658rp, 2003.
Blyakharchuk, T. A. and Kurina, I.: Late Holocene environmental and climatic changes in the Western Sayan Mountains based on high-resolution multi-proxy data, Boreas, 50, 919–934, https://doi.org/10.1111/bor.12493, 2021.
Blyakharchuk, T. A. and Sulerzhitsky, L. D.: Holocene vegetational and climatic changes in the forest zone of Western Siberia according to pollen records from the extrazonal palsa bog Bugristoe, The Holocene, 9, 621–628, https://doi.org/10.1191/095968399676614561, 1999.
Blyakharchuk, T. A., Kurina, I. V., and Pologova, N. N.: Late-Holocene dynamics of vegetation cover and humidity of climate in the southeastern sector of the West Siberian Plain according to the data of palynological and rhizopod research of peat deposits, Tomsk State University Journal of Biology, 45, 164–189, https://doi.org/10.17223/19988591/44/9, 2019 (in Russian, English Summary).
Borisova, O. K., Novenko, E. Y., Zelikson, E. M., and Kremenetski, K. V.: Lateglacial and Holocene vegetational and climatic changes in the southern taiga zone of West Siberia according to pollen records from Zhukovskoye peat mire, Quatern. Int., 237, 65–73, https://doi.org/10.1016/j.quaint.2011.01.015, 2011.
Bova, S., Rosenthal, Y., Liu, Z., Godat, S. P., and Yan, M.: Seasonal origin of the thermal maxima at the Holocene and the last interglacial, Nature, 589, 548–553, https://doi.org/10.1038/s41586-020-03155-x, 2021.
Camill, P., Barry, A., Williams, E., Andreassi, C., Limmer, J., and Solick, D.: Climate vegetation–fire interactions and their impact on long-term carbon dynamics in a boreal peatland landscape in northern Manitoba, Canada, J. Geophys. Res., 114, G04017, https://doi.org/10.1029/2009JG001071, 2009.
Chambers, F. M. and Charman, D. J.: Holocene environmental change: contributions from the peatland archive, The Holocene, 14, 1–6, https://doi.org/10.1191/0959683604hl684ed, 2004.
Charman, D. J., Hendon, D., and Woodland, W. A.: The Identification of Testate Amoebae (Protozoa: Rhizopoda) in Peats, Technical Guide No. 9. Quaternary Research Association, London, ISBN 0907780482, 2000.
Coop, J. D., Parks, S. A., Stevens-Rumann, C. S., Crausbay, S. D., Higuera, P. E., Hurteau, M. D., Tepley, A., Whitman, E., Assal, T., Collins, B. M., and Davis, K. T.: Wildfire-driven forest conversion in western North American landscapes, BioScience, 70, 659–73, https://doi.org/10.1093/biosci/biaa061, 2020.
Courtney Mustaphi, C. J. and Pisaric, M. F.: A classification for macroscopic charcoal morphologies found in Holocene lacustrine sediments, Prog. Phys. Geog., 38, 734–754, https://doi.org/10.1177/0309133314548886, 2014.
Crawford, A. J. and Belcher, C. M.: Charcoal morphometry for paleoecological analysis: the effects of fuel type and transportation on morphological parameters, Appl. Plant Sci., 2, 1400004, https://doi.org/10.3732/apps.1400004, 2014.
Dieleman, C. M., Rogers, B. M., Potter, S., Veraverbeke, S., Johnstone, J. F., Laflamme, J., Solvik, K., Walker, X. J., Mack, M. C., and Turetsky, M. R.: Wildfire combustion and carbon stocks in the southern Canadian boreal forest: implications for a warming world, Global Change Biol., 26, 6062–6079, https://doi.org/10.1111/gcb.15158, 2020.
Enache, M. D. and Cumming, B. F.: Tracking recorded fires using charcoal morphology from the sedimentary sequence of Prosser Lake, British Columbia (Canada), Quaternary Res., 65, 282–292, https://doi.org/10.1016/j.yqres.2005.09.003, 2006.
Feurdean, A.: Experimental production of charcoal morphologies to discriminate fuel source and fire type: an example from Siberian taiga, Biogeosciences, 18, 3805–3821, https://doi.org/10.5194/bg-18-3805-2021, 2021.
