the Creative Commons Attribution 4.0 License.
the Creative Commons Attribution 4.0 License.
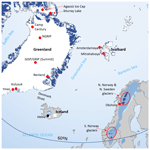
Is there evidence for a 4.2 ka BP event in the northern North Atlantic region?
Raymond S. Bradley
Jostein Bakke
We review paleoceanographic and paleoclimatic records from the northern North Atlantic to assess the nature of climatic conditions at 4.2 ka BP, which has been identified as a time of exceptional climatic anomalies in many parts of the world. The northern North Atlantic region experienced relatively warm conditions from 6 to 8 ka BP, followed by a general decline in temperatures after ∼5 ka BP, which led to the onset of neoglaciation. Over the last 5000 years, a series of multi-decadal- to century-scale fluctuations occurred, superimposed on an overall decline in temperature. Although a few records do show a glacial advance around 4.2 ka BP, because they are not widespread we interpret them as local events – simply one glacial advance of many that occurred in response to the overall climatic deterioration that characterized the late Holocene.
- Article
(5804 KB) - Full-text XML
- BibTeX
- EndNote
The North Atlantic is a key area in the global climate system because changes in atmospheric and oceanographic conditions in this region can have widespread effects on global climate. It is the core region for ventilation of the North Atlantic that drives the Atlantic Meridional Overturning Circulation (AMOC), with global teleconnections through the conveyor belt system of ocean currents. Detailed studies of two sediment cores in the North Atlantic (at ∼65 and N) by Bond et al. (1997) revealed quasiperiodic variations in the percentage of hematite-stained grains and Icelandic glass during the Holocene, which were interpreted as evidence for pulses of ice rafting. They argued that during these episodes, “cool, ice-bearing surface waters shifted across more than 5∘ of latitude, each time penetrating well into the core of the North Atlantic Current”. One of the eight Holocene episodes (later dubbed “Bond events”) occurred at ∼4.2 ka calendar years BP. Given that this is the time at which exceptional climatic anomalies appear to have occurred in many parts of the world (“the 4.2 ka BP event”; see Weiss, 2017) it is important to reassess the evidence for the disruption of the North Atlantic Current at that time.
Bond et al. (2001) argued that the colder episodes they had identified were driven by a reduction in solar insolation (Wanner and Bütikofer, 2008; Wanner et al., 2011), notwithstanding the fact that total solar irradiance did not vary by more than ±0.15 % over this period (Vieira et al., 2011; Roth and Joos, 2013; Wu et al., 2018). Nevertheless, the literature is replete with studies that have tried to link diverse paleoclimatic records from around the world to the timing of Bond events (e.g., Fleitmann et al., 2003; Gupta et al., 2003; Wang et al., 2005; Pèlachs et al., 2011), despite the fact that other paleoceanographic studies have been unable to reproduce the record of ice rafting reported in Bond et al. (1997) (e.g., Andrews et al., 2014). Here we review sedimentary records from the northern North Atlantic (north of 60∘ N) with a specific focus on whether there is evidence for an “event” around 4.2 ka BP. We do not focus on records from Iceland as these have been reviewed separately by Geirsdóttir et al. (2019).
The North Atlantic has a very distinct pattern of sea surface temperatures, reflecting the ocean currents that traverse the region (Fig. 1). Warm subtropical water enters the region from the southwest via the Gulf Stream (North Atlantic Current) and this transfers heat to subpolar latitudes north of Scandinavia by way of the Norwegian Atlantic and West Spitsbergen currents, as well as around the western and northwestern coast of Iceland via the Irminger Current. In contrast, cold polar water exits the Arctic Ocean via the East Greenland Current, which extends to the southern tip of Greenland. The region between these water masses is where deepwater formation occurs, driving the large-scale Atlantic Meridional Overturning Circulation (AMOC). On the timescale of the Holocene, there have been significant changes in the characteristics and position of these major oceanographic features, as recorded by various paleoceanographic proxies.
First, we consider a transect of sediment cores that are aligned along the axis of the main influx of Atlantic water entering the North Atlantic, from west of the UK to Svalbard (Fig. 1). We focus on studies that have provided estimates of paleo sea surface temperatures. Effectively, this means only those that have analyzed alkenones and diatoms, which reflect conditions in the photic zone or mixed layer near the ocean surface. Figure 1 shows the location of all available Holocene alkenone-based paleotemperature estimates (Fig. 2; see references in the caption). These indicate that sea surface temperatures (SSTs) were higher in the early Holocene, with the largest anomalies (relative to today) at high latitudes (that is, there was strong polar amplification of the warming) (Andersson et al., 2010). This early Holocene warming was a consequence of orbital forcing: June–July insolation was ∼10 % higher than today at the start of the Holocene in the northern parts of the region, but the peak warming was delayed due to the influence of the decaying Laurentide and Scandinavian ice sheets and associated icebergs and freshwater (Renssen et al., 2009, 2012; Zhang et al., 2016). Consequently, maximum temperatures were a few thousand years later than the peak insolation, punctuated by a short-lived cooling event around 8.2 ka BP associated with the final major freshwater discharge event of the Laurentide Ice Sheet (Barber et al., 1999; Rohling and Pälike, 2005). Thereafter, as insolation declined so sea surface temperatures declined steadily or, by some estimates, in a more step-like manner (e.g., Calvo et al., 2002; Risebrobakken et al., 2010). For example, Birks and Koç (2002), Andersen et al. (2004), and Berner et al. (2011) all found that August SSTs at 67∘ N (core MD95-2011) were 4–5 ∘C warmer than today from ∼9000–6500 years BP, then steadily declined. These analyses were based on diatoms, but similar results (albeit with a smaller change in temperature, ∼2.5 ∘C, perhaps reflecting a different seasonal bias) were obtained in a study of alkenones from the same core (Calvo et al., 2002). Studies further north paint a similar picture (Sarnthein et al., 2003; Risebrobakken et al., 2003, 2010; Werner et al., 2014). This pattern of maximum SSTs in the first half of the Holocene and cooling thereafter is seen throughout the eastern North Atlantic in all proxies that are indicative of conditions in the photic zone (Rimbu et al., 2003; Leduc et al., 2010; Sejrup et al., 2016). The timing of the onset of cooling varies, but cooling was well underway by ∼5.5 ka BP in what some refer to as a “transition period” that subsequently led to much cooler conditions in the late Holocene (after 3.5 ka BP) (e.g., Aagaard-Sorensen et al., 2014; Andersen et al., 2004; Leduc et al., 2010; Sejrup et al., 2016). Although there were short-lived cooling episodes superimposed on the overall first-order pattern of temperature change (e.g., Werner et al., 2014), there is no evidence for quasiperiodic cooling episodes disrupting the northward flux of Atlantic water, as described by Bond et al. (1997). Proxies of subsurface conditions (below the mixed layer) – Mg∕Ca ratios and oxygen isotopes in forams, as well as foram assemblage changes – generally do not show the same pattern of pan-Holocene cooling as the SST proxies, often indicating slight warming through the Holocene (e.g., Andersson et al., 2010; Sejrup et al., 2011). But these records also do not show a pattern of quasiperiodic cooling events. Could this be because of low resolution in sampling or poor chronologies? This seems very unlikely as many of these records are from high-deposition-rate sites, providing high-resolution records that are generally well dated (e.g., Berner et al., 2011). Indeed, one exceptionally well-dated, high-resolution sediment core from the Storegga Slide region (90 AMS 14C dates over 8000 calendar years) provides oxygen isotope data on planktonic forams at a resolution of ±20 years within the core of the Norwegian Atlantic Current at ∼64∘ N (Sjejrup et al., 2011). This clearly shows multi-decadal- to century-scale variability throughout the last 8000 years, but none of the cold-water flux episodes that one would expect to see based on the work of Bond et al. (1997). We therefore conclude that there is no signal of a 4.2 ka BP event in paleoceanographic proxies from regions influenced by the flux of warm water from the subtropical Atlantic into the Nordic Seas. Cooling of the sea surface had set in more than a millennium earlier in this region.