Feurdean, A., Veski, S., Florescu, G., Vannière, B., Pfeiffer, M., O'Hara, R. B., Stivrins, N., Amon, L., Heinsalu, A., Vassiljev, J., and Hickler, T.: Broadleaf deciduous forest counterbalanced the direct effect of climate on Holocene fire regime in hemiboreal/boreal region (NE Europe), Quaternary Sci. Rev., 169, 378–390, https://doi.org/10.1016/j.quascirev.2017.05.024, 2017.
Feurdean, A., Gałka, M., Tantau, I., Florescu, G., Hutchinson, S. M., Diaconu, A., and Kirpotin, S.: 2000 years of variability in hydroclimate and carbon accumulation in western Siberia and the relationship with large scale atmospheric circulation: A multiproxy peat record, Quaternary Sci. Rev., 226, 105948, https://doi.org/10.1016/j.quascirev.2019.105948, 2019.
Feurdean, A., Florescu, G., Tanţău, I., Vannière, B., Diaconu, A. C., Pfeiffer, M., Warren, D., Hutchinson, S. M., Gorina, N., Gałka, M., and Kirpotin, S.: Recent fire regime in the southern boreal forests of western Siberia is unprecedented in the last five millennia, Quaternary Sci. Rev., 244, 106495, https://doi.org/10.1016/j.quascirev.2020.106495, 2020a.
Feurdean, A., Vannière, B., Finsinger, W., Warren, D., Connor, S. C., Forrest, M., Liakka, J., Panait, A., Werner, C., Andrič, M., Bobek, P., Carter, V. A., Davis, B., Diaconu, A.-C., Dietze, E., Feeser, I., Florescu, G., Gałka, M., Giesecke, T., Jahns, S., Jamrichová, E., Kajukało, K., Kaplan, J., Karpińska-Kołaczek, M., Kołaczek, P., Kuneš, P., Kupriyanov, D., Lamentowicz, M., Lemmen, C., Magyari, E. K., Marcisz, K., Marinova, E., Niamir, A., Novenko, E., Obremska, M., Pędziszewska, A., Pfeiffer, M., Poska, A., Rösch, M., Słowiński, M., Stančikaitė, M., Szal, M., Święta-Musznicka, J., Tanţău, I., Theuerkauf, M., Tonkov, S., Valkó, O., Vassiljev, J., Veski, S., Vincze, I., Wacnik, A., Wiethold, J., and Hickler, T.: Fire hazard modulation by long-term dynamics in land cover and dominant forest type in eastern and central Europe, Biogeosciences, 17, 1213–1230, https://doi.org/10.5194/bg-17-1213-2020, 2020b.
Gałka, M., Tanţău, I., Ersek, V., and Feurdean, A.: A 9000 year record of cyclic vegetation changes identified in a montane peatland deposit located in the Eastern Carpathians (Central-Eastern Europe): Autogenic succession or regional climatic influences?, Palaeogeogr. Palaeocl., 449, 52–61, https://doi.org/10.1016/j.palaeo.2016.02.007, 2016.
Gewin, B.: How peat could protect the planet, Nature, 578, 204–208, https://doi.org/10.1038/d41586-020-00355-3, 2020.
Gill, A. M.: Fire adaptive traits of vascular plants, in: Fire regimes and ecosystem properties: proceedings of the conference, Honolulu, Hawaii, 11–15 December 1978, U.S. Forest Service, Washington, WO-26, 208–230, 1981.
Glückler, R., Herzschuh, U., Kruse, S., Andreev, A., Vyse, S. A., Winkler, B., Biskaborn, B. K., Pestryakova, L., and Dietze, E.: Wildfire history of the boreal forest of south-western Yakutia (Siberia) over the last two millennia documented by a lake-sediment charcoal record, Biogeosciences, 18, 4185–4209, https://doi.org/10.5194/bg-18-4185-2021, 2021.
Goldammer, J. G. and Furyaev, V. V.: Fire in Ecosystems of Boreal Eurasia: Ecological Impacts and Links to the Global System, in: Fire in Ecosystems of Boreal Eurasia. Forestry Sciences, vol. 48, edited by: Goldammer, J. G. and Furyaev, V. V., Springer, Dordrecht, https://doi.org/10.1007/978-94-015-8737-2_1, 1996.
Groisman, P. Y., Blyakharchuk, T. A., Chernokulsky, A. V., Arzhanov, M. M., Marchesini, L. B., Bogdanova, E. G., Borzenkova, II, Bulygina, O. N., Karpenko, A. A., Karpenko, L. V., and Knight, R. W.: Climate changes in Siberia, in: Regional environmental changes in Siberia and their global consequences, edited by: Groisman, P. Ya. and Gutman, G., Springer, Dordrecht, https://doi.org/10.1007/978-94-007-4569-8, 2012.
Grooth, W. J., Cantin, A. S., Flannigan, M. D., Soja, A. J., Gowman, L. M., and Newbery, A.: A comparison of Canadian and Russian boreal forest fie regimes, Forest Ecol. Manag.,, 294, 23–34, https://doi.org/10.1016/j.foreco.2012.07.033, 2013.
Grospietsch, T.: Wechseltierchen (Rhizopoden), Kosmos Verlag, Stuttgart, ISBN B0000BIT21, 1958.
Hendon, D. and Charman, D. J.: The preparation of testate amoebae (Protozoa: Rhizopoda) samples from peat, Holocene, 7, 199–205, https://doi.org/10.1177/095968369700700207, 1997.
Higuera, P., Brubaker, L., Anderson, P., Hu, F., and Brown, T.: Vegetation mediated the impacts of postglacial climate change on fire regimes in the south-central Brooks Range, Alaska, Ecol. Monogr., 79, 201–219, https://doi.org/10.1890/07-2019.12009, 2009.
Higuera, P. E., Sprugel, D. G., and Brubaker, L. B.: Reconstructing fire regimes with charcoal from small-hollow sediments: a calibration with tree-ring records of fire, The Holocene, 15, 238–251, https://doi.org/10.1191/0959683605hl789rp, 2005.
Higuera, P. E., Briles, C. E., and Whitlock, C.: Fire-regime complacency and sensitivity to centennial-through millennial-scale climate change in Rocky Mountain subalpine forests, Colorado, USA, J. Ecol., 102, 1429–1441, https://doi.org/10.1111/1365-2745.12296, 2014.
Holden, J., Palmer, S. M., Johnston, K., Wearing, C., Irvine, B., and Brown, L. E.: Impact of prescribed burning on blanket peat hydrology, Water Resour. Res., 51, 6472–6484, https://doi.org/10.1002/2014WR016782, 2015.
Hudspith, V. A., Hadden, R. M., Bartlett, A. I., and Belcher, C. M.: Does fuel type influence the amount of charcoal produced in wildfires? Implications for the fossil record, Palaeontology, 61, 159–171, https://doi.org/10.1111/pala.12341, 2018.
Hutchinson, S. M., Akinyemi, F. O., Mîndrescu, M., Begy, R., and Feurdean, A.: Recent sediment accumulation rates in contrasting lakes in the Carpathians (Romania): impacts of shifts in socio-economic regime, Reg. Environ. Change, 16, 501–513, https://doi.org/10.1007/s10113-015-0764-7, 2016.
Idimeshev, A. A., Bychkov, D. A., and Asochakova, E. M.: Stone industry of Samuska III settlement based on the results of the statistic analysis, Tomsk Journal of Linguistics and Anthropology, 3, 115–127, https://doi.org/10.23951/2307-6119-2020-3-115-127, 2020.
IPCC: Summary for Policymakers, in: Climate Change 2021: The Physical Science Basis. Contribution of Working Group I to the Sixth Assessment Report of the Intergovernmental Panel on Climate Change, edited by: Masson-Delmotte, V., Zhai, P., Pirani, A., Connors, S. L., Péan, C., Berger, S., Caud, N., Chen, Y., Goldfarb, L., Gomis, M. I., Huang, M., Leitzell, K., Lonnoy, E., Matthews, J. B. R., Maycock, T. K., Waterfield, T., Yelekçi, O., Yu. R., and Zhou, B., Cambridge University Press, Cambridge, United Kingdom and New York, NY, USA, 3–32, https://doi.org/10.1017/9781009157896.001, 2021.