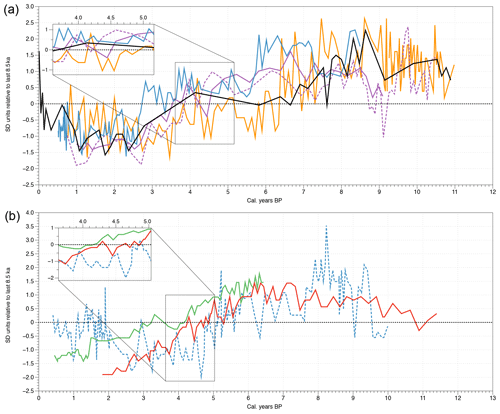
Figure 2Alkenone-based paleo SST estimates from North Atlantic sites (shown in Fig. 1). (1) Black – Risebrobakken et al. (2010): core PSh-5159N; 71.35∘ N, 22.63∘ E. (2) Purple – Marchal et al. (2002): core M23258-2; 75∘ N, 13.97∘ E. (3) Dashed purple – Rigual-Hernández et al. (2017): core SV-04; 74.957∘ N, 13.899∘ E. (4) Orange – Marchal et al. (2002): core MD95-2015; 58.76∘ N, 25.958∘ W. (5) Blue – Calvo et al. (2002): core MD95-2011; 66.97∘ N, 7.633∘ E. (6) Red – Emeis et al. (2003): core IOW 22517; 57.67∘ N, 7.091∘ E. (7) Green – Emeis et al. (2003): core IOW 22514; 57.84∘ N, 8.704∘ E. (8) Dashed blue – Kristiánsdóttir et al. (2017): core MD2269; 66.63∘ N, 20.85∘ W.
Next, we consider studies in the western part of the North Atlantic, north of Iceland on the Icelandic Shelf, and further to the west near Denmark Strait. Here, many studies have examined, inter alia, foraminiferal assemblages, coccoliths, dinoflagellate cysts, and sea ice biomarkers and ice-rafted debris (IRD) reflecting the transport of material in the cold East Greenland Current (e.g., Andrews et al., 1997; Jennings et al., 2002; Giraudeau et al., 2004; Solignac et al., 2006; Sicre et al., 2008; Justwan et al., 2008; Perner et al., 2015; Moossen et al., 2015; Cabedo-Sanz et al., 2016; Kolling et al., 2017). In this region, the warmest conditions occurred around 6.0±1.5 ka BP (the timing depending on location); these conditions were associated with minimal input of IRD, reflecting the recession of tidewater glaciers onto land along the eastern coast of Greenland, and a weak East Greenland Current, with minimal stratification of the water column at that time as the flux of warmer, more saline Irminger Current water increased (Justwan et al., 2008; Jennings et al., 2011; Werner et al., 2014; Telesiński et al., 2014; Perner et al., 2016). Conditions began to change by ka BP (the timing varying geographically) when cold-water diatoms and forams, sea ice (as tracked by the biomarker index IP25), and IRD started to increase, and the water column became more stratified as the East Greenland Current strengthened (Moros et al., 2006; Telesinski et al., 2014; Perner et al., 2016; Kristiánsdóttir et al., 2017). These changes correspond to the readvance of glaciers in East Greenland, part of the much more widespread onset of neoglaciation that is well documented in many regions around the North Atlantic (Solomina et al., 2015). Warmer conditions (related to a strengthened Irminger Current) developed over the past 2000 years, but this period is also characterized by a series of minor fluctuations in the extent of ice in the region, with much colder conditions after ∼1.0 ka BP when the coldest conditions of the last 8000 years occurred, with abundant IRD and sea ice in Denmark Strait and off the north coast of Iceland (Bendle and Rosell-Mele, 2007; Andresen et al., 2013; Cabedo-Sanz at al 2016; Kolling et al., 2017). None of these records show evidence of an unusual anomaly at 4.2 ka BP; rather, the overall cooling of the late Holocene began 500–1000 years earlier (Orme et al., 2018). Similar variability is also seen further south and southwest of Iceland at ∼59∘ N (Farmer et al., 2008; Moros et al., 2012; Orme et al., 2018), though there is evidence from dinocysts for an anomaly in the seasonality of SSTs at ∼4.5 ka BP, perhaps related to a westward shift in the Subpolar Gyre, allowing warmer Atlantic water to influence the site (van Nieuwenhove et al., 2018).
This review of paleoceanographic studies extending from southern Greenland to Fram Strait, and from western Svalbard and the southern Barents Sea southward to 60∘ N, provides no evidence for a significant change in major oceanographic conditions that could be linked to the 4.2 ka BP climate anomaly seen elsewhere. Rather, the evidence points to a more gradual change that was well under way by ∼5 ka BP from the relatively warm conditions of the early Holocene (driven by precessional forcing) to much colder conditions that have characterized the last 3 millennia.
3.1 Eastern Greenland and the Greenland Ice Sheet
Lake sediment records from sites along the coast of eastern Greenland provide a record of Holocene environmental conditions that generally reinforces the paleoceanographic evidence discussed earlier. A “Holocene thermal maximum” (characterized inter alia by longer ice-free conditions, higher levels of lacustrine productivity, increased evaporation, more tundra vegetation and higher levels of terrestrial plant material transferred to lakes) is clearly seen from ∼8 ka BP (or earlier) to ka BP (e.g., Kaplan et al., 2002; Andresen et al., 2004; Schmidt et al., 2011; Balascio et al., 2013; Wagner and Bennike, 2015; Axford et al., 2017; van der Bilt et al., 2018a). Thereafter, conditions became colder, often with a decline in vegetation cover, an increase in the flux of coarse-grained sediments, and a shift in the types of chironomids and diatoms present towards species that thrive in cooler conditions. At the same time, in glacierized watersheds, the growth of glaciers led to an increase in the flux of minerogenic material, which is a diagnostic signal of the onset of late Holocene neoglaciation across the region. In Kulusuk Lake (65∘ N on the coast of southeastern Greenland) this change occurred at ∼4.2 ka BP, when there was an abrupt increase in clastic sediments from glaciers that had probably disappeared during the mid-Holocene warm period (Balascio et al., 2015). A similar transition is seen in sediments from nearby Ymer Lake, where a higher frequency of avalanches and a longer season with ice cover is thought to have favored the transfer of coarser material into the lake after ∼4 ka BP (van der Bilt et al., 2018a). At another site in the same region, the Holocene thermal maximum was identified (via the evaporative enrichment of δD in leaf wax n-alkanes) from 8.4 to 4.1 ka B.P, followed by a decrease in evaporation as the open-water season became shorter. At the same time, there was an increase in the flux of clastic sediments and terrestrial organic material into the lake as river runoff increased (Balascio et al., 2013). In all of these studies, it is clear that there was a fairly rapid transition from warm mid-Holocene conditions to the colder, wetter late Holocene that encompassed the 4.2 ka BP interval of interest. In some cases, there is evidence for a short-lived event at around that time (e.g., at Kulusuk Lake; Balascio et al., 2015), but this appears to be simply a part of the overall deterioration in climate that led to ice growth across the region. There is currently no evidence for a more widespread glacial advance at 4.2 ka BP. Given that cooling was persistent over the last 5000 years and the elevational threshold for glacierization is close to mountain tops across the region (declining in elevation poleward), it is understandable that different locations would have experienced the onset of neoglaciation at different times (Geirsdóttir et al., 2019). However, as the equilibrium line altitude (ELA) continued to lower over the last 3–4 millennia, glaciers that had greatly diminished in size or disappeared entirely during the warmest period of the Holocene were eventually regenerated, with the exact timing varying across the region. In the case of Kulusuk Lake, it seems reasonable to conclude that the steady decline in temperatures and the specific hypsography of that basin led to a short-lived positive mass balance, with early ice growth and associated sediment input to the lake around 4.2 ka BP. This was the first of several advances within the Neoglacial period.