Ivanova, G. A.: Vegetation zone-specific characteristics of Scots pine forest fires in central Siberia, PhD thesis, V.N. Sukachev Institute of Forest Publishing, Krasnoyarsk, Russia, 2005 (in Russian).
Jensen, K., Lynch, E., Calcote, R., and Hotchkiss, S. C.: Interpretation of charcoal morphotypes in sediments from Ferry Lake, Wisconsin, USA: do different plant fuel sources produce distinctive charcoal morphotypes?, Holocene, 17, 907– 915, https://doi.org/10.1177/0959683607082405, 2007.
Kaufman, D., McKay, N., Routson, C., Erb, M., Dätwyler, C., Sommer, P. S., Heiri, O., and Davis, B.: Holocene global mean surface temperature, a multi-method reconstruction approach, Scientific Data, 7, 201, https://doi.org/10.1038/s41597-020-0530-7, 2020.
Kasischke, E. S., Verbyla, D. L., Rupp, T. S., McGuire, A. D., Murphy, K. A., Randi, J., Barnes, J. L., Hoy, E. E., Duffy, P. A., Calef, M., and Turetsky, M. R.: Alaska’s changing fire regime–implications for the vulnerability of its boreal forests, Can. J. Forest Res., 40, 1313–1324, https://doi.org/10.1139/X10-098, 2010.
Kelly, R., Chipman, M. L., Higuera, P. E., Stefanova, I., Brubaker, L. B., and Hu, F. S.: Recent burning of boreal forests exceeds fire regime limits of the past 10,000 years, P. Natl. Acad. Sci. USA, 110, 13055–13060, https://doi.org/10.1073/pnas.1305069110, 2013.
Kelly, R. F., Higuera, P. E., Barrett, C. M., and Hu, F. S.: A signal-to-noise index to quantify the potential for peak detection in sediment-charcoal records, Quaternary Res., 75, 11–17, https://doi.org/10.1016/j.yqres.2010.07.011, 2011.
Kettridge, N., Turetsky, M., Sherwood, J. H., Thompson, D. K., Miller, C. A., Benscoter, B. W., Flannigan, M. D., Wotton, B. M., and Waddington, J. M.: Moderate drop in water table increases peatland vulnerability to post-fire regime shift, Scientific Reports, 5, 8063, https://doi.org/10.1038/srep08063, 2015.
Kharuk, V. I., Ponomarev, E. I., Ivanova, G. A., Dvinskaya, M. L., Coogan, S. C., and Flannigan, M. D.: Wildfires in the Siberian taiga, Ambio, 50, pages 1953–1974, https://doi.org/10.1007/s13280-020-01490-x, 2021.
Kirpotin, S. N., Antoshkina, O. A., Berezin, A. E., Elshehawi, S., Feurdean, A., Lapshina, E. D., Pokrovsky, O. S., Peregon, A. M., Semenova, N. M., Tanneberger, F., and Volkov, I. V.: Great Vasyugan Mire: How the world's largest peatland helps addressing the world's largest problems, Ambio, 50, 2038–2049, https://doi.org/10.1007/s13280-021-01520-2, 2021.
Kukavskaya, E. A., Ivanova, G. A., Conard, S. G., McRae, D. J., and Ivanov, V. A.: Biomass dynamics of central Siberian Scots pine forests following surface fires of varying severity, Int. J. Wildland Fire, 23, 872–886, https://doi.org/10.1071/WF13043, 2014.
Kukavskaya, E. A., Buryak, L. V., Shvetsov, E. G., Conard, S. G., and Kalenskaya, O. P.: The impact of increasing fire frequency on forest transformations in southern Siberia, Forest Ecol. Manag., 382, 225–235, https://doi.org/10.1016/j.foreco.2016.10.015, 2016.
Kuosmanen, N., Fang, K., Bradshaw, R. H., Clear, J. L., and Seppä, H.: Role of forest fires in Holocene stand-scale dynamics in the unmanaged taiga forest of northwestern Russia, The Holocene 24, 1503–1514, https://doi.org/10.1177/0959683614544065, 2014.
Kurina, I. V. and Li, H.: Why Do Testate Amoeba Optima Related to Water Table Depth Vary?, Microb. Ecol., 77, 37–55, https://doi.org/10.1007/s00248-018-1202-4, 2019.