Ice cores from Greenland provide records of past climate variations from oxygen isotopes, glaciochemistry and physical characteristics, which are broadly consistent with those from coastal lake sediments. Alley and Anandakrishnan (1995) examined evidence for summer melting in the GISP2 ice core, as recorded by changes in the physical properties of the ice. Their analysis was at a relatively low resolution, but they showed maximum Holocene summer temperatures from ∼7.5 ka BP, followed by a two-step transition to colder conditions, from ∼6.5 to 5.5 ka BP and ∼4.5 to 4 ka BP, with persistently low summer temperatures (minimal melting) thereafter. After adjusting for ice thickness changes, Vinther et al. (2009) also showed that there was an overall decline in temperature at Summit on the Greenland Ice Sheet (73∘ N, 3210 m a.s.l.) over the last ∼9000 years (interpreted from changes in δ18O in the GISP2 ice core). The mean temperature of the warmest and coldest millennia (7–8 ka and 0–1 ka b2k, respectively) differ by ∼2.35 ∘C (assuming no change in the seasonality of snowfall on the ice sheet). Superimposed on the long-term temperature decline there were multi-decadal anomalies on the order of ±1 ∘C. One of the largest of the negative anomalies after the well-known 8.2 ka BP event began ∼4400 b2k and reached a minimum at 4340 b2k, but by 4200 b2k, temperatures had sharply increased (Fig. 3). In the Vinther et al. (2009) reconstruction (Fig. 3a, which combines data from Renland and Agassiz ice caps), this appears to be driven mainly by the record from Agassiz Ice Cap on Ellesmere Island (Fig. 3b); nothing comparable is seen in oxygen isotopic records from Summit or Renland (Fig. 3b, c), or in the Summit temperature reconstruction of Kobashi et al. (2017), based on the differential diffusion of argon and nitrogen isotopes in firn prior to its densification into ice (Fig. 3d). However, δ18O in chironomid head capsules from a lake in northwest Greenland also recorded the highest values of the last ∼6000 years at ∼4.2 ka BP (Lasher et al., 2017), and at Camp Century there was a local isotopic maximum shortly before 4000 BP (Fig. 3b). In Murray Lake (northeastern Ellesmere Island), relatively warm conditions at ∼4.2 ka BP were reconstructed from varve thickness (Cook et al., 2009). Similarly, Gkinis et al. (2014) found an abrupt increase in temperature in the NorthGRIP ice core at ∼4200 b2k after deconvolving the isotopic record to take into account diffusion effects that have smoothed the signal. However, this technique is very sensitive to the assumptions made about the past accumulation rate, as diffusion is a function of both past accumulation and temperature. For example, a 15 % reduction in accumulation would reduce an apparent temperature anomaly from 5 to 3.5 ∘C (V. Gkinis, personal communication, 2019). Under the assumption of no changes in accumulation rate, Gkinis et al. (2014) identify a warm period in the NorthGRIP core at 4.2 ka BP and refer to this as the “mid-Holocene optimum”. It will be interesting to see if this technique, when applied to other ice cores, reveals more details about short-term temperature fluctuations that may have been obscured by diffusion effects. But for now, only three records, from northwest Greenland and northern Ellesmere Island, point to short-lived warmer conditions at ∼4.2 ka BP in contrast to the majority of records that indicate temperatures were declining at that time.
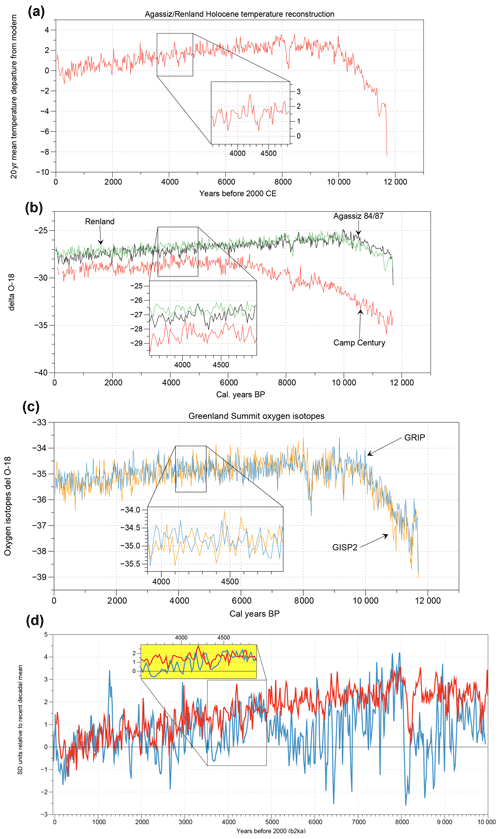
Figure 3(a) Temperature anomalies from the smoothed estimate of present temperatures in Greenland based on oxygen isotope records from Renland Ice Cap (East Greenland) and Agassiz Ice Cap (Ellesmere Island). Timescale is in years b2k (before 2000 CE). The interval around 4.2 ka BP is enlarged in the box (data source: Vinther et al., 2009). For locations, see Fig. 5. (b) Individual oxygen isotopic records from Renland and Agassiz ice caps (which were combined to create the record in Fig. 3a) and from Camp Century. (c) Individual oxygen isotopic records from GRIP and GISP2 at Summit, Greenland Ice Sheet. (d) Paleotemperature estimates from argon and nitrogen isotopes (in blue) (from Kobashi et al., 2017) and from the Renland–Agassiz joint record (in red) as shown in Fig. 3a (from Vinther et al., 2009).
3.2 Iceland
Iceland is in a central location to experience changes in the major oceanic and atmospheric circulation patterns of the North Atlantic. We did not undertake a review of the literature on the Holocene paleoclimatology of Iceland as that is well summarized by Geirsdóttir et al. (2019). They conclude that neoglaciation in Iceland had begun by 5 ka BP but different topographic features and proximity to the ocean led to varying environmental effects across the island. Several step-like changes occurred during the last 5 kyr, culminating in the most extensive glacier advances during the last millennium. One of the step-like changes occurred at ∼4.5–4.0 ka BP, and they conclude that this is indistinguishable from a 4.2 ka BP event. They note that the eruption of Hekla at 4.2 ka deposited at ≥ 1 cm of tephra over 80 % of Iceland, so the direct effects on the landscape at that time complicate the detection of a signal that may be related to other forcing factors. Of the two lakes in NE Iceland that did not have a tephra in the sediments, one (Skoravatn) shows an abrupt change at 4.2 ka BP, while the other (Tröllkonuvatn) does not, making it difficult to draw conclusions about the impact of the eruption on changes recorded at that time.
3.3 Svalbard
Lake sediment records from Svalbard record changes in climate at the northernmost limit of North Atlantic water (the West Spitsbergen Current). All studies describe a warm early Holocene phase when many of the glaciers seen today were small or absent (Farnsworth, 2018). On Amsterdamoya, at the northwestern edge of Svalbard, warm and dry conditions spanned the interval from 7.7 to 5 ka BP; glaciers were small or absent by 8.4 ka BP, only reforming in the late Holocene (Gjerde et al., 2018; de Wet et al., 2018). To the south, on the Mitrahalvoya Peninsula, there is also evidence that glaciers reached their minimum size by the mid-Holocene but subsequently reformed or readvanced. Karlbreen began to grow around 3.5 ka BP (Røthe et al., 2015), but in the neighboring watershed of Hajeren an abrupt increase in minerogenic sediments at 4.25 ka BP registered the onset of neoglaciation in that basin (van der Bilt et al., 2015). Paleotemperature estimates (from alkenones) in the same record indicate this advance was triggered by an abrupt drop in temperature at that time; thereafter, temperatures remained low (van der Bilt et al., 2018b). Other records from the region indicate that the first neoglacial advances of glaciers occurred around 4.6 ka BP (e.g., Svendsen and Mangerud, 1997; Reusche et al., 2014).
3.4 Scandinavia
As most glaciers in Scandinavia had their largest areal extent during the “Little Ice Age” (∼ 1400–1850 CE), information about past glaciers in Norway during the late Holocene is based on reconstructions from indirect evidence, mainly sediments deposited in distal glacier-fed lakes (e.g., Nesje, 2009; Bakke et al., 2010, 2013). After several large glacier advances in the earliest Holocene, the climate was generally warm during the early Holocene (8.5–6.5 ka BP) and most glaciers melted away completely (Nesje, 2009) (Fig. 4). Around 6 ka BP glaciers start to regrow mainly as a function of decreasing summer insolation over the Northern Hemisphere (Wanner et al., 2008). The regrowth of glaciers follows a pattern of gradual increases in glacier size interrupted by smaller glacier advances (Bakke et al., 2010, 2013; Vasskog et al., 2012). Along a coastal south–north transect through Scandinavia, different locations experienced the onset of neoglaciation at different times, mainly as a function of altitude (Geirsdóttir et al., 2018). By 2 ka BP many glaciers had reached present-day size, but maximum glacier extent was in the 18th century during the Little Ice Age (Nesje, 2009). A review of more than 20 papers shows that none of them indicate any abrupt anomalous change in glacier extent connected to a perturbation of climate around 4.2 ka (Bakke et al., 2005a, b, 2008, 2010, 2013; Dahl and Nesje, 1992, 1994, 1996; Lauritzen, 1996; Snowball and Sandgren, 1996; Seierstad et al., 2002; Lie et al., 2004; Nesje et al., 2009; Vasskog et al., 2011, 2012; Støren et al., 2008; Wittmeier et al., 2015; Shakesby et al., 2007; Kvisvik et al., 2015; Gjerde et al., 2016). Investigating this further, we examined other terrestrial evidence, mainly pollen, macrofossil and diatom records derived from lake sediments (e.g., Bjune et al., 2005; Velle et al., 2005). They have a time resolution somewhat lower than the glacier reconstructions (typical 500-year spacing) but they all reflect the general decrease in summer insolation over the Northern Hemisphere and no abrupt transition close to 4.2 ka BP (Bjune, 2005; Bjune et al., 2004; Velle et al., 2005). The only terrestrial evidence from Scandinavia that shows a clear anomaly close to 4.2 ka BP is a speleothem record of δ18O from northern Norway, which records a short-lived temperature maximum (isotopic minimum) at ∼4 ka, before rapidly decreasing to much colder temperatures at ∼3.7 ka BP (Lauritzen and Lundberg, 1999). However, a speleothem from a nearby cave (Okshola) does not show a comparable anomaly at this time (Linge et al., 2009).
A review of paleoceanographic and terrestrial paleoclimatic data from around the northern North Atlantic reveals no compelling evidence for a significant and widespread climatic anomaly at ∼4.2 ka BP (i.e., an event) in most areas. In particular, there is no supporting evidence for “cool, ice-bearing surface waters… penetrating well into the core of the North Atlantic Current” at that time, as described by Bond et al. (2001). The region experienced relatively warm conditions from 6 to 8 ka BP, followed by a general decline in temperatures after ∼5 ka BP, signalling the onset of neoglaciation. Over the last 5000 years, a series of multi-decadal- to century-scale fluctuations occurred, superimposed on an overall decline in temperature. Against this background of declining temperatures, three records in northwest Greenland and Ellesmere Island show an unusual warm anomaly around 4.2 ka BP, and a few others (in SE Greenland, Iceland and western Svalbard) show a cold anomaly associated with a glacial advance. We interpret these as local events – simply one glacial advance of many that occurred in response to the overall climatic deterioration that characterized the late Holocene. Given that the northern North Atlantic is a key region for the formation of deepwater, which has consequences for the overall global oceanic circulation (the “conveyor belt”), the absence of a strong signal of an abrupt climatic event at 4.2 ka BP suggests that – whatever the cause of changes seen elsewhere – it is unlikely that the North Atlantic Ocean circulation played a driving role. If this conclusion is correct, it requires the cause of the 4.2 ka BP event to be sought elsewhere in terms of direct radiative forcing (possibly due to explosive volcanic events or earth surface aerosols resulting from aridity or – less likely – solar forcing). Currently, none of these possibilities provide a compelling argument. The alternative is that the observed changes were a consequence of internal climate system variability, perhaps modulated by the overall decline in summer radiation across the Northern Hemisphere due to orbital changes, which are generally considered the cause of neoglaciation in the late Holocene, the onset of which roughly corresponds to the 4.2 ka event as described by Weiss (2017).
Data for figures were obtained from the NOAA and PANGAEA paleoclimatology databases (https://www.ncdc.noaa.gov/data-access/paleoclimatology-data/datasets and https://www.pangaea.de, respectively).
RB designed the study; RB and JB undertook reviews of the literature and cowrote the paper.
The authors declare that they have no conflict of interest.
This article is part of the special issue “The 4.2 ka BP climatic event”. It is a result of “The 4.2 ka BP Event: An International Workshop”, Pisa, Italy, 10–12 January 2018.
This research has been supported by the NSF (grant no. PLR 1417667).