Kurina, I. V., Veretennikova, E. E., Golovatskaya, E. A., Blyakharchuk, T. A., and Smirnov, S. V.: Dynamics of the surface wetness of mires in the southern taiga subzone of Western Siberia in the middle and late Holocene. Tomsk State University Journal of Biology, 42, 218–241, https://doi.org/10.17223/19988591/42/12, 2018.
Lashchinsky, N. N. and Korolyuk, A. Yu.: Syntaxonomy of zonal dark-coniferous forests of southern taiga of the West Siberian plain and of humid low-mountains of the Altai-Sayan mounain region, Vegetation of Russia, 26, 85–107, https://doi.org/10.31111/vegrus/2015.26.85, 2015.
Lamentowicz, M., Słowinski, M., Marcisz, K., Zielinska, M., Kaliszan, K., Lapshina, E., Gilbert, D., Buttler, A., Fiałkiewicz-Kozieł, B., Jassey, V. E., and Laggoun-Defarge, F.: Hydrological dynamics and fire history of the last 1300 years in western Siberia reconstructed from a high-resolution, ombrotrophic peat archive, Quaternary Res., 84, 312–325, https://doi.org/10.1016/j.yqres.2015.09.002, 2015.
Leshchinskiy, S. V., Blyakharchuk, T. A., Vvedenskaya, I. A., and Orlova, L. A.: The first terrace above the Ob’ floodplain near Kolpashevo: the age and formation conditions, Russ. Geol. Geophys., 52, 641–649, https://doi.org/10.1016/j.rgg.2011.05.007, 2011.
Line, J. M., ter Braak, C. J. F., and Birks, H. J. B.: WACALIB version 3.3 – a computer program to reconstruct environmental variables from fossil assemblages by weighted averaging and to derive sample-specific errors of prediction, J. Paleolimnol., 10, 147–152, 1994.
Liss, O. L., Abramova, L. I., Avetov, N. A., Berezina, N. A., Inisheva, L. I., Kurnishkova, T. V., Sluka, Z. A., Tolpycheva, T. Yu., and Shvedchikova, N. K.: Mire systems of Western Siberia and their environmental importance, Grifi K Publisher, Tula, 2001 (in Russian).
Loisel, J., Gallego-Sala, A. V., Amesbury, M. J., Magnan, G., Anshari, G., Beilman, D. W., Benavides, J. C., Blewett, J., Camill, P., Charman, D. J., Chawchai, S., Hedgpeth, A., Kleinen, T., Korhola, A., Large, D., Mansilla, C., Müller, J., van Bellen, S., West, J. B., Yu, Z., Bubier, J. L., Garneau, M., Moore, T., Britta, A., Sannel, K., Page, S., Väliranta, M., Bechtold,M., Brovkin, V., Cole, L. E. S., Chanton, J. P., Christensen, T. R., Davies, M. A., De Vleeschouwer, F., Finkelstein, S. A., Frolking, S., Gałka, M., Gandois, L., Girkin, N., Harris, L. I., Heinemeyer, A., Hoyt, A. M., Jones, M.-C., Joos, F., Juutinen, S., Kaiser, K., Lacourse, T., Lamentowicz, M., Larmola, T., Leifeld, L., Lohila, A., Milner, A. M., Minkkinen, K., Moss, P., Naafs, B. D. A., Nichols, J., O'donnell, J., Payne, R., Philben, M., Piilo, S., Quillet, A., Ratnayake, A. S., Roland, T. P., Sjögersten, S., Sonnentag, O., Swindles, G. T., Swinnen, W., Talbot, J., Treat, C., Valach, A. C., and Wu, J.: Expert assessment of future vulnerability of the global peatland carbon sink, Nat. Clim. Change, 11, 70–77, https://doi.org/10.1038/s41558-020-00944-0, 2021.
Magnan, G., Lavoie, M., and Payette, S.: Impact of fire on long-term vegetation dynamics of ombrotrophic peatlands in northwestern Quebec, Canada, Quaternary Res., 77, 110–121, https://doi.org/10.1016/j.yqres.2011.10.006, 2012.