This paper was edited by Giovanni Zanchetta and reviewed by Harvey Weiss and one anonymous referee.
Aagaard-Sørensen, S., Husum, K., Hald, M., Marchitto, T., and Godtliebsen, F.: Sub-sea surface temperatures in the Polar North Atlantic during the Holocene: Planktic foraminiferal Mg∕Ca temperature reconstructions, Holocene, 24, 93–103, 2014.
Alley, R. B. and Anandakrishnan, S.: Variations in melt-layer frequency in the GISP2 ice core: implications for Holocene summer temperatures in central Greenland, Ann. Glaciol., 21, 64–70, 1995.
Andersen, C., Koc, N., Jennings, A., and Andrews, J. T.: Nonuniform response of the major surface currents in the Nordic Seas to insolation forcing: implications for the Holocene climate variability, Paleoceanogr. Paleocl., 19, PA2003, https://doi.org/10.1029/2002P, 2004.
Andersson, C., Pausata, F. S. R., Jansen, E., Risebrobakken, B., and Telford, R. J.: Holocene trends in the foraminifer record from the Norwegian Sea and the North Atlantic Ocean, Clim. Past, 6, 179–193, https://doi.org/10.5194/cp-6-179-2010, 2010.
Andresen, C. S., Björck, S., Bennike, O., and Bond, G.: Holocene climate changes in southern Greenland: evidence from lake sediments, J. Quaternary Sci., 19, 783–795, 2004.
Andresen, C. S., Hansen, M. J., Seidenkrantz, M. S., Jennings, A. E., Knudsen, M. F., Nørgaard-Pedersen, N., Larsen, N. K., Kuijpers, A., and Pearce, C.: Mid-to late-Holocene oceanographic variability on the Southeast Greenland shelf, Holocene, 23, 167–178, 2013.
Andrews, J. T., Smith, L. M., Preston, R., Cooper, T., and Jennings, A. E.: Spatial and temporal patterns of iceberg rafting (IRD) along the East Greenland margin, ca. 68∘ N, over the last 14 cal. Ka, J. Quaternary Sci. 12, 1–13, 1997.
Andrews, J. T., Bigg, G. R., and Wilton, D. J.: Holocene ice-rafting and sediment transport from the glaciated margin of East Greenland (67–70∘ N) to the N. Iceland shelves: detecting and modeling changing sediment sources, Quaternary Sci. Rev., 91, 204–217, 2014.
Axford, Y., Levy, L. B., Kelly, M. A., Francis, D. R., Hall, B. L., Langdon, P. G., and Lowell, T. V.: Timing and magnitude of early to middle Holocene warming in East Greenland inferred from chironomids, Boreas, 46, 678–687, 2017.
Bakke, J., Nesje, A., and Dahl, S. O.: Utilizing physical sediment variability in glacier-fed lakes for continuous glacier reconstructions during the Holocene, northern Folgefonna, western Norway, Holocene, 15, 161–176, 2005a.
Bakke, J., Dahl, S. O., Paasche, Ø., Løvlie, R., and Nesje, A.: Glacier fluctuations, equilibrium-line altitudes and palaeoclimate in Lyngen, northern Norway, during the Lateglacial and Holocene, Holocene, 15, 518–540, 2005b.
Bakke, J., Lie, Ø., Dahl, S. O., Nesje, A., and Bjune, A. E.: Strength and spatial patterns of the Holocene wintertime westerlies in the NE Atlantic region, Glob. Planet. Change, 60, 28–41, 2008.
Bakke, J., Dahl, S. O., Paasche, Ø., Simonsen, J. R., Kvisvik, B., Bakke, K., and Nesje, A.: A complete record of Holocene glacier variability at Austre Okstindbreen, northern Norway: an integrated approach, Quaternary Sci. Rev., 29, 1246–1262, 2010.
Bakke, J., Trachsel, M., Kvisvik, B. C., Nesje, A., and Lyså, A.: Numerical analyses of a multi-proxy data set from a distal glaxcier-fed lake, Sørsendalensvatn, western Norway, Quaternary Sci. Rev., 73, 182–195, 2013.
Balascio, N. L., D'Andrea, W. J., Bradley, R. S., and Perren, B. B.: Biogeochemical evidence for hydrologic changes during the Holocene in a lake sediment record from Southeast Greenland, Holocene, 23, 1428–1439, 2013.
Balascio, N. L., D'Andrea, W. J., and Bradley, R. S.: Glacier response to North Atlantic climate variability during the Holocene, Clim. Past, 11, 1587–1598, https://doi.org/10.5194/cp-11-1587-2015, 2015.
Barber, D. C., Dyke, A., Hillaire-Marcel, C., Jennings, A. E., Andrews, J. T., Kerwin, M. W., Bilodeau, G., McNeely, R., Southon, J., Morehead, M. D., and Gagnon, J. M: Forcing of the cold event of 8,200 years ago by catastrophic drainage of Laurentide lakes, Nature, 400, 344–348, 1999.
Bendle, J. A. and Rosell-Melé, A.: High-resolution alkenone sea surface temperature variability on the North Icelandic Shelf: implications for Nordic Seas palaeoclimatic development during the Holocene, Holocene, 17, 9–24, 2007.
Berner, K. S., Koç, N., Godtliebsen, F., and Divine, D.: Holocene climate variability of the Norwegian Atlantic Current during high and low solar insolation forcing, Paleoceanogr. Paleocl., 6, PA2220, https://doi.org/10.1029/2010PA002002 2011.
Bond, G., Showers, W., Cheseby, M., Lotti, R., Almasi, P., Priore, P., Cullen, H., and Bonani, G.: A pervasive millennial-scale cycle in North Atlantic Holocene and glacial climates, Science, 78, 1257–1266, 1997.
Bond, G., Kromer, B., Beer, J., Muscheler, R., Evans, M. N., Showers, W., Hoffmann, S., Lotti-Bond, R., Hajdas, I., and Bonani, G.: Persistent solar influence on North Atlantic climate during the Holocene, Science, 294, 2130–2136, 2001.
Birks, C. J. and Koc, N.: A high‐resolution diatom record of late‐Quaternary sea‐surface temperatures and oceanographic conditions from the eastern Norwegian Sea, Boreas, 31, 323–344, 2002.
Bjune, A. E.: Holocene vegetation history and tree-line changes on a north–south transect crossing major climate gradients in southern Norway – evidence from pollen and plant macrofossils in lake sediments, Rev. Palaeobot. Palyno., 133, 249–275, 2005.
Bjune, A. E., Birks, H. J. B., and Seppä, H.: Holocene vegetation and climate history on a continental – oceanic transect in northern Fennoscandia based on pollen and plant macrofossils, Boreas, 33, 211–223, 2004.
Bjune, A. E., Bakke, J., Nesje, A., and Birks, H. J. B.: Holocene mean July temperature and winter precipitation in western Norway inferred from palynological and glaciological lake-sediment proxies, Holocene, 15, 177–189, 2005.
Cabedo-Sanz, P., Belt, S. T., Jennings, A. E., Andrews, J. T., and Geirsdóttir, Á.: Variability in drift ice export from the Arctic Ocean to the North Icelandic Shelf over the last 8000 years: a multi-proxy evaluation, Quaternary Sci. Rev., 146, 99–115, 2016.