Marlon, J. R., Kelly, R., Daniau, A.-L., Vannière, B., Power, M. J., Bartlein, P., Higuera, P., Blarquez, O., Brewer, S., Brücher, T., Feurdean, A., Romera, G. G., Iglesias, V., Maezumi, S. Y., Magi, B., Courtney Mustaphi, C. J., and Zhihai, T.: Reconstructions of biomass burning from sediment-charcoal records to improve data–model comparisons, Biogeosciences, 13, 3225–3244, https://doi.org/10.5194/bg-13-3225-2016, 2016.
Mazei, Y. and Tsyganov, A.: Freshwater Testate Amoebae, KMK, Moscow, 2006.
Mekonnen, Z. A., Riley, W. J., Randerson, J. T., Grant, R. F, and Rogers, B. M.: Expansion of high-latitude deciduous forests driven by interactions between climate warming and fire, Nat. Plants, 5, 952–958, https://doi.org/10.1038/s41477-019-0495-8, 2019.
Mikhailova, A. B., Grenaderova, A. V., Kurina, I.V ., Shumilovskikh, L., and Stojko, T. G.: Holocene vegetation and hydroclimate changes in the Kansk forest steppe, Yenisei River Basin, East Siberia, Boreas, 50, 948–966, https://doi.org/10.1111/bor.12542, 2021.
Morales-Molino, C., Tinner, W., Perea, R., Carrión, J. S., Colombaroli, D., Valbuena-Carabaña, M., Zafra, E., and Gil, L.: Unprecedented herbivory threatens rear-edge populations of Betula in southwestern Eurasia, Ecology, 100, e02833, https://doi.org/10.1002/ecy.2833, 2019.
Moritz, M. A., Batllori, E., Bradstock, R. A., Gill, A. M., Handmer, J., Hessburg, P. F., Leonard, J., McCaffrey, S., Odion, D. C., Schoennagel, T., and Syphard, A. D.: Learning to coexist with wildfire, Nature, 515, 58–66, https://doi.org/10.1038/nature13946, 2014.
Naumov, I. V.: The history of Siberia, Routledge, London, New York, https://doi.org/10.4324/9780203027981, 2006.
Novenko, E. Y., Kupryanov, D. A., Mazei, N. G., Prokushkin, A., Phelps, L. N., Buri, A., and Davis, B. A.: Evidence that modern fires may be unprecedented during the last 3400 years in permafrost zone of central Siberia, Russia, Environ. Res. Lett., 17, 025004, https://doi.org/10.1088/1748-9326/ac4b53, 2022.
Ogden, C. G. and Hedley, R. H.: An Atlas of Freshwater Testate Amoebae, Oxford University Press, London, https://doi.org/10.1111/j.1550-7408.1980.tb04269.x, 1980.
Page, S., Hoscilo, A., Langner, A., Tansey, K., Siegert, F., Limin S., and Rieley, J.: Tropical peatland fires in Southeast Asia, in: Tropical Fire Ecology, edited by: Cochrane, M. A., Springer Praxis Books, Springer, Berlin, Heidelberg, https://doi.org/10.1007/978-3-540-77381-8_9, 2009.
Power, M. J., Marlon, J. R., Bartlein, P. J., and Harrison, S. P.: Fire history and the Global Charcoal Database: a new tool for hypothesis testing and data exploration, Palaeogeogr. Palaeocl., 291, 52–59, https://doi.org/10.1016/j.palaeo.2009.09.014, 2010.
Qin, Y., Li, H., Mazei, Y., Kurina, I., Swindles, G. T., Bobrov, A., Tsyganov, A. N., Gu, Y., Huang, X., Xue, J., Lamentowicz, L., Marcisz, K., Roland, T., Payne, R., Mitchell, E. A. M., and Xie, S.: Developing a continental-scale testate amoeba hydrological transfer function for Asian peatlands, Quaternary Sci. Rev., 258, 106868, https://doi.org/10.1016/j.quascirev.2021.106868, 2021.
Reimer, P., Austin, W., Bard, E., Bayliss, A., Blackwell, P., Bronk Ramsey, C., and Talamo, S.: The IntCal20 Northern Hemisphere Radiocarbon Age Calibration Curve (0–55 cal kBP), Radiocarbon, 62, 725–757, https://doi.org/10.1017/RDC.2020.41, 2020.