Calvo, E., Grimalt, J., and Jansen, E.: High resolution U37K sea surface temperature reconstruction in the Norwegian Sea during the Holocene, Quaternary Sci. Rev., 21, 1385–1394, 2002.
Cook, T. L., Bradley, R. S., Stoner, J. S., and Francus, P.: Five thousand years of sediment transfer in a high arctic watershed recorded in annually laminated sediments from Lower Murray Lake, Ellesmere Island, Nunavut, Canada, J. Paleolimnol., 41, 77–94, 2009.
Dahl, S. O. and Nesje, A.: Paleoclimatic implications based on equilibrium line altitude depressions of reconstructed Younger Dryas and Holocene cirque glaciers in inner Nordfjord, western Norway, Palaeogeogr. Palaeocl., 94, 87–97, 1992.
Dahl, S. O. and Nesje, A.: Holocene glacier fluctuations at Hardangerjøkulen, central-southern Norway: a high-resolution composite chronology from lacustrine and terrestrial deposits, Holocene, 4, 269–277, 1994.
Dahl, S. O. and Nesje, A.: A new approach to calculating Holocene winter precipitation by combining glacier equilibrium-line altitudes and pine-tree limits: a case study from Hardangerjøkulen, central southern Norway, Holocene, 6, 381–398, 1996.
de Wet, G., Bakke, J., D'Andrea, W. J., Balascio, N. L., Bradley, R. S., and Perren, B. B.: Holocene climate change reconstructed from proglacial lake Gjoavatnet on Amsterdamoya, N.W. Svalbard, Quaternary Sci. Rev., 183, 188–203, 2018.
Emeis, K. C. and Dawson, A. G.: Holocene palaeoclimate records over Europe and the North Atlantic, The Holocene, 13, 305–309, 2003.
Farmer, E. J., Chapman, M. R., and Andrews, J. E.: Centennial-scale Holocene North Atlantic surface temperatures from Mg∕Ca ratios in Globigerina bulloides, Geochem. Geophy. Geosy., 9, Q12029, https://doi.org/10.1029/2008G, 2008.
Farnsworth, W. R.: Holocene glacier history of Svalbard, Ph.D. thesis, Department of Geosciences, The Arctic University of Norway, Trømso, 188 pp., 2018.
Fleitmann, D., Burns, S. J., Mudelsee, M., Neff, U., Kramers, J., Mangini, A., and Matter, A.: Holocene forcing of the Indian monsoon recorded in a stalagmite from southern Oman, Science, 300, 1737–1739, 2003.
Geirsdóttir, Á., Miller, G. H., Andrews, J. T., Harning, D. J., Anderson, L. S., Florian, C., Larsen, D. J., and Thordarson, T.: The onset of neoglaciation in Iceland and the 4.2 ka event, Clim. Past, 15, 25–40, https://doi.org/10.5194/cp-15-25-2019, 2019.
Giraudeau, J., Jennings, A. E., and Andrews, J. T.: Timing and mechanisms of surface and intermediate water circulation changes in the Nordic Seas over the last 10,000 cal years: a view from the North Iceland shelf, Quaternary Sci. Rev., 23, 2127–2139, 2004.
Gjerde, M., Bakke, J., Vasskog, K, Nesje, A., and Hormes, A.: Holocene glacier variability and Neoglacial hydroclimate at Ålfotbreen, western Norway, Quaternary Sci. Rev., 133, 28–47, 2016.
Gjerde, M., Bakke, J., D'Andrea, W. J., Balascio, N. L., Bradley, R. S., Vasskog, K., Ólafsdóttir, S., Røthe, T. O., Perren, B. B., and Hormes, A.: Holocene multi-proxy environmental reconstruction from Lake Hakluytvatnet, Amsterdamøya Island, Svalbard (79.5∘ N), Quaternary Sci. Rev., 183, 164–176, 2018.
Gkinis, V., Simonsen, S. B., Buchardt, S. L., White, J. W. C., and Vinther, B. M.: Water isotope diffusion rates from the NorthGRIP ice core for the last 16,000 years–glaciological and paleoclimatic implications, Earth Planet. Sc. Lett., 405, 132–141, 2014.
Gupta, A. K., Anderson, D. M., and Overpeck, J. T.: Abrupt changes in the Asian southwest monsoon during the Holocene and their links to the North Atlantic Ocean, Nature, 421, 354–357, 2003.
Jennings, A. E., Knudsen, K. L., Hald, M., Hansen, C. V., and Andrews, J. T.: A mid-Holocene shift in Arctic sea-ice variability on the East Greenland Shelf, Holocene, 12, 49–58, 2002.
Jennings, A., Andrews, J., and Wilson, L.: Holocene environmental evolution of the SE Greenland Shelf North and South of the Denmark Strait: Irminger and East Greenland current interactions, Quaternary Sci. Rev., 30, 980–998, 2011.
Justwan, A., Koç, N., and Jennings, A. E.: Evolution of the Irminger and East Icelandic Current systems through the Holocene, revealed by diatom-based sea surface temperature reconstructions, Quaternary Sci. Rev., 27, 1571–1582, 2008.
Kaplan, M. R., Wolfe, A. P., and Miller, G. H.: Holocene environmental variability in southern Greenland inferred from lake sediments, Quaternary Res., 58, 149–159, 2002.
Kobashi, T., Menviel, L., Jeltsch-Thömmes, A., Vinther, B. M., Box, J. E., Muscheler, R., Nakaegawa, T., Pfister, P. L., Döring, M., Leuenberger, M., and Wanner, H: Volcanic influence on centennial to millennial Holocene Greenland temperature change, Sci. Rep., 7, 1441–1451, 2017.
Kolling, H. M., Stein, R., Fahl, K., Perner, K., and Moros, M.: Short-term variability in late Holocene sea ice cover on the East Greenland Shelf and its driving mechanisms, Palaeogeogr. Palaeocl., 485, 336–350, 2017.
Kristjánsdóttir, G. B., Moros, M., Andrews, J. T., and Jennings, A. E.: Holocene Mg∕Ca, alkenones, and light stable isotope measurements on the outer North Iceland shelf (MD99-2269): A comparison with other multi-proxy data and sub-division of the Holocene, Holocene, 27, 52–62, 2017.
Kvisvik, B., Paasche, Ø., and Dahl, S. O.: Holocene cirque glacier activity in Rondane, southern Norway, Geomorphology, 246, 433–444, 2015.
Lasher, G. E., Axford, Y., McFarlin, J. M., Kelly, M. A., Osterberg, E. C., and Berkelhammer, M. B.: Holocene temperatures and isotopes of precipitation in Northwest Greenland recorded in lacustrine organic materials, Quaternary Sci. Rev., 170, 45–55, 2017.
Lauritzen, S.-E.: Calibration of speleothem stable isotopes against historical records: a Holocene temperature curve for North Norway?, Karst Waters Institute Special Publication, 2, 78–80, 1996.
Lauritzen, S.-E. and Lundberg, J.: Calibration of the speleothem delta function: an absolute temperature record for the Holocene in northern Norway, Holocene, 9, 659–669, 1999.
Leduc, G., Schneider, R., Kim, J. H., and Lohmann, G.: Holocene and Eemian sea surface temperature trends as revealed by alkenone and Mg∕Ca paleothermometry, Quaternary Sci. Rev., 29, 989–1004, 2010.
Lie, Ø., Dahl, S. O., Nesje, A., Matthews, J. A., and Sandvold, S.: Holocene fluctuations of a polythermal glacier in high-alpine eastern Jotunheimen, central southern Norway, Quaternary Sci. Rev., 23, 1925–1945, 2004.
Linge, H., Lauritzen, S.-E., Andersson, C., Hansen, J. K., Skoglund, R. Ø., and Sundqvist, H. S.: Stable isotope records for the last 10 000 years from Okshola cave (Fauske, northern Norway) and regional comparisons, Clim. Past, 5, 667–682, https://doi.org/10.5194/cp-5-667-2009, 2009.
Marchal, O., Cacho, I., Stocker, T. F., Grimalt, J. O., Calvo, E., Martrat, B., Shackleton, N., Vautravers, M., Cortijo, E., van Kreveld, S., and Andersson, C.: Apparent long-term cooling of the sea surface in the northeast Atlantic and Mediterranean during the Holocene, Quaternary Sci. Rev., 21, 455–483, 2002.
Moossen, H., Bendle, J., Seki, O., Quillmann, U., and Kawamura, K.: North Atlantic Holocene climate evolution recorded by high-resolution terrestrial and marine biomarker records, Quaternary Sci. Rev., 129, 111–127, 2015.
Moros, M., Andrews, J. T., Eberl, D. D., and Jansen, E.: Holocene history of drift ice in the northern North Atlantic: Evidence for different spatial and temporal modes, Paleoceanography, 21, PA2017, https://doi.org/10.1029/2005PA001214, 2006.
Moros, M., Jansen, E., Oppo, D. W., Giraudeau, J., and Kuijpers, A: Reconstruction of the late-Holocene changes in the Sub-Arctic Front position at the Reykjanes Ridge, north Atlantic, Holocene, 22, 877–886, 2012.
Nesje, A.: Latest Pleistocene and Holocene alpine glacier fluctuations Scandinavia, Quaternary Sci. Rev., 28, 2119–2136, 2009.
Orme, L. C., Miettinen, A., Divine, D., Husum, K., Pearce, C., Van Nieuwenhove, N., Born, A., Mohan, R., and Seidenkrantz, M. S: Subpolar North Atlantic sea surface temperature since 6 ka BP: Indications of anomalous ocean-atmosphere interactions at 4–2 ka BP, Quaternary Sci. Rev., 194, 128–142, 2018.
Pèlachs, A., Julià, R., Pérez-Obiol, R., Soriano, J. M., Bal, M. C., Cunill, R., and Catalan, J.: Potential influence of Bond events on mid-Holocene climate and vegetation in southern Pyrenees as assessed from Burg lake LOI and pollen records, Holocene, 21, 95–104, 2011.
Perner, K., Moros, M., Lloyd, J. M., Jansen, E., and Stein, R.: Mid to late Holocene strengthening of the East Greenland Current linked to warm subsurface Atlantic water, Quaternary Sci. Rev., 129, 296–307, 2015.
Perner, K., Jennings, A. E., Moros, M., Andrews, J. T., and Wacker, L.: Interaction between warm Atlantic-sourced waters and the East Greenland Current in northern Denmark Strait (68∘ N) during the last 10 600 cal a BP, J. Quaternary Sci., 31, 472–483, 2016.
Renssen, H., Seppä, H., Heiri, O., Roche, D. M., Goosse, H., and Fichefet, T.: The spatial and temporal complexity of the Holocene thermal maximum, Nat. Geosci., 2, 411–414, 2009.
Renssen, H., Seppä, H., Crosta, X., Goosse, H., and Roche, D. M.: Global characterization of the Holocene thermal maximum, Quaternary Sci. Rev., 48, 7–19, 2012.
Reusche, M., Winsor, K., Carlson, A. E., Marcott, S. A., Rood, D. H., Novak, A., Roof, S., Retelle, M., Werner, A., Caffee, M., and Clark, P. U: 10Be surface exposure ages on the late-Pleistocene and Holocene history of Linnébreen on Svalbard, Quaternary Sci. Rev., 89, 5–12, 2014.
Rimbu, N., Lohmann, G., Kim, J. H., Arz, H. W., and Schneider, R.: Arctic/North Atlantic Oscillation signature in Holocene sea surface temperature trends as obtained from alkenone data, Geophys. Res. Lett., 30, GL016570, https://doi.org/10.1029/2002GL016570, 2003.
Risebrobakken, B., Jansen, E., Andersson, C., Mjelde, E., and Hevrøy, K.: A high-resolution study of Holocene paleoclimatic and paleoceanographic changes in the Nordic Seas, Paleoceanography, 18, PA000764, https://doi.org/10.1029/2002PA000764, 2003.
Risebrobakken, B., Moros, M., Ivanova, E. V., Chistyakova, N., and Rosenberg, R.: Climate and oceanographic variability in the SW Barents Sea during the Holocene, Holocene, 20, 609–621, 2010.
Rohling, E. J. and Pälike, H.: Centennial-scale climate cooling with a sudden cold event around 8,200 years ago, Nature, 434, 975–979, 2005.
Roth, R. and Joos, F.: A reconstruction of radiocarbon production and total solar irradiance from the Holocene 14C and CO2 records: implications of data and model uncertainties, Clim. Past, 9, 1879–1909, https://doi.org/10.5194/cp-9-1879-2013, 2013.
Røthe, T., Bakke, J., Vasskog, K., Gjerde, M., D'Andrea, W. J., and Bradley, R. S.: Arctic Holocene glacier fluctuations reconstructed from lake sediments at Mitrahalvøya, Spitsbergen, Quaternary Sci. Rev., 109, 111–125, 2015.
Sarnthein, M., Van Kreveld, S., Erlenkeuser, H., Grootes, P. M., Kucera, M., Pflaumann, U., and Schulz, M.: Centennial-to-millennial-scale periodicities of Holocene climate and sediment injections off the western Barents shelf, 75∘ N, Boreas, 32, 447–461, 2003.
Schmidt, S., Wagner, B., Heiri, O., Klug, M., Bennike, O., and Melles, M.: Chironomids as indicators of the Holocene climatic and environmental history of two lakes in Northeast Greenland, Boreas, 40, 116–130, 2011.
Seierstad, J., Nesje, A., Dahl, S. O., and Simonsen, J. R.: Holocene glacier fluctuations of Grovabreen and Holocene snow-avalanche activity reconstructed from lake sediments in Grningstlsvatnet, western Norway, Holocene, 12, 211–222, 2002.
Sejrup, H. P., Haflidason, H., and Andrews, J. T.: A Holocene North Atlantic SST record and regional climate variability, Quaternary Sci. Rev., 30, 3181–3195, 2011.
Sejrup, H. P., Seppä, H., McKay, N. P., Kaufman, D. S., Geirsdóttir, Á., de Vernal, A., Renssen, H., Husum, K., Jennings, A., and Andrews, J. T.: North Atlantic-Fennoscandian Holocene climate trends and mechanisms, Quaternary Sci. Rev., 147, 365–378, 2016.