Remy, C. C., Fouquemberg, C., Asselin, H., Andrieux, B., Magnan, G., Brossier, B., Grondin, P., Bergeron, Y., Talon, B., Girardin, M. P., Blarquez, O.: Guidelines for the use and interpretation of palaeofire reconstructions based on various archives and proxies, Quaternary. Sci. Rev., 193, 312–322, https://doi.org/10.1016/j.quascirev.2018.06.010, 2018.
Rogers, B. M., Soja, A. J., Goulden, M. L., and Randerson, J. T.: Influence of tree species on continental differences in boreal fires and climate feedbacks, Nat. Geosci., 8, 228–234, https://doi.org/10.1038/ngeo2352, 2015.
Rowe, J. S.: Concepts of fire effects on plant individuals and species, in: The role of fire in northern circumpolar ecosystems, 18th edn., edited by: Wein, R. W. and McLean, D. A., Wiley, Chichester, 1983.
Rudaya, N., Krivonogov, S., Słowiński, M., Cao, S., and Zhilich, S: Postglacial history of the Steppe Altai: Climate, fire and plant diversity, Quaternary Sci. Rev., 249, 106616, https://doi.org/10.1016/j.quascirev.2020.106616, 2020.
Sannikov, S. N. and Goldammer, J. G.: Fire ecology of pine forests of northern Eurasia, in: Fire in ecosystems of boreal Eurasia, Forestry Sciences, edited by: Goldammer, J. G. and Furyaev, V. V., Kluver, Dordrecht, 48, 151–167, https://doi.org/10.1007/978-94-015-8737-2_1, 1996.
Scheffer M., Hirota M., Holmgren M., Van Nes, E. H., and Chapin III, F. S.: Thresholds for Boreal Biome Transitions, P. Natl. Acad. Sci. USA, 109, 21384–21389, https://doi.org/10.1073/pnas.1219844110, 2012.
Scholten, R. C., Jandt, R., Miller, E. A., Rogers, B. M., and Veraverbeke, S.: Overwintering fires in boreal forests, Nature, 593, 399–404, https://doi.org/10.1038/s41586-021-03437-y, 2021.
Stivrins, N., Aakala, T., Ilvonen, L., Pasanen, L., Kuuluvainen, T., Vasander, H., Gałka, M., Disbrey, H. R., Liepins, J., Holmström, L., and Seppä, H.: Integrating fire-scar, charcoal and fungal spore data to study fire events in the boreal forest of northern Europe, The Holocene, 29, 1480–1490, https://doi.org/10.1177/0959683619854524, 2019.
Swindles, G., Morris, P., Mullan, D., Watson, E. J., Turner, T. E., Roland, T. P., Amesbury, M. J., Kokfelt, U., Schoning, K., Pratte, S., and Gallego-Sala, A.: The long-term fate of permafrost peatlands under rapid climate warming, Scientific Reports, 5, 17951, https://doi.org/10.1038/srep17951, 2016.
Swindles, G. T., Morris, P. J., Mullan, D. J., Payne, R. J., Roland, T. M., Amesbury, M. J., Lamentowicz, M., Turner, T. E., Gallego-Sala, A., Sim, T., Barr, L. D., Blaauw, B., Blundell, B., Chambers, F. M., Charman, D. J., Feurdean, A., Galloway, J. M., Gałka, M., Green, S., Kajukało, K., Karofeld, E., Korhola, A., Lamentowicz, Ł., Langdon, P., Marcisz, K., Mauquoy, D., Mazei, Y. A., McKeown, M., Mitchell, E. A. D., Novenko, E., Plunkett, G., Roe, H. M., Schoning, K., Sillasoo, Ü., Tsyganov, A., van der Linden, M., Väliranta, M., and Warner, B.: Widespread drying of European peatlands in recent centuries, Nat. Geosci., 12, 922–928, https://doi.org/10.1038/s41561-019-0462-z, 2019.
Tautenhahn, S., Lichstein, J. W., Jung, M., Kattge, J., Bohlman, S. A., Heilmeier, H., Prokushkin, A., Kahl, A., and Wirth, C.: Dispersal limitation drives successional pathways in Central Siberian forests under current and intensified fire regimes, Global Change Biol., 22, 2178–2197, https://doi.org/10.1111/gcb.13181, 2016.