Shakesby, R. A., Smith, J. G., Matthews, J. A., Winkler, S., Quentin Dresser, P., Bakke, J., Dahl, S. O., Lie, Ø., and Nesje, A.: Reconstruction of Holocene glacier history from distal sources: glaciofluvial stream-bank mires and a glaciolacustrine sediment core near Sota Sæter, Breheimen, southern Norway, Holocene, 17, 729–745, 2007.
Sicre, M. A., Jacob, J., Ezat, U., Rousse, S., Kissel, C., Yiou, P., Eiríksson, J., Knudsen, K. L., Jansen, E., and Turon, J. L.: Decadal variability of sea surface temperatures off North Iceland over the last 2000 years, Earth Planet. Sc. Lett., 268, 137–142, 2008.
Snowball, I. and Sandgren, P.: Lake sediment studies of Holocene glacial activity in the Kårsa valley, northern Sweden: contrast in interpretation, Holocene, 6, 367–372, 1996.
Solignac, S., Giraudeau, J., and de Vernal, A.: Holocene sea surface conditions in the western North Atlantic: spatial and temporal heterogeneities, Paleoceanography, 21, PA001175, https://doi.org/10.1029/2005PA001175, 2006.
Solomina, O. N., Bradley, R. S., Hodgson, D. A., Ivy-Ochs, S., Jomelli, V., Mackintosh, A. N., Nesje, A., Owen, L. A., Wanner, H., Wiles, G. C., and Young, N. E.: Holocene glacier fluctuations, Quaternary Sci. Rev., 111, 9–34, 2015.
Støren, E. N., Dahl, S. O., and Lie, Ø.: Separation of late-Holocene episodic paraglacial events and glacier fluctuations in eastern Jotunheimen, central southern Norway, Holocene, 18, 1179–1191, 2008.
Svendsen, J. I. and Mangerud, J.: Holocene glacial and climatic variations on Spitsbergen, Svalbard, Holocene, 7, 45–57, 1997.
Telesiński, M. M., Spielhagen, R. F., and Lind, E. M.: A high-resolution Late glacial and Holocene palaeoceanographic record from the Greenland Sea, Boreas, 43, 273–285, 2014.
Van der Bilt, W. G. M, Bakke, J., Vasskog, K., D'Andrea, W. J., Bradley, R. S., and Ólafsdóttir, S.: Reconstruction of glacier variability from lake sediments reveals dynamic Holocene climate in Svalbard, Quaternary Sci. Rev., 126, 201–218, 2015.
Van der Bilt, W. G. M., Rea, B., Spagnolo, M., Roerdink, D. I., Jorgensen, S. I., and Bakke, J.: Novel sedimentological fingerprints link shifting depositional processes to Holocene climate transitions in East Greenland, Global Planet. Change, 164, 52–64, 2018a.
Van der Bilt, W. G. M., D'Andrea, W. J., Bakke, J., Balascio, N. L., Werner, J. P., Gjerde, M., and Bradley, R. S.: Alkenone-based reconstructions reveal four-phase Holocene temperature evolution for High Arctic Svalbard, Quaternary Sci. Rev., 183, 204–213, 2018b.
Van Nieuwenhove, N., Knudsen, M. F., Pearce, C., Røy, H., and Seidenkrantz, M.-S.: Meltwater and seasonality influence on subpolar Gyre circulation during the Holocene, Palaeogeogr. Palaeocl., 502, 104–118, 2018.
Vasskog, K., Nesje, A., Støren, E. N., Waldmann, N., Chapron, E., and Ariztegui, D.: A Holocene record of snow-avalanche and flood activity reconstructed from a lacustrine sedimentary sequence in Oldevatnet, western Norway, Holocene, 21, 597–614, 2011.
Vasskog, K., Paasche, Ø., Nesje, A., Boyle, J. F., and Birks, H. J. B.: A new approach for reconstructing glacier variability based on lake sediments recording input from more than one glacier, Quaternary Res., 77, 192–204, 2012.
Velle, G., Larsen, J., Eide, W., Peglar, S. M., and Birks, H. J. B.: Holocene environmental history and climate of Råtåsjøen, a low alpine lake in south-central Norway, J. Paleolimnol., 33, 129–153, 2005.
Vieira, L. E. A., Solanki, S. K., Krivova, N. A., and Usoskin, I.: Evolution of the solar irradiance during the Holocene, Astron. Astrophys., 531, A6, https://doi.org/10.1051/0004-6361/201015843, 2011.
Vinther, B., Buchardt, S. L., Clausen, H. B., Dahl-Jensen, D., Johnsen, S. J., Fisher, D. A., Koerner, R. M., Raynaud, D., Lipenkov, V., Andersen, K. K., Blunier, T., Rasmussen, S. O., Steffensen, J. P., and Svensson, A. M.: Significant Holocene thinning of the Greenland ice sheet, Nature, 461, 385–388, 2009.
Wagner, B. and Bennike, O.: Holocene environmental change in the Skallingen area, eastern North Greenland, based on a lacustrine record, Boreas, 44, 45–59, 2015.
Wang, Y., Cheng, H., Edwards, R. L., He, Y., Kong, X., An, Z., Wu, J., Kelly, M. J., Dykoski, C. A., and Li, X.: Holocene Asian monsoon: links to solar changes and North Atlantic climate, Science, 308, 854–857, 2005.
Wanner, H. and Bütikofer, J.: Holocene Bond Cycles: real or imaginary?, Geografie, 113, 338–349, 2008.
Wanner, H., Beer, J., Buetikofer, J., Crowley, T. J., Cubasch, U., Flueckiger, J., Goosse, H., Grosjean, M., Joos, F., and Kaplan, J. O.: Mid-to late Holocene climate change: an overview, Quaternary Sci. Rev., 27, 1791–1828, 2008.
Wanner, H., Solomina, O., Grosjean, M., Ritz, S. P., and Jetel, M.: Structure and origin of Holocene cold events, Quaternary Sci. Rev., 30, 3109–3123, 2011.
Weiss, H.: 4.2 ka BP megadrought and the Akkadian collapse, in: Megadrought and Collapse: from early agriculture to Angkor, edited by: Weiss, H., Oxford University Press, Oxford, 93–160, 2017.
Werner, K., Frank, M., Teschner, C., Müller, J., and Spielhagen, R. F.: Neoglacial change in deep water exchange and increase of sea-ice transport through eastern Fram Strait: evidence from radiogenic isotopes, Quaternary Sci. Rev., 92, 190–207, 2014.
Wittmeier, H. E., Bakke, J., Vasskog, K., and Trachsel, M.: Reconstructing Holocene glacier activity at Langfjordjøkelen, Arctic Norway, using multi-proxy fingerprinting of distal glacier-fed lake sediments, Quaternary Sci. Rev., 114, 78–99, 2015.
Wu, C. J., Usoskin, I. G., Krivova, N., Kovaltsov, G. A., Baroni, M., Bard, E., and Solanki, S. K.: Solar activity over nine millennia: A consistent multi-proxy reconstruction, Astron. Astrophys., 615, A93, https://doi.org/10.1051/0004-6361/201731892, 2018.
Zhang, Y., Renssen, H., and Seppä, H.: Effects of melting ice sheets and orbital forcing on the early Holocene warming in the extratropical Northern Hemisphere, Clim. Past, 12, 1119–1135, https://doi.org/10.5194/cp-12-1119-2016, 2016.