Turunen, J., Tahvanainen, T., Tolonen, K., and Pitkänen, A.: Carbon accumulation in West Siberian Mires, Russia Sphagnum peatland distribution in North America and Eurasia during the past 21,000 years, Global Biogeochem. Cy., 15, 285–296, https://doi.org/10.1029/2000GB001312, 2001.
Vachula, R. S., Sae-Lim, J., and Li, R.: A critical appraisal of charcoal morphometry as a paleofire fuel type proxy, Quaternary Sci. Rev., 262, 106979, https://doi.org/10.1016/j.quascirev.2021.106979, 2021.
van Marle, M. J. E., Kloster, S., Magi, B. I., Marlon, J. R., Daniau, A.-L., Field, R. D., Arneth, A., Forrest, M., Hantson, S., Kehrwald, N. M., Knorr, W., Lasslop, G., Li, F., Mangeon, S., Yue, C., Kaiser, J. W., and van der Werf, G. R.: Historic global biomass burning emissions for CMIP6 (BB4CMIP) based on merging satellite observations with proxies and fire models (1750–2015), Geosci. Model Dev., 10, 3329–3357, https://doi.org/10.5194/gmd-10-3329-2017, 2017.
Vompersky, S. E., Ivanova, A. I., Tsyganova, O. P., Valiaeva, N. A., Glukhova, T. V., Dubinin, F. I., and Markelova, L. G.: Wet soilsand mires in Russia and their carbon pool, Pochvovedenie, 12, 17–25, 1994 (in Russian).
Walker, X. J., Baltzer, J. L., Cumming, S. G., Day, N. J., Ebert, C., Goetz, S., Johnstone, J. F., Potter, S., Rogers, B. M., Schuur, E. A. G., Turetsky, M. R., and Mack, M. C.: Increasing wildfires threaten historic carbon sink of boreal forest soils, Nature, 572, 520–523, https://doi.org/10.1038/s41586-019-1474-y, 2019.
Wirth, C.: Fire regime and tree diversity in boreal forests: implications for the carbon cycle, in: Forest Diversity and Function, edited by: Scherer-Lorenzen, M., Körner, C., and Schulze, E. D., Springer, Berlin, Heidelberg, 309–344, https://doi.org/10.1007/3-540-26599-6_15, 2005.
Whitlock, C. and Larsen, C.: Charcoal as a fire proxy, in: Tracking environmental change using lake sediments. Volume 3: Terrestrial, algal, and siliceous indicators, edited by: Smol, J. P., Birks, H. J. B., and Last, W. M., Kluwer Academic Publishers, 75–97, https://doi.org/10.1007/0-306-47668-1_5, 2001.
Whitlock, C., Colombaroli, D., Conedera, M., and Tinner, W.: Land-use history as a guide for forest conservation and management, Conserv. Biol., 32, 84–97, https://doi.org/10.1111/cobi.12960, 2018.
Whitman, E., Parisien, M. A., Thompson, D. K., and Flannigan, M. D.: Topoedaphic and forest controls on post-fire vegetation assemblies are modified by fire history and burn severity in the northwestern Canadian boreal forest, Forests, 9, 151, https://doi.org/10.3390/f9030151, 2018.
Whitman, E., Parisien, M. A., Thompson, D. K., and Flannigan, M. D.: Short-interval wildfire and drought overwhelm boreal forest resilience, Scientific Reports, 9, 18796, https://doi.org/10.1038/s41598-019-55036-7, 2019.
Zhang, D. and Feng, Z.: Holocene climate variations in the Altai Mountains and the surrounding areas: A synthesis of pollen records, Earth-Sci. Rev., 185, 847–869, https://doi.org/10.1016/j.earscirev.2018.08.007, 2018.
Zolnikov, I. D., Nikulina, A. V., Pavlenok, K. K., Vybornov, A. V., Postnov, A. V., Bychkov, D. A., and Glushkova, N. V.: Regularities in the spatial location of archaeological objects in Timks, Rossiiskaia arkheologiia, 1, 22–31, https://doi.org/10.31857/S086960630008251-5, 2020